Abstract
The fungal pathogen Drechmeria coniospora infects C. elegans and elicits an innate immune response mediated, in part, by the induction of antimicrobial peptides in the epidermis. The signaling pathways controlling this phenomenon remain to be fully characterized. In this issue of Virulence, Couillault and colleagues use both proteomics and genetics to discover an unexpected role for the unfolded protein response (UPR) target chaperone BiP/GRP78/hsp-3 in the control of fungal infection-induced antimicrobial peptide expression in C. elegans. Although the expression of hsp-3 is regulated by the UPR, Couillault and colleagues describe a novel signaling role for this BiP/GRP78 homolog.
Keywords: :
The nematode C. elegans has emerged as a powerful in vivo platform for studies of the innate immune response. C. elegans can be infected with a wide variety of bacterial and fungal pathogens that interact with both intestinal and epidermal epithelial cells. The natural fungal pathogen Drechmeria coniospora infects C. elegans by first adhering to the cuticle and then extending invasive hyphae that penetrate the cuticle and grow into the underlying hypodermal epithelial cells. This infection process leads to activation of the worm’s innate immune response, which involves the transcriptional upregulation of many genes, including several proposed antimicrobial peptides (AMPs), including the neuropeptide-like protein (nlp) and caenicin (cnc) family of genes.Citation1 The expression of many nlp and cnc genes is regulated by a well-conserved p38 MAP kinase signaling pathway,Citation2 although the most distal parts of this pathway that detect the pathogen and activate p38 MAP kinase signaling are not well defined.
Previous studies have characterized the transcriptional response of C. elegans to D. coniospora infection.Citation3 To gain additional insights, Couillault et al.Citation4 used a comparative proteomics approach to identify host proteins whose abundance in specific subcellular fractions was altered upon infection. Among the proteins identified, HSP-3 representation was increased upon infection whereas representation of its homolog HSP-4 was not. hsp-3 and hsp-4 encode the worm of orthologs of mammalian BiP/GRP78, which, unlike C. elegans, is encoded by a single gene.Citation5 BiP encodes an ER resident protein chaperone with two roles in the UPR. First, BiP regulates UPR signaling via interactions with the ER stress sensors ATF6, PERK and IRE1. Second, BiP is a transcriptional target of the UPR and therefore acts in a feedback loop to regulate UPR activity and restore ER homeostasis.Citation6 Although C. elegans hsp-3 and hsp-4 also share these roles,Citation7 previous studies have been unable to functionally differentiate these two genes. Using genetics approaches, Couillault et al. provide the first data demonstrating functional differences in hsp-3 and hsp-4. They find that hsp-3, but not hsp-4, is required to activate AMP expression following D. coniospora infection. Interestingly, hsp-3 was not required for AMP expression following induction by other stressors, including physical wounding or hyperosmotic stress. Therefore, hsp-3 has a specific functional role in the regulation of infection-induced pathways controlling AMP expression. The mechanism(s) by which hsp-3 contributes to innate immune signaling remains unresolved. Whether such a mechanism involves exotic cytoplasmic functions of this typically ER localized chaperone or more typical chaperone-client protein regulatory interactions will require additional studies to determine.
Most previous studies in C. elegans have investigated the role of the UPR in response to stressors delivered to the intestine, such as pore-forming toxins or intestine colonizing bacteria.Citation8,Citation9 The findings by Couillault represent one of the first non-intestinal stress-responsive roles described for the UPR in C. elegans. One interesting observation made by the authors is that treatment of larval, but not adult, worms with the UPR inducer tunicamycin (as well as other ER stressors like DTT) activated expression of the AMP reporter transgene nlp-29::GFP in the hypodermis. Why might generic ER stress act in such a developmentally and tissue-restricted manner to activate AMP levels? One possibility is that D. coniospora places a proteotoxic burden on the hypodermal secretory system, perhaps via massive biosynthesis of AMPs or as a compensatory attempt to repair/replace widespread damage to the hypodermal membrane or extracellular matrix. However, this load only exceeds ER folding capacity in larval animals because it occurs contemporaneously with the synthesis of the new cuticle, when many secretory proteins (i.e., collagens) are being produced. Given this massive secretory load in larval animals, UPR inducers, such as tunicamycin, may therefore exceed the folding capacity of the secretory pathway in larval animals, but not in adults, which have a lower secretory burden. In this respect, the UPR may be an important “sensor” of infection in larvae but not in adults. Further work is needed to unravel which UPR pathways may be important for activation of AMP gene expression during this potentially vulnerable developmental window.
The findings of Couillault and colleagues raise several interesting questions that need to be further addressed in order to understand the role of hsp-3 in the antimicrobial signaling pathway. Indeed, the genetic interactions presented in the manuscript position hsp-3 downstream of nipi-3 and upstream of tpa-1. However HSP-3, an ER luminal protein, is not likely to interact with those proteins in the cytosol (see ), a possibility noted by Couillault et al. A more likely possibility is that HSP-3 regulates the folding, maturation, or processing of one or more client proteins whose activity is required for the Nipi signaling pathway (). A prediction of this model is that inhibition of such a HSP-3 client protein(s) should produce a Nipi phenotype. Perhaps upon D. coniospora infection, the innate immune response elicits major demands on the secretory pathway to bring specific proteins to the cell surface as an adaptive response, and increased HSP-3 abundance/activity is required to meet such demands. Interestingly, hsp-3 has not been found to be upregulated in previous transcriptional studies of D. coniospora infection,Citation3 suggesting that this mechanism may involve regulation of HSP-3 function via post-transcriptional mechanisms, such as altered protein stability, subcellular localization or post-translational modification(s). Further studies will be required to distinguish among these possibilities.
Figure 1. HSP-3 contains a C-terminal KDEL motif and an N-terminal signal sequence and is therefore predicted to be a resident ER protein. We diagram an alternative model to that suggested by Couillault et al. Given the chaperone activity and predicted ER localization of HSP-3, we suggest that HSP-3 does not play a direct role in signaling with NIPI-3 and TPA-1, which are likely cytoplasmic signaling proteins. Rather, HSP-3 may be required for the processing of an additional signaling molecule within the ER that matures through the secretory pathway and acts as an intermediate in signaling between NIPI-3 and TPA-1. Given that hsp-3 mutants have a Nipi phenotype, a prediction of this model is that mutations in the unidentified substrate may also produce a Nipi phenotype. We suggest that this unidentified molecule is a transmembrane protein, although it is also possible that it could encode a secreted membrane anchored protein. Loss of function mutations in this hypothetical hsp-3 client would act genetically upstream of tpa-1 but downstream of nipi-3, just as the authors described for hsp-3.
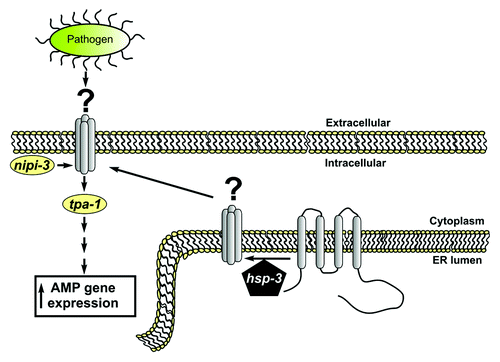
In conclusion, Couillault et al. have identified an unexpected and specific role for hsp-3 in the regulation of the innate immune signaling pathway. Such roles might only be differentiated in C. elegans, where the functional redundancy between BiP homologs permits such a separation of function. Going forward, it will be important to determine if BiP plays similar functional roles in the vertebrate innate immune response to pathogen infection.
References
- Shivers RP, Youngman MJ, Kim DH. Transcriptional responses to pathogens in Caenorhabditis elegans. Curr Opin Microbiol 2008; 11:251 - 6; http://dx.doi.org/10.1016/j.mib.2008.05.014; PMID: 18567532
- Ziegler K, Kurz CL, Cypowyj S, Couillault C, Pophillat M, Pujol N, et al. Antifungal innate immunity in C. elegans: PKCdelta links G protein signaling and a conserved p38 MAPK cascade. Cell Host Microbe 2009; 5:341 - 52; http://dx.doi.org/10.1016/j.chom.2009.03.006; PMID: 19380113
- Pujol N, Zugasti O, Wong D, Couillault C, Kurz CL, Schulenburg H, et al. Anti-fungal innate immunity in C. elegans is enhanced by evolutionary diversification of antimicrobial peptides. PLoS Pathog 2008; 4:e1000105; http://dx.doi.org/10.1371/journal.ppat.1000105; PMID: 18636113
- Couillault C, Fourquet P, Pophillat M, Ewbank J. A UPR-independent infection-specific role for a BiP/GRP78 protein in the control of antimicrobial peptide expression in C. elegans epidermis. Virulence 2012; 3:299 - 308; http://dx.doi.org/10.4161/viru.20384; PMID: 22546897
- Heschl MF, Baillie DL. Functional elements and domains inferred from sequence comparisons of a heat shock gene in two nematodes. J Mol Evol 1990; 31:3 - 9; http://dx.doi.org/10.1007/BF02101786; PMID: 2116528
- Schröder M, Kaufman RJ. The mammalian unfolded protein response. Annu Rev Biochem 2005; 74:739 - 89; http://dx.doi.org/10.1146/annurev.biochem.73.011303.074134; PMID: 15952902
- Kapulkin WJ, Hiester BG, Link CD. Compensatory regulation among ER chaperones in C. elegans. FEBS Lett 2005; 579:3063 - 8; http://dx.doi.org/10.1016/j.febslet.2005.04.062; PMID: 15907843
- Bischof LJ, Kao CY, Los FC, Gonzalez MR, Shen Z, Briggs SP, et al. Activation of the unfolded protein response is required for defenses against bacterial pore-forming toxin in vivo. PLoS Pathog 2008; 4:e1000176; http://dx.doi.org/10.1371/journal.ppat.1000176; PMID: 18846208
- Richardson CE, Kooistra T, Kim DH. An essential role for XBP-1 in host protection against immune activation in C. elegans. Nature 2010; 463:1092 - 5; http://dx.doi.org/10.1038/nature08762; PMID: 20182512