Abstract
Human cytomegalovirus (HCMV), a member of the herpesvirus family, establishes life-long persistence and latency after primary infection and can be reactivated later in life. In immunosuppressed patients, it is an important pathogen that can cause severe disease. HCMV is also thought to play a causative role in inflammatory diseases and cancer. The virus can infect different immune cells, including dendritic cells (DCs) and can take advantage of host immune functions to avoid immune recognition. These characteristics have sparked major interest in understanding HCMV and its interaction with immune cells and their relevance to disease pathogenesis. In this review, we focus on the complex host-pathogen relationship between HCMV and DCs, including the persistence of the virus in these cells, their function in the immune response to HCMV infection and the potential clinical consequences of HCMV infection in DCs.
Introduction
Human cytomegalovirus (HCMV) belongs to the herpesvirus family, and 60–100% of individuals in populations worldwide are persistently infected with and carry the virus. Usually acquired early in life, the infection can be transferred through all bodily fluids. Interest in HCMV as an important human pathogen has risen over the past decades, owing to increases in the numbers of AIDS patients and patients undergoing immunosuppressive therapy after organ or bone marrow transplantation.Citation1 HCMV infection is usually subclinical in immunocompetent individuals, but the virus can cause fatal disease in immunocompromised patients. HCMV infections occur in about 50–90% of patients after bone marrow and organ transplantation. In HIV-infected patients, however, the prevalence of HCMV approaches 100%.Citation1 Furthermore, HCMV infection is the most common congenital viral infection (~1% of live births); it may cause viral hepatitis with jaundice as well as permanent disabilities, including hearing loss, visual impairment, mental retardation and other neurological impairments.
In immunocompetent hosts, the infection rarely causes symptoms. However, mononucleosis caused by HCMV infection is sometimes observed (reviewed in ref. Citation2). For many years, HCMV was not considered to be a major human pathogen, but active HCMV has been found in tissue specimens from immunocompetent patients with inflammatory diseases, including inflammatory bowel disease (IBD), systemic lupus erythematosus (SLE), psoriasis and atherosclerosis; it has also been implicated in the development of these diseases and in restenosis after coronary angioplasty, chronic rejection in organ transplant patients, chronic graft-vs.-host disease in bone marrow transplant patients and tumors (reviewed in ref. Citation3).
After primary HCMV infection, the virus persists in a latent state and can be reactivated later in life. Latency is characterized by persistence of the viral genome without production of infectious virus—a strategy that enables the virus to co-exist with its host by reducing its visibility, leading to an avoidance of immune recognition. HCMV infects many different immune cells, including monocytes, macrophages, dendritic cells (DCs) and granulocytes, and takes advantage of host immune functions to escape immune recognition to avoid viral clearance.
In this review, we focus on the complex host-pathogen relationship between HCMV and DCs, including the persistence of the virus in these cells, their function in both the innate and adaptive immune response to HCMV infection and the potential clinical consequences of HCMV infection in DCs.
HCMV Immune Evasion Strategies
A functional immune system is of utmost importance to efficiently combat viral infections. HCMV has developed multiple immune evasion strategies to establish latency and co-exist with its host. Increasing evidence suggests that HCMV infection adversely affects both innate and adaptive immune responses. For example, HCMV escapes immune recognition by CD8+ and CD4+ T cells by downregulating expression of major histocompatibility complex (MHC) class I and II on infected cells. HCMV interferes with MHC class II expression in several ways, including US2- and pp65-dependent degradation of MHC class II proteins;Citation4,Citation5 binding of US3 to class II α/β complexes and preventing their association with the invariant chain;Citation6 inhibiting CD10 and CD13 metalloproteinases, which participate in peptide trimming in both the MHC class I and II antigen presentation pathways and interfering with the CIITA (class II activator)/JAK-STAT signaling pathway, which tightly controls the promoter of the MHC class II region (reviewed in ref. Citation7).
Cytotoxic T cells (CD8 T cells) are crucial for clearing viral infections, and decreased expression of MHC class I molecules on virus-infected cells potentially facilitates the establishment of a persistent or latent infection. The antigen-presentation route of MHC class I peptide complexes can be blocked by several HCMV genes, including US2, US3, US6, US10 and US11 (reviewed in ref. Citation8) as well as by the tegument proteins UL82 (pp71) and UL83 (pp65),Citation8 and by specific downregulation of ERAP1, an aminopeptidases that reside in the endoplasmic reticulum, by the HCMV microRNA miR-US4-1.Citation9 Downregulation of MHC class I molecules and avoidance of CD8+ T-cell responses appear to be critical in allowing superinfection of persistently infected hosts,Citation10 which generally occurs in immunocompetent hosts who may carry multiple strains of HCMV.
The downregulation of MHC-class I antigens on the cell surface would render the cells susceptible to lysis by natural killer (NK) cells, which respond to the absence of MHC class I molecules. However, HCMV-infected cells are resistant to NK lysis. Potential mechanisms for this resistance have been extensively reviewed.Citation11 In short, two HCMV proteins act on the NK cell inhibitory receptors to prevent lysis mediated by NK cells. The first is UL18,Citation12 an MHC class I homolog that mediates resistance to NK cells. However, cells infected with a UL18 knockout strain of HCMV are less sensitive to NK lysis, implying additional pathways for the lytic effect.Citation13 The second is a UL40-derived peptide that promotes the cell-surface expression of HLA-E, which suppresses lysis by NK cells by interacting with the NK cell inhibitory receptor complex CD94/NKG2A.Citation11 HCMV also encodes several genes that act on NK cell activating receptors: UL16, UL83, UL141, UL142 and microRNA-UL112.Citation11
DCs and macrophages are specialized antigen-presenting cells (APCs) that are important mediators of immunity. Professional APCs capture, digest and present antigens to lymphocytes, produce cytokines and respond to stimuli from responding lymphocytes. APCs constitutively express MHC class II proteins and normally present antigens as MHC class II-bound peptides to resting CD4+ T cells. Since DCs play a major role in both the innate and adaptive immune responses and affect most immune cells, interference with their functions by HCMV is a powerful potential mechanism for the virus to inhibit the immune response and thereby avoid immune recognition.
Development and Function of Different Human DC Subsets
DCs play a central role in activating T and B cells and thereby direct the immune response against invading microorganisms. DCs vary in their function, location and cell-surface markers, and all but Langerhans cells (LCs) are believed to be derived from hematopoietic stem cells (CD34) residing in the bone marrow.Citation14,Citation15 Different subsets of DCs are found in distinct tissue locations throughout the body, where they mediate key functions of the innate and adaptive immune responses. Immature DCs reside in peripheral tissues, such as the lungs, skin and gastrointestinal tract. Upon exposure to pathogens, immature DCs becomes activated, mature, migrate to lymph nodes and present their captured antigens to naïve T cells. Their activation is influenced by pathogen-associated molecular patterns and their vertebrate receptors, including toll-like receptors (TLRs). MHC class I and II molecules present the captured antigen to immature DCs, which mature and are transported to draining lymph nodes to prime naïve T cells. Two major subtypes of DCs have identified in human blood: CD11c+ myeloid DCs (mDCs) and CD11c–CD123+ plasmacytoid DCs (pDCs).
Myeloid DCs
The complex heterogeneity of DCs makes it difficult to define their origin or their divergence from monocytes and macrophages; this concept has been extensively reviewed elsewhere.Citation14,Citation15 In brief, monocytes are considered to be progenitors of both DCs and macrophages and can differentiate into either cell type in vitro, depending on the cytokine stimuli they are exposed to. Upon stimulation with granulocyte-monocyte colony-stimulating factor (GM-CSF) and interleukin (IL) 4,Citation16 monocytes differentiate into immature DCs; these monocyte-derived DCs (moDCs) express high levels of CD11c and MHC class II.Citation15 Upon stimulation with cytokines, such as mononuclear phagocyte colony-stimulating factor (M-CSF) and IFN-γ/tumor necrosis factor (TNF)-α or allogeneic cytokines, monocytes differentiate into macrophages of the mononuclear phagocyte system.Citation17,Citation18 Stimulation with GM-CSF results in type 1 macrophages, which are proinflammatory, while stimulation with M-CSF results in type 2 macrophages, which have anti-inflammatory functions.Citation19
LCs reside in the skin and migrate to the lymph nodes to present antigens, and have many features of DCs. LCs are believed to be self-renewing in situ from a local population of myelomonocytic precursor cells in the skin. These cells appear to resemble the bone marrow precursors of myeloid cells that are present in the early fetal development and colonize the skin and proliferate around birth.Citation14,Citation15
Plasmacytoid DCs
Plasmacytoid DCs (pDCs) originate in the bone marrow from myeloid and lymphoid precursorsCitation20 and are important mediators of antiviral immunity. They are primarily involved in the innate immune response as they secrete large amounts of type I IFNs upon viral infection, which is largely mediated through the TLR pathwayCitation20 and results in the activation of T cells, NK cells and B cells. pDCs develop fully in the bone marrow, are released into the bloodstream and are found in small numbers mainly in secondary lymphoid organs and the thymus.Citation21 Upon viral infection, pDCs sense viral nucleic acids within the early endosomes through TLR7 and TLR9.Citation22 TLR9 senses double-stranded DNA of bacteria and viruses rich in unmethylated CpG sequences. TLR7 detects single-stranded viral RNA. TLR9 is required for pDCs to respond to DNA viruses, such as herpesviruses (HSV-1, HSV-2, murine CMV and HCMV).Citation23-Citation26 Type I IFNs produced by pDCs inhibit viral infection by augmenting the antiviral state through induction and activation of numerous antiviral molecules and functions in other types of lymphoid and myeloid cells (NK cells, mDCs and B and T cells) involved in innate and adaptive immunity.
HCMV Resides In and Can Reactivate from Latency in Cells of the Myeloid Lineage
An important biological property of HCMV is its ability to persist in a latent form and be reactivated under certain conditions. One of the key cell types in HCMV infection and latency are cells of the myeloid lineage, which develop from hematopoietic stem cells. HCMV can infect monocytes, macrophages, DCs, granulocytes, fibroblasts and endothelial, epithelial, neuronal and smooth muscle cells. Neutrophils are considered to be the major cell type carrying the virus during acute infection,Citation27 but they cannot support viral replication. Monocytes/macrophages are thought to be responsible for dissemination of the virus and are the primary reservoir of latent HCMV in the peripheral blood in seropositive individuals.Citation28
Current evidence suggests that HCMV cannot efficiently replicate in monocytes. In these cells, HCMV infection is nonpermissive, as viral gene expression and transcription are either absent or restricted to immediate-early gene products.Citation29-Citation32 The absence of late gene expression and viral production is consistent with the hypothesis that monocytes or premonocytic cells are reservoirs for latent virus. In contrast, tissue macrophages in HCMV-infected patients can express late viral genesCitation33 and produce infectious virus. Differentiation of monocytes into macrophages is a prerequisite for productive HCMV infection.Citation18,Citation29,Citation34
HCMV infection behaves differently in macrophages generated by different stimuli. For example, HCMV infects relatively few macrophages generated by cocultivation of monocytes with concanavalin A stimulated autologous nonadherent cells, but infects the majority of macrophages generated by allogeneic stimulation of peripheral blood mononuclear cells.Citation35 The state of cellular differentiation is therefore a major factor in the ability of the virus to replicate, which is also the case for reactivation of latent HCMV. Since peripheral blood monocytes have a short half-life, reactivation of HCMV in macrophages suggests that HCMV is maintained in a precursor population of the myeloid cell lineage. Such cells would provide an ideal latency site for a virus whose activation is closely linked to the immune system. HCMV can infect CD34+ pluripotent stem cells both in vitro and in vivo.Citation36-Citation38 HCMV DNA follows the myeloid differentiation pathway.Citation39 Latent HCMV can be reactivated in myeloid DCs from HCMV-seropositive donors after ex vivo differentiation to mature DCsCitation40 or in macrophages produced by allogeneic stimulation of peripheral blood mononuclear cells (PBMCs).Citation41 The inflammatory cytokines IFN-γ and TNF-α produced by allo-stimulated T cells are important for the reactivation of latent virus and the growth of HCMV in differentiated macrophages.Citation42 IL-6 and subsequent signaling are important for reactivation from both experimental and natural latency in differentiating DCs.Citation43,Citation44 Clinically, immune activation and concomitant production of pro-inflammatory cytokines appear to be essential for reactivation and replication of HCMV in infected patients.Citation45-Citation47 Thus, immune activation, production of inflammatory cytokines and myeloid cell differentiation are likely to be important for viral reactivation and replication in HCMV-infected patients.
HCMV Inhibits Differentiation of Myeloid Cells
The maintenance of latency and reactivation is closely linked to the stage of cellular differentiation, and differentiation of all infected cells after viral entry would efficiently eliminate the virus during the infectious cycle. We showed that, after stimulation with IL-4 and GM-CSF, HCMV-infected monocytes fail to differentiate into moDCs and have a decreased ability to further mature in response to stimulation with lipopolysaccharide ().Citation48 The infected cells also had severe impairments in immunological functions, including endocytosis, phagocytosis, migration in response to inflammatory chemokines and induction of T-cell responses in vitro. In a recent study, monocytes were infected with an endothelial cell-adapted HCMV strain, and differentiated into moDCs; the resulting CMV-moDCs did not acquire the standard CD1a+ immature phenotype of moDCs, but instead acquired the maturation marker CD83.Citation49 CMV-moDCs had decreased STAT5 phosphorylation, indicating defective GM-CSF signaling that was mediated by soluble factors and involved the secretion of IL-6 by infected cells. Consistent with our findings, CMV-moDCs had a decreased ability for phagocytosis and stimulating T-cell proliferation and failed to induce a TH1 response.Citation49
Figure 1. HCMV-infected monocytes fail to differentiate into MoDCs upon cytokine stimulation. HCMV infection of moDCs further impairs the function of these cells, whose maturation is impaired both by HCMV infection and the virally encoded protein cmvIL-10. HCMV infection of moDCs impairs the ability of the DCs to present antigens to T cells and subsequent T-cell proliferation, through mechanisms such as downregulating class II and CD1 expression, inducing apoptosis of T cells via CD95L and TRAIL, and altering cytokine production as well as mechanisms involving release of soluble CD83 and virally encoded UL18 and cmvIL-10. HCMV infection of moDCs also impairs the ability of the DCs to migrate in response to chemokines by causing the cell to internalize CCR1 and CCR5, by inhibiting the switch from CCR5 to CCR7, and by mechanisms involving virally encoded UL18.
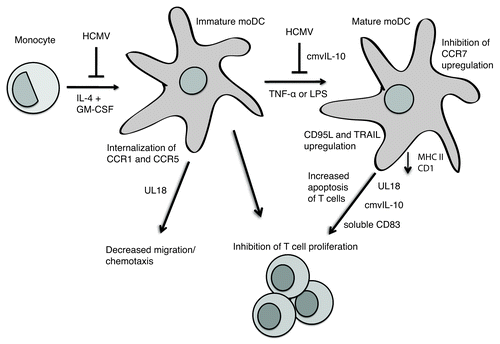
Besides affecting DC differentiation, HCMV reversibly blocks cytokine-induced macrophage differentiation, leading to impairments in phagocytosis and migration of these cells and possibly to a depressed immune response during an acute HCMV infection.Citation50 The cell-surface molecule CD13 (a putative HCMV receptor)Citation51 and HCMV glycoprotein B seem to be involved in the inhibition of macrophage differentiation.Citation52
The inhibitory effect of HCMV on monocyte differentiation, either directly or by viral proteins such as glycoprotein B or monocyte-produced cytokines, and the central role of monocytes, DCs and macrophages in innate and adaptive immunity are consistent with the increased sensitivity of HCMV-infected patients to other infections.Citation53-Citation55
Monocyte-Derived DCs and HCMV Infection
Interest in HCMV-infected DCs, especially moDCs, has grown with improvements in experimental conditions for infecting myeloid cells with HCMV in vitro. MoDCs are produced by stimulating peripheral blood (CD14+) monocytes with IL-4 and GM-CSF,Citation16 resulting in the generation of immature moDCs that mature in response to treatment with TNF-α or lipopolysaccharide. Although HCMV can productively infect moDCs in vitro,Citation56 laboratory-adapted HCMV strains do not exhibit a full replication cycle in DCs, which is dependent on mutations in the UL128-UL131A gene region, leading to loss of dendritic and endothelial cell tropism.Citation57-Citation59 Instead, clinical isolates grown in endothelial cells that maintain the UL128–131A locus can be used to infect DCs.Citation60 HCMV strains that productively infect cells of myeloid origin (monocytes/macrophages and DCs) in vitro include TB40/E, VHLE, VR1814 and FIX.Citation44,Citation56,Citation59 HCMV infection of moDCs is facilitated by the binding of HCMV glycoprotein B to the DC membrane protein DC-SIGN, which increases the susceptibility of moDCs to HCMV.Citation61 Soluble DC-SIGN (sDC-SIGN), which is increased in body fluids in inflammatory settings and upon HCMV infection in human tissue explants, enhances HCMV infection in moDCs, potentially by serving as an opsonin for HCMV virions.Citation62
HCMV enters DCs via a macropinocytosis-like pathway.Citation63 An early study showed that HCMV infection in moDCs results in maturation of the surviving cells, upregulation of the costimulatory molecules CD40, CD80 and CD86, and downregulation of MHC class I and class II molecules.Citation64 HCMV-infected mature moDCs suppressed T-cell proliferation and induced apoptosis in T cells through CD95L and TRAIL ().Citation64 However, subsequent studies yielded somewhat different findings, including an HCMV-induced block of DC maturation, decreased expression of co-stimulatory molecules, and unaffected or slightly reduced levels of CD86 and MHC class II expression.Citation65,Citation66 HCMV infection of DCs also decreased production of the cytokines TNF-α and IL-12 and impaired the proliferation and cytotoxity of T cells.Citation66
DCs are essential for generating an antiviral response, in part by inducing cytokine and chemokine responses. For example, type I IFNs produced by immune cells limit viral spread and activate cytotoxic T-cell and B-cell responses against infected cells. HCMV infection in moDCs upregulates the expression of genes encoding proinflammatory cytokines and chemokines, TLR3 expression and expression of genes whose products function downstream of the TLR3 signaling pathway (e.g., IFN-α and IFN-β).Citation67 However, the early HCMV-triggered immune response in human moDCs appears to be independent of TLR3, as silencing of TLR3 expression before HCMV infection of the cells does not influence IFN-β expression.Citation67
One of the major effects of HCMV infection in moDCs in vitro is inhibition of T-cell proliferation, possibly because the virus inhibits the maturation of moDCs.Citation65,Citation66 HCMV infection of mature moDCs results in release of the soluble form of CD83 that specifically inhibits T-cell proliferation ().Citation68 Furthermore, the HCMV-encoded MHC class I homolog UL18 impairs induction of T-cell proliferation after CD40L-induced DC maturation ().Citation69 Although the binding of UL18 to immature moDCs increased secretion of IL-10 and IL-6, the decreased T-cell proliferation was not explained by IL-10. UL18 also induced the expression of the maturation marker CD83 on moDCs.
To fulfill their function and activate the immune response, DCs must migrate from the site of infection to the lymph nodes, the site of T-cell priming, in response to CCL19 and CCL21, chemokines produced by secondary lymphoid organs. A switch from CCR5 to CCR7 during DC maturation enables the cells respond to these chemokines. However, HCMV can prevent this chemokine receptor switch in infected moDCs—and thereby inhibit migration of mature moDCs in response to CCL19 and CCL21.Citation70 In immature moDCs, HCMV infection impairs chemotaxis in response to CCL3 and CCL5, and internalizes CCR1 and CCR5, but does not affect CCR7.Citation71 The HCMV protein UL18 also reduces the chemotaxis of moDCs in response to RANTES.Citation69 Evidently, HCMV uses multiple strategies to alter DC trafficking, which is important for an adequate immune response upon infection ().
During latency, HCMV appears to produce a latency-associated form of the HCMV-encoded IL-10 homolog cmvIL-10Citation72 through altered splicing.Citation73 Treatment of myeloid cell populations with recombinant latency-associated cmvIL-10 (LAcmvIL-10) downregulates MHC class II expression in granulocyte-macrophage progenitor cells and monocytes but does not affect the maturation of moDCs or their production of proinflammatory cytokines.Citation74 cmvIL-10 inhibits differentiation of DCs from latently infected CD34+ progenitor cells, possibly by suppressing the transcription of proinflammatory cytokines.Citation75 cmvIL-10 can also inhibit the maturation of moDCs and production of the proinflammatory cytokines IL-6, IL-12 and TNF-α;Citation76 however, cmvIL-10-treated moDCs that did mature migrated to a greater extent than mock-treated moDCs.Citation76 Furthermore, cmvIL-10 impairs the functional maturation of immature moDCs, suppressing their production of IL-10 and IL-12, increasing apoptosis of the cells.Citation77 By upregulating DC-SIGN, cmvIL-10 increases the susceptibility of DCs to HCMV infection.Citation77 In moDCs, cmvIL-10 interferes with CD1 antigen presentation by decreasing transcription of CD1 through an unknown mechanism.Citation78
HCMV Infection in Langerhans DCs
HCMV infection of Langerhans DCs (LCs) has been studied in an in vitro system in which LCs are derived from CD34+ progenitor cells.Citation79 The susceptibility of LCs to HCMV was dependent on the stage of maturation. Specifically, immature LCs supported productive infection with endotheliotropic strains of HCMV to a very low degree (3–4%), whereas LC-derived mature DCs were highly susceptible to infection.Citation79 In LC-derived mature DCs, HCMV infection downregulates MHC class I and II, CD1a, CD80, CD86, CD83 and CD54, reduces the ability to stimulate a T-cell response,Citation79 lowers cell-surface expression of MHC class II, mostly by binding or penetration by HCMV particles, since conditioned medium from infected cells did not mediate this effect,Citation80 leads to a dramatic loss of dendrites and inhibits migration in response to lymphoid chemokines.Citation80 In addition, HCMV infection of LC-derived mature DCs inhibits constitutive expression of class II transcriptional regulators, most likely at the transcriptional level, resulting in reduced biosynthesis of MHC II.Citation81
HCMV Infection in Plasmacytoid DCs
Plasmacytoid DCs (pDCs), the main producers of type I IFN in response to viral infection, are essential for antiviral immunity. Circulating blood plasmacytoid DCs (bpDCs) have low susceptibility to HCMV infection, and one group suggested that bpDCs are resistant to HCMV infection in vitro.Citation82 In that study, few CD11c+ DCs isolated from peripheral blood were sensitive to in vitro HCMV infection, while immature and mature moDCs were more readily infected. Upon HCMV exposure, both bpDCs and CD11c+ DCs produced high amounts of IFN-α and exhibited increased survival. In CD11c+ DCs, production of IFN-α decreased sensitivity to HCMV; upon HCMV exposure, the cells matured and increased their levels of MHC class I, MHC class II and CD83; and even though class I and CD83 expression decreased on HCMV antigen-positive cells, the DCs retained full T-cell stimulatory capacity.Citation82
In contrast, we showed that HCMV infection of bpDCs is nonpermissive,Citation26 induces partial maturation of the cells and upregulates their expression of MHC class II and CD83. By engaging the TLR7 and/or TLR9 pathways, HCMV increased production of IFN-α and secretion of IL-6 and IL-10 ().Citation26 HCMV infection inhibited T-cell proliferation but triggered activation and proliferation of B cells by soluble factors produced by HCMV-infected bpDCs and induced plasma cell differentiation and antibody production in the presence of T cells or IL-2 ().Citation26 Even though bpDCs are relatively resistant to HCMV infection, the virus causes them to release large amounts of IFN-α, which renders other cell types more resistant to HCMV infection.Citation83 Since cmvIL-10 suppresses the production of type I IFNs by bpDCs, this may be a potential immune evasion mechanism to increase viral susceptibly of surrounding cell types.
Figure 2. HCMV infects pDCs nonpermissively. Through engagement of the TLR7 and/or TLR9 pathways, HCMV induces production of IFN-α and secretion of IL-6 and IL-10. HCMV infection in pDCs has an inhibitory effect on T-cell proliferation, but triggers B-cell activation and proliferation and antibody production. HCMV infection of pDCs has a pronounced effect on NK cells: it increases expression of NK-cell activation markers, induces production of high levels of inflammatory cytokines, and increases migration, but impairs the ability to kill target cells. IFN-α production by HCMV-infected pDCs together with chronic HCMV antigen stimulation may lead to differentiation of CD4+CD28-null T cells.
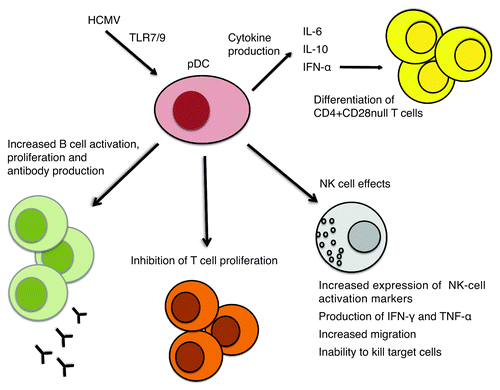
HCMV-infected pDCs can also induce NK cells to increase their expression of activation markers, produce high levels of the inflammatory cytokines IFN-γ and TNF-α and increase migration via soluble factors; however, the infected pDCs impair the cytotoxicity of NK cells ().Citation84 pDCs isolated from peripheral blood or lymphoid organs (tonsils) consist of two subpopulations that behave differently upon HCMV infection: blood-pDCs (bpDCs), which are resistant to HCMV infection, and tonsillary pDCs (tpDCs), which are fully permissive.Citation85 In tpDCs, HCMV infection reduces expression of adhesion and costimulatory molecules and the ability to allogeneically stimulate T cells and impairs IFN-α production.Citation85 In bpDCs, infection leads to activation, increases expression of MHC class I, adhesion molecules and costimulatory molecules and production of IFN-α,Citation85 but markedly reduces allogeneic T-cell stimulation. The decrease in allogeneic T-cell stimulation in infected tpDCs and bpDCs was mediated by an HCMV-induced soluble factor.
The effects of HCMV-infected pDCs on T cells, NK cells and B cells emphasize the role of pDCs in controlling innate and adaptive immune system responses and HCMV´s ability to subvert important mediators of the immune response.
HCMV-Induced Cross-Presentation by DCs
A cytotoxic T-cell response is dependent on the activation of native cytotoxic T lymphocytes (CTLs) by professional APCs, usually DCs. Since HCMV can compromise the endogenous MHC class I pathway and infect DCs to a variable degree, the immune system could take advantage of cross-presentation to evoke a CTL response. Cross-presentation occurs when the APCs present extracellular antigen on their MHC class I molecules.Citation86 Indeed, HCMV infection of fibroblasts induces cross-presentation to CD8 T cells.Citation87,Citation88 Since immature moDCs are not permissive to fibroblast-adapted laboratory strains of HCMV, cross-priming has been studied in an in vitro system that uses strain AD169. Uninfected immature moDCs internalized pp65-positive apoptotic HCMV-infected fibroblasts, which led to an anti-pp65 CD8 T-cell response, suggesting that the moDCs acquired and processed pp65 from the apoptotic fibroblasts.Citation87,Citation89 In addition, HCMV-infected fibroblasts induced maturation of moDCs and upregulated expression of CD83 and HLA class II.Citation88
Cross-Talk between NK Cells and DCs during HCMV Infection
DCs play a key role in innate immunity through bidirectional cross-talk with NK cells. The NK cell-mediated response to HCMV infection has mostly been studied in fibroblasts, so data on NK-DC crosstalk in HCMV infection are limited. In an autologous system, NK cells upregulated the surface expression of activation markers, secreted high concentrations of IFN-γ and degranulated in the presence of HCMV-infected moDCs, an effect that was mainly attributed to NKp46 and DNAM-1.Citation90 In a similar system, HCMV infection of M1 and M2 macrophages triggered NK cell cytotoxicity involving NKp46, DNAM-1 and 2B4 receptors.Citation91 Even though HCMV-infected M1 and M2 macrophages caused similar levels of NK cell degranulation, only M1 macrophages produced IL-12 and effectively activated NK cell-mediated IFN-γ secretion. Thus, distinct subsets of APCs (e.g., DCs or macrophages) trigger NK cell response through different mechanisms, highlighting the importance of studying mechanisms in a suitable cellular system.
Impact of HCMV in DCs on Clinical Pathology
HCMV infection and reactivation in the immunocompetent host
The numerous mechanisms by which HCMV avoids recognition and elimination by the host immune system may have implications for the host during acute infection or after reactivation of latent virus. In immunocompetent hosts, primary HCMV infection can be asymptomatic, although viral shedding can persist for months or, after congenital infection, for many years.Citation1,Citation92 In patients who have a normal immune response, most diagnosed primary HCMV infections cause an acute infection accompanied by a transient immunosuppression lasting weeks or months,Citation93 along with clinical symptoms of mononucleosis. The immunosuppression most likely reflects direct effects of the virus on cell types involved in the normal immune response as discussed above. Peripheral blood lymphocytes from patients with an acute HCMV infection exhibit a diminished proliferative response to mitogens and an increase in CD8+ cells that reverses the CD4/CD8 ratio.Citation94 HCMV-infected monocytes also suppress the autologous lymphocyte response to concanavalian A.Citation95
Acute HCMV infection is associated with a loss of DCs in peripheral blood
As described above, in vitro studies provide evidence that HCMV affects DCs at multiple levels to impair or enhance their functions. For example, HCMV can induce DCs to secrete proinflammatory factors, inhibit the immunostimulatory and migratory abilities of DCs, and in cooperation with virally encoded IL-10, cause long-term inhibition of DC function. However, there are only limited data on the potential relevance of HCMV infection of DCs in vivo and the resultant DC homeostasis in HCMV-infected patients. We showed that patients with HCMV-induced mononucleosis have fewer mDCs and pDCs in peripheral blood than healthy controls.Citation96 The decrease correlated with elevated levels of inflammatory mediators, in particular with high TNF-α levels, which activate DCs and endothelial cells. Further, the decreased level of DCs in blood correlated with the high levels of TNF-α and IL-8 by a hyperbolic function, suggesting a direct relationship.
At high levels, inflammatory factors facilitate alterations in DC homeostasis during primary HCMV infection, and DCs are the most potent APCs that stimulate naive T cells and modulate the effector response to infections. Therefore, the reduced numbers of circulating DCs may result from enhanced compartmentalization of these cells in peripheral tissue during HCMV infection, as in other infections.Citation97,Citation98 This scenario could lead to HCMV-induced modulation of host immunity and thereby contribute to HCMV-related diseases. If this scenario is dependent on TNF-α, the same situation may arise in acute infection, chronic inflammation or autoimmune diseases that increase TNF-α levels (e.g., sepsis, cardiovascular disease, IBD, rheumatoid arthritis and SLE). TNF-α induces DC maturation and migration, upregulates the production of vasodilatatory mediators, adhesion molecules and chemokines and alters the junctional organization of endothelial cells, thereby facilitating transendothelial migration of DCs.Citation99,Citation100
In addition, monocyte-derived DCs from symptomatic HCMV-infected immunocompetent subjects had an altered immunophenotype characterized by decreased expression of MHC class II molecules.Citation101 These DCs had an impaired ability to stimulate T-cell proliferation and a proinflammatory pattern of cyto-chemokine secretion characterized by significantly increased production of IL-1β, TNF-α, CCL2 and CCL3 and reduced secretion of the anti-inflammatory cytokine IL-10.Citation101
Reactivation of HCMV in immunocompetent hosts is associated with different types of stress (e.g., work stress or oral herpesvirus lesions), suggesting that the virus can be reactivated in the absence of a clinical diagnosed immunodeficiency.Citation102 Indeed, in multiple studies, HCMV was reactivated in more than 30% of immunocompetent patients admitted to the intensive care unit and increased both the length of hospitalization and the risk of death.Citation103,Citation104 Typically, the virus is reactivated 1–3 weeks after hospitalization.Citation104 Patients with bacterial sepsis have a greater risk of HCMV reactivation.Citation45,Citation103 Critically ill immunocompetent patients with reactivated HCMV seem to die not from HCMV viremia but from other mechanisms, such as cytopathology in the affected organ, immunopathology from the immune response to the virus and bacterial and fungal superinfections (reviewed in ref. Citation105). More studies on the specific cell types involved in the reactivation of HCMV infection under these conditions are necessary. However, continuous HCMV monitoring and prophylaxis or early treatment may reduce HCMV reactivation and secondary infection in immunocompetent patients with critical illnesses.
Effects on HCMV infection and reactivation in transplant patients
HCMV is one of the most important pathogens in recipients of stem cell and organ grafts. A key risk factor for HCMV-related disease is the serostatus of the donor and recipient; the risk is highest for HCMV-negative recipients of tissue from HCMV-positive donors.Citation106,Citation107 In transplant recipients, HCMV infection produces symptoms such as fever, bone marrow suppression and organ-invasive disease, usually of the transplanted organ itself, which also appears to be more susceptible to the direct effects of HCMV infection than native organs.Citation108
Low pretransplant levels of mDCs are associated with higher incidence of post-transplant HCMV infection and death in kidney transplant patients, but no association has been found between pDC levels and HCMV infection.Citation109 Purified DCs from kidney transplant patients with HCMV viremia contain HCMV DNA, showing that DCs indeed are infected in vivo.Citation65 In subsets of blood DCs and monocyte-derived DCs from heart-transplant patients undergoing an acute HCMV infection, both HCMV RNA and antigens could be detected,Citation110 indicating active replication. Infection was associated with an impaired immunophenotype characterized by increased secretion of IL-10 by immature DCs and reduced ability of mature DCs to stimulate allogeneic T-cell proliferation.Citation110
The ability of HCMV to infect mDCs may contribute to HCMV reactivation and pathogenesis. In allogeneic stem-cell transplant patients, certain DC-SIGN alleles are associated with HCMV reactivation (G allele of rs735240) and HCMV disease (C allele of rs2287886).Citation111 In healthy subjects, these alleles influence the expression levels of DC-SIGN on immature DCs;Citation111 HCMV infection in DCs was more efficient in subjects carrying alleles that correlate with higher DC-SIGN expression levels.
The effects of HCMV infection in transplant patients extend beyond the acute phase; such patients are more susceptible to bacterial, fungal and other opportunistic infections (for a review, see refs. Citation106–Citation108). HCMV has also been implicated in acute and chronic rejection of transplanted organs, and the relation between rejection and HCMV appears to be bidirectional, with HCMV causing rejection, and the inflammation caused by rejection increasing viral replication.Citation108,Citation112 HCMV may also mediate post-transplant diabetes mellitus, cardiovascular diseases and malignancies.Citation112 Strong evidence from epidemiological and clinical treatment studies suggests a causal relationship between HCMV and these diseases; however, the virus has been difficult to detect in the patients. Therefore, the term “indirect effects” has been used to describe the contribution of the virus to these complications. However, it is difficult to imagine how latent HCMV can affect so many different diseases. Indeed, using optimized staining protocols to examine kidney grafts after chronic rejection, we detected high levels of viral protein expression in a majority of the samples.Citation113 This finding challenges the notion that HCMV has indirect effects in this disease. Thus, HCMV may be more active in the affected tissue than previously recognized and confer direct molecular effects relevant in disease pathogenesis of chronic rejection in the graft, rather than mediating its pathogenic effects indirectly.
Evidence for a role of HCMV in common diseases: associations between HCMV, chronic inflammation and autoimmunity
Although primary HCMV infection is generally mild in immunocompetent subjects, symptomatic disease may develop, and some case reports have implied a connection with immune dysfunction and autoimmune phenomena; primary HCMV infection may trigger or exacerbate inflammation and autoimmunity in seemingly immunocompetent hosts. For example, active HCMV infection is often detected in patients with SLE, where it is associated with higher disease activity scores and has been implicated in both the development and progression of the disease (reviewed in ref. Citation114). HCMV is also associated with rheumatoid arthritis, myositis, Guillain-Barre syndrome, Wegner granulomatosis, psoriasis, vasculitis, atherosclerosis and IBD (reviewed in ref. Citation114).
HCMV infection is associated with various manifestations of autoimmunity. Primary HCMV infection often results in production of autoantibodies against endothelial cells and smooth muscle cells (i.e., antinuclear, antiphospholipid and anti-CD13 autoantibodies) (reviewed in ref. Citation114). The immunogen may be CD13, a structural component of HCMV particles; CD13-specific auto-antibodies were detected in stem cell recipients and in IBD patients who had had an HCMV infection but not in those who were HCMV negative.Citation115,Citation116 Thus, during HCMV infections, the HCMV-associated auto-antigen CD13 may become immunogenic through its presence in the viral particle. This novel mechanism of autoimmunity may be further enhanced by DCs. pDCs induce plasma cell differentiation by producing type I IFN and IL-6;Citation117 HCMV induces IFN-α secretion from bpDCs through the TLR7/TLR9 signaling pathways and induces IL-6 production,Citation26 which would increase antibody production.
Although HCMV infection sometimes seems to precede the onset of autoimmune disease, the presence of auto-antibodies during primary HCMV infection is usually not associated with clinical autoimmunity. Clinical autoimmunity likely occurs more frequently in people who are genetically predisposed to the condition, but no clear risk profile has been identified. Most likely, autoimmunity depends on the functionality of the immune system and is influenced by inherited defects in immunity, such as polymorphisms in the genes encoding TLR and mannose-binding lectin, cytokine and chemokine defects, HCMV-specific T-cell compartments and expression of programmed cell death 1 and immune-evasion genes (reviewed in ref. Citation106).
Allogeneic hematopoietic stem cell transplant patients diagnosed with HCMV infection have a significantly higher frequency of the T-1237C (TLR9) polymorphism than patients without infection,Citation118 implying a potential role for TLR9 in the recognition and response to HCMV in vivo. Patients with chronic mucocutaneous candidiasis have a higher frequency of a TLR3 variant (L412F), and an increased frequency of autoimmune disorders and severe viral infections, including HCMV infections.Citation119 These patients also have a weak proliferative response to HCMV antigens in vitro. In human moDCs, TLR3 is localized in intracellular endosomal compartments and detects pathogens after cellular uptake. This finding implies that antiviral mechanisms related to TLR3 functions may not be working properly in these patients.
HCMV may be linked to autoimmunity through several different mechanisms, such as molecular mimicry, epitope spreading, cross reactivity or virally induced expression of cryptic antigens normally hidden to the immune system.Citation114 Patients with HCMV-induced autoimmunity may be coinfected by other pathogens,Citation120 which may increase TLR activation and complement activation, resulting in an enhanced immune response. Recently, it was reported that a majority of SLE patients have antibodies to the C terminus of the HCMV protein pp65, in particular to a subfragment of pp65.Citation121 Immune reactivity against this peptide was also found in 14–20% of patients with rheumatoid arthritis, Sjögren syndrome and systemic sclerosis but only in 4% of healthy controls.Citation121 Antibodies against HCMV pp65 cross-reacted with nuclear proteins and double-stranded DNA and were therefore proposed to be pathogenic.Citation121 This hypothesis is further supported by animal experiments; in lupus-prone mice, immunization with pp65 resulted in autoantibody production and glomerulonephritis.Citation122 Immunization of BALB/c mice with the subfragment of pp65 resulted in production of multiple antibodies that recognize nuclear structures, including chromatin, centriole mitotic spindle type I/II and double-stranded DNA.Citation121 Interestingly, TLR3, TLR7 and TLR9, which induce IFN-α production upon ligand binding, are associated with SLE and linked to production of auto-antibodies and IgM rheumatoid factor.Citation123,Citation124
We recently described a previously healthy woman who had a primary HCMV infection and bacterial infection of the urinary tract. Subsequently, she developed systemic vasculitis and had extraordinarily higher plasma levels of proinflammatory cytokines, auto-antibodies and viral DNA than other patients with primary HCMV infection.Citation120 We also described a previously healthy patient who acquired a symptomatic primary HCMV infection that resulted in severe autoimmune encephalitis.Citation125 None of these patients had a family history of autoimmunity, and it is not known why they developed severe infection and subsequent clinical autoimmunity. Mice that are deficient in TLR7/9 pathways are more susceptible to murine CMV infection and have a higher mortality.Citation126 Thus, viral persistence due to an inability to clear the active infection may be connected to TLR functions. Studies are needed to further define the genetic susceptibility to HCMV-induced pathologies in patients with HCMV infection and autoimmunity.
HCMV infection in chronic inflammatory diseases
HCMV may trigger autoimmune disease in genetically susceptible people. However, the virus is more likely to contribute to autoimmune or chronic inflammatory diseases after reactivation by an inflammatory insult initiated by other antigens; thereafter, HCMV proteins would sustain and exacerbate inflammatory processes. HCMV can most likely be reactivated in monocyte-derived macrophages or moDCs in tissues with active inflammation; HCMV peptides will further drive immune reactions locally, as the immune system will consider them non-self antigens. Furthermore, viral mechanisms that induce inflammation and autoimmune reactions will further sustain and exacerbate inflammation. For example, HCMV infection induces expression of COX-2 and 5-LO and production of PGE2, LTB4 and IL-6—all potent inflammatory mediators. Through interactions with TLRs on pDCs, HCMV induces production of type I cytokines.Citation26
A better understanding of the role of HCMV in chronic inflammation would likely improve our understanding of the pathogenesis of inflammatory diseases and the potential impact of antiviral therapy on disease progression. Indeed, in many case reports, antiviral therapy appeared to result in clinical improvement of IBD patients with HCMV infection. HCMV nucleic acids and proteins are frequently found in biopsy specimens of patients with IBD.Citation127 In particular, HCMV infection should be suspected in patients with steroid-resistant ulcerative colitis.
As a target organ for HCMV, the gut is especially interesting from the perspective of immune regulation. The gut can mount an immune response against pathogenic bacteria and viruses but not against commensal bacteria. It is unclear why this is so. DCs in the gut would be the main primers of naive T cells to the invading pathogen. IBD patients appear to have lower levels of immature DCs in peripheral blood,Citation128 and these DCs have increased levels of CD86, which further increase upon stimulation ex vivo.Citation128 We showed that the loss of peripheral blood DCs correlates with high levels of TNF-α,Citation96 which increase during flare-ups of IBD and during HCMV infection.Citation45,Citation129 The apparent chronic presence of HCMV antigens in the bowel of a majority of IBD patients would challenge DCs to prime an immune response against HCMV and direct the it toward expansion of T helper 1 cells, T helper 2 cells or regulatory T cells. Regulatory T cells (Tregs), a subpopulation of CD4 T cells expressing the FoxP3 transcription factor and CD25, are involved in the control of a number immunological conditions, including autoimmunity, transplant rejection and host response to pathogens,Citation130 and also seem to be involved in the control of CMV disease. For example, impaired Treg reconstitution after allogeneic bone marrow transplantation (BMT) was suggested to be associated with HCMV viremia as well as acute graft vs. host disease.Citation131 Increased levels of Tregs seems to protect against HCMV infection after allogeneic BMT, possibly depending on the lower ability of patients with lower Treg numbers to recover their HCMV-specific CD8 T cells.Citation132 In agreement with these findings, adoptive transfer of Tregs in a murine GVHD-model led to protection against lethal MCMV infection due to MCMV-specific immunity with increased viral clearance.Citation133 In contrast, MCMV infection in vitro and in a murine BMT-model suggest that high numbers of Tregs suppress the immune response to MCMV via effects on effector T cells and by the suppression of peripheral T cell generation.Citation134,Citation135
Expansion of antigen-specific Tregs can be controlled by antigen-specific DC subsets in the peripheral tissues.Citation130 MCMV infection of mouse fibroblasts was found to stimulate the differentiation to Tregs in vitro.Citation135 Presence of HCMV antigens in different peripheral tissues during HCMV infection in autoimmune and inflammatory conditions would challenge DCs to prime an immune response against HCMV and would thereby be able to induce a Treg expansion. However, since HCMV infection in DCs impairs their function with effects on the maturation, T cell proliferation and migration, the ability of DCs to induce an HCMV-specific Treg expansion might be suppressed by the virus, thereby potentially leading to loss of viral control. Further studies would be necessary to define the effect HCMV on Tregs in patients with HCMV infection and different autoimmune and inflammatory conditions. Thus, a greater understanding of the interactions between HCMV, DCs and the resultant T-cell phenotypes expanded in autoimmune and inflammatory conditions should shed further light on the pathogenesis of the diseases.
HCMV in cardiovascular diseases
HCMV is associated with atherosclerosis, restenosis after coronary angioplasty, aortic aneurysms, transplant vascular sclerosis and other vascular diseases.Citation136 Inflammation is thought to be a key feature of these diseases, and T cells are considered to be the major disease-promoting cells, often triggered by oxidized low-density lipoproteins, which may be an auto-antigen in atherosclerotic plaques.Citation137 Since DCs direct the adaptive T-cell response, it will be interesting to assess their role in atherosclerosis. Both mDCs and pDCs are present in early lesions in healthy subjects and in atherosclerotic plaques,Citation138,Citation139 and advanced and vulnerable plaques appear to have higher numbers of DCs.Citation140 It has been suggested that different vessels have specific TLR profiles, suggesting that a vessel-specific risk may exist in inflammatory and pathogen-mediated vascular diseases.Citation141,Citation142
Although HCMV has been detected in atherosclerotic plaques,Citation143,Citation144 it has not been determined whether HCMV is an epiphenomenon of vascular diseases or contributes to the pathogenesis.Citation3,Citation136 Most likely, HCMV acts locally in the plaque and does not produce signs of systemic infection in most patients with atherosclerosis. However, we found that active HCMV replication is more common in monocytes from patients with stable or unstable coronary artery disease; specifically, CMV RNA was detected in blood monocytes in 15% of patients with acute coronary syndrome and 10% of those with stable angina, but in only 2% of age- and sex-matched controls.Citation145 Furthermore, two large clinical studies of more than 14,000 and 1,400 subjects, respectively, provide compelling evidence that high HCMV antibody titers and enhanced CRP levels are strongly predictive of cardiovascular mortality.Citation146,Citation147 Thus, HCMV antibody levels may be a hitherto unrecognized prognostic factor for cardiovascular events and a useful biomarker for identifying patients at risk for MI and stroke. The relationship of high HCMV IgG antibody levels to mortality may be mediated mainly by IL-6 and TNF-α,Citation146 further supporting the importance of a high-inflammatory state in patients with clinical complications of HCMV infection. We showed that high TNF-α levels are linked to lower levels of DCs in the blood of patients with HCMV mononucleosis. HCMV induces production of IL-6 and TNF-α, and TNF-α acts directly on the HCMV immediately early promoter to drive reactivation and replication of the virus.Citation148 TNF-α levels are also elevated in several chronic inflammatory and autoimmune diseases and used as a target for therapy.
CD28-null T cells: a novel T-cell population associated with chronic inflammation in HCMV-infected patients
Emerging evidence implies that HCMV can be reactivated silently in immunocompetent subjects, leading to expansion of memory T cells that lose expression of the co-stimulatory molecules CD27 and CD28 and express CD57 and the NK cell marker CD244. This population, often referred to as CD28-null T cells, expands with HCMV infection, increasing age and during inflammatory conditions such as myositis, rheumatoid arthritis, IBD, Wegner granulomatosis, SLE, atherosclerosis and chronic transplant rejection.Citation114,Citation149-Citation152 These T cells are mainly directed against HCMV and provide high inflammatory activity, as they contain high perforin levels and produce high levels of IFN-γ and TNF-α upon stimulation,Citation153 and thereby contribute to inflammation. However, CD4+CD27–CD28– T cells that are in vitro stimulated with HCMV was shown to exhibit a Treg phenotype and inhibit de novo HCMV-specific proliferation of autologous PBMC.Citation154 Thus, their role in pathogenesis remains obscure. While CD8+ cells lacking CD28 are described in patients who are infected with HCMV, HIV, hepatitis C and EBV,Citation155 CD4+CD28– cells exist only in HCMV-infected individuals.Citation149 Since most carriers of HIV, hepatitis C or EBV also are HCMV positive, it is possible that HCMV drives the expansion of these cells by unknown mechanisms.Citation156 Experimental data suggest that pDCs and IFN-α are necessary to induce differentiation of CD28-null T cells ().Citation156 However, this scenario would exist during many microbial infections, and, thus, it is not known why CD4+CD28– T cells are present only in HCMV-infected individuals and what the role is of these cells.
Conclusions
DCs play a crucial role in initiating and maintaining immune responses, and HCMV infection of DCs may inhibit their functions, lead to virus-induced immunopathology, enable the virus to persist in its host and control reactivation of the virus. Viral infection of progenitor cells in the bone marrow and further differentiated cells of myeloid and lymphoid origin, including multiple DC subsets, may contribute to HCMV-induced immunosuppression in patients with primary HCMV infection and in immunosuppressed patients with HCMV disease, and increase the risk for invasive fungal and bacterial infections. Increasing numbers of patients are being treated with immunosuppressants, and there is emerging evidence of an active HCMV infection in many inflammatory diseases and cancer. Thus, further research on the interaction of HCMV and DCs, is needed to understand the role of HCMV in the pathogenesis of these diseases and to develop new therapies that target this interaction.
Acknowledgments
The work was supported by grants from the Wenner-Gren Foundations (SGR), Torsten Söderbergs Foundation, Stockholm County Council and the Swedish Medical Research Foundation in Sweden. The authors thank Stephen Ordway for editorial assistance.
References
- Britt WA, Alford CA. Cytomegalovirus. In: Fields BN, Roizman B, Knipe DM, Melnick JL, Howley PM, Chanock RM, et al., eds. Fields Virology. Philiadelphia: Lippincott-Raven Publishers, 1996:2493-523.
- Pass RF. Cytomegalovirus. In: Knipe DM, Howley PM, eds. Fields Virology. Philadephia: Lippincott Williams & Wilkins, 2001:2675-706.
- Söderberg-Nauclér C. Does cytomegalovirus play a causative role in the development of various inflammatory diseases and cancer?. J Intern Med 2006; 259:219 - 46; http://dx.doi.org/10.1111/j.1365-2796.2006.01618.x; PMID: 16476101
- Tomazin R, Boname J, Hegde NR, Lewinsohn DM, Altschuler Y, Jones TR, et al. Cytomegalovirus US2 destroys two components of the MHC class II pathway, preventing recognition by CD4+ T cells. Nat Med 1999; 5:1039 - 43; http://dx.doi.org/10.1038/12478; PMID: 10470081
- Odeberg J, Plachter B, Brandén L, Söderberg-Nauclér C. Human cytomegalovirus protein pp65 mediates accumulation of HLA-DR in lysosomes and destruction of the HLA-DR alpha-chain. Blood 2003; 101:4870 - 7; http://dx.doi.org/10.1182/blood-2002-05-1504; PMID: 12609847
- Hegde NR, Tomazin RA, Wisner TW, Dunn C, Boname JM, Lewinsohn DM, et al. Inhibition of HLA-DR assembly, transport, and loading by human cytomegalovirus glycoprotein US3: a novel mechanism for evading major histocompatibility complex class II antigen presentation. J Virol 2002; 76:10929 - 41; http://dx.doi.org/10.1128/JVI.76.21.10929-10941.2002; PMID: 12368336
- Loenen WA, Bruggeman CA, Wiertz EJ. Immune evasion by human cytomegalovirus: lessons in immunology and cell biology. Semin Immunol 2001; 13:41 - 9; http://dx.doi.org/10.1006/smim.2001.0294; PMID: 11289798
- Noriega V, Redmann V, Gardner T, Tortorella D. Diverse immune evasion strategies by human cytomegalovirus. Immunol Res 2012; http://dx.doi.org/10.1007/s12026-012-8304-8; PMID: 22454101
- Kim S, Lee S, Shin J, Kim Y, Evnouchidou I, Kim D, et al. Human cytomegalovirus microRNA miR-US4-1 inhibits CD8(+) T cell responses by targeting the aminopeptidase ERAP1. Nat Immunol 2011; 12:984 - 91; http://dx.doi.org/10.1038/ni.2097; PMID: 21892175
- Hansen SG, Powers CJ, Richards R, Ventura AB, Ford JC, Siess D, et al. Evasion of CD8+ T cells is critical for superinfection by cytomegalovirus. Science 2010; 328:102 - 6; http://dx.doi.org/10.1126/science.1185350; PMID: 20360110
- Wilkinson GW, Tomasec P, Stanton RJ, Armstrong M, Prod’homme V, Aicheler R, et al. Modulation of natural killer cells by human cytomegalovirus. J Clin Virol 2008; 41:206 - 12; http://dx.doi.org/10.1016/j.jcv.2007.10.027; PMID: 18069056
- Beck S, Barrell BG. Human cytomegalovirus encodes a glycoprotein homologous to MHC class-I antigens. Nature 1988; 331:269 - 72; http://dx.doi.org/10.1038/331269a0; PMID: 2827039
- Odeberg J, Cerboni C, Browne H, Kärre K, Möller E, Carbone E, et al. Human cytomegalovirus (HCMV)-infected endothelial cells and macrophages are less susceptible to natural killer lysis independent of the downregulation of classical HLA class I molecules or expression of the HCMV class I homologue, UL18. Scand J Immunol 2002; 55:149 - 61; http://dx.doi.org/10.1046/j.1365-3083.2002.01025.x; PMID: 11896931
- Belz GT, Nutt SL. Transcriptional programming of the dendritic cell network. Nat Rev Immunol 2012; 12:101 - 13; http://dx.doi.org/10.1038/nri3149; PMID: 22273772
- Liu K, Nussenzweig MC. Origin and development of dendritic cells. Immunol Rev 2010; 234:45 - 54; http://dx.doi.org/10.1111/j.0105-2896.2009.00879.x; PMID: 20193011
- Sallusto F, Lanzavecchia A. Efficient presentation of soluble antigen by cultured human dendritic cells is maintained by granulocyte/macrophage colony-stimulating factor plus interleukin 4 and downregulated by tumor necrosis factor alpha. J Exp Med 1994; 179:1109 - 18; http://dx.doi.org/10.1084/jem.179.4.1109; PMID: 8145033
- Ross MJ, Auger JA. The biology of the macrophage. In: Burke B, Lewis CE, eds. The Macrophage. Oxford: Oxford University Press, 2002:1-72.
- Söderberg-Nauclér C, Streblow DN, Fish KN, Allan-Yorke J, Smith PP, Nelson JA. Reactivation of latent human cytomegalovirus in CD14(+) monocytes is differentiation dependent. J Virol 2001; 75:7543 - 54; http://dx.doi.org/10.1128/JVI.75.16.7543-7554.2001; PMID: 11462026
- Verreck FA, de Boer T, Langenberg DM, Hoeve MA, Kramer M, Vaisberg E, et al. Human IL-23-producing type 1 macrophages promote but IL-10-producing type 2 macrophages subvert immunity to (myco)bacteria. Proc Natl Acad Sci USA 2004; 101:4560 - 5; http://dx.doi.org/10.1073/pnas.0400983101; PMID: 15070757
- Swiecki M, Colonna M. Unraveling the functions of plasmacytoid dendritic cells during viral infections, autoimmunity, and tolerance. Immunol Rev 2010; 234:142 - 62; http://dx.doi.org/10.1111/j.0105-2896.2009.00881.x; PMID: 20193017
- Villadangos JA, Young L. Antigen-presentation properties of plasmacytoid dendritic cells. Immunity 2008; 29:352 - 61; http://dx.doi.org/10.1016/j.immuni.2008.09.002; PMID: 18799143
- Gilliet M, Cao W, Liu YJ. Plasmacytoid dendritic cells: sensing nucleic acids in viral infection and autoimmune diseases. Nat Rev Immunol 2008; 8:594 - 606; http://dx.doi.org/10.1038/nri2358; PMID: 18641647
- Krug A, Luker GD, Barchet W, Leib DA, Akira S, Colonna M. Herpes simplex virus type 1 activates murine natural interferon-producing cells through toll-like receptor 9. Blood 2004; 103:1433 - 7; http://dx.doi.org/10.1182/blood-2003-08-2674; PMID: 14563635
- Lund J, Sato A, Akira S, Medzhitov R, Iwasaki A. Toll-like receptor 9-mediated recognition of Herpes simplex virus-2 by plasmacytoid dendritic cells. J Exp Med 2003; 198:513 - 20; http://dx.doi.org/10.1084/jem.20030162; PMID: 12900525
- Krug A, French AR, Barchet W, Fischer JA, Dzionek A, Pingel JT, et al. TLR9-dependent recognition of MCMV by IPC and DC generates coordinated cytokine responses that activate antiviral NK cell function. Immunity 2004; 21:107 - 19; http://dx.doi.org/10.1016/j.immuni.2004.06.007; PMID: 15345224
- Varani S, Cederarv M, Feld S, Tammik C, Frascaroli G, Landini MP, et al. Human cytomegalovirus differentially controls B cell and T cell responses through effects on plasmacytoid dendritic cells. J Immunol 2007; 179:7767 - 76; PMID: 18025223
- Gerna G, Zipeto D, Percivalle E, Parea M, Revello MG, Maccario R, et al. Human cytomegalovirus infection of the major leukocyte subpopulations and evidence for initial viral replication in polymorphonuclear leukocytes from viremic patients. J Infect Dis 1992; 166:1236 - 44; http://dx.doi.org/10.1093/infdis/166.6.1236; PMID: 1331248
- Taylor-Wiedeman J, Sissons JG, Borysiewicz LK, Sinclair JH. Monocytes are a major site of persistence of human cytomegalovirus in peripheral blood mononuclear cells. J Gen Virol 1991; 72:2059 - 64; http://dx.doi.org/10.1099/0022-1317-72-9-2059; PMID: 1654370
- Ibanez CE, Schrier R, Ghazal P, Wiley C, Nelson JA. Human cytomegalovirus productively infects primary differentiated macrophages. J Virol 1991; 65:6581 - 8; PMID: 1658363
- Taylor-Wiedeman J, Sissons P, Sinclair J. Induction of endogenous human cytomegalovirus gene expression after differentiation of monocytes from healthy carriers. J Virol 1994; 68:1597 - 604; PMID: 8107221
- Minton EJ, Tysoe C, Sinclair JH, Sissons JG. Human cytomegalovirus infection of the monocyte/macrophage lineage in bone marrow. J Virol 1994; 68:4017 - 21; PMID: 8189535
- Einhorn L, Ost A. Cytomegalovirus infection of human blood cells. J Infect Dis 1984; 149:207 - 14; http://dx.doi.org/10.1093/infdis/149.2.207; PMID: 6321607
- Gnann JW Jr., Ahlmén J, Svalander C, Olding L, Oldstone MB, Nelson JA. Inflammatory cells in transplanted kidneys are infected by human cytomegalovirus. Am J Pathol 1988; 132:239 - 48; PMID: 2840830
- Fish KN, Depto AS, Moses AV, Britt W, Nelson JA. Growth kinetics of human cytomegalovirus are altered in monocyte-derived macrophages. J Virol 1995; 69:3737 - 43; PMID: 7745721
- Söderberg-Nauclér C, Fish KN, Nelson JA. Growth of human cytomegalovirus in primary macrophages. Methods 1998; 16:126 - 38; http://dx.doi.org/10.1006/meth.1998.0650; PMID: 9774522
- Sindre H, Tjøonnfjord GE, Rollag H, Ranneberg-Nilsen T, Veiby OP, Beck S, et al. Human cytomegalovirus suppression of and latency in early hematopoietic progenitor cells. Blood 1996; 88:4526 - 33; PMID: 8977244
- Mendelson M, Monard S, Sissons P, Sinclair J. Detection of endogenous human cytomegalovirus in CD34+ bone marrow progenitors. J Gen Virol 1996; 77:3099 - 102; http://dx.doi.org/10.1099/0022-1317-77-12-3099; PMID: 9000102
- Movassagh M, Gozlan J, Senechal B, Baillou C, Petit JC, Lemoine FM. Direct infection of CD34+ progenitor cells by human cytomegalovirus: evidence for inhibition of hematopoiesis and viral replication. Blood 1996; 88:1277 - 83; PMID: 8695845
- Khaiboullina SF, Maciejewski JP, Crapnell K, Spallone PA, Dean Stock A, Pari GS, et al. Human cytomegalovirus persists in myeloid progenitors and is passed to the myeloid progeny in a latent form. Br J Haematol 2004; 126:410 - 7; http://dx.doi.org/10.1111/j.1365-2141.2004.05056.x; PMID: 15257715
- Reeves MB, MacAry PA, Lehner PJ, Sissons JG, Sinclair JH. Latency, chromatin remodeling, and reactivation of human cytomegalovirus in the dendritic cells of healthy carriers. Proc Natl Acad Sci USA 2005; 102:4140 - 5; http://dx.doi.org/10.1073/pnas.0408994102; PMID: 15738399
- Söderberg-Nauclér C, Fish KN, Nelson JA. Reactivation of latent human cytomegalovirus by allogeneic stimulation of blood cells from healthy donors. Cell 1997; 91:119 - 26; http://dx.doi.org/10.1016/S0092-8674(01)80014-3; PMID: 9335340
- Söderberg-Nauclér C, Fish KN, Nelson JA. Interferon-gamma and tumor necrosis factor-alpha specifically induce formation of cytomegalovirus-permissive monocyte-derived macrophages that are refractory to the antiviral activity of these cytokines. J Clin Invest 1997; 100:3154 - 63; http://dx.doi.org/10.1172/JCI119871; PMID: 9399963
- Reeves MB, Compton T. Inhibition of inflammatory interleukin-6 activity via extracellular signal-regulated kinase-mitogen-activated protein kinase signaling antagonizes human cytomegalovirus reactivation from dendritic cells. J Virol 2011; 85:12750 - 8; http://dx.doi.org/10.1128/JVI.05878-11; PMID: 21937636
- Hargett D, Shenk TE. Experimental human cytomegalovirus latency in CD14+ monocytes. Proc Natl Acad Sci USA 2010; 107:20039 - 44; http://dx.doi.org/10.1073/pnas.1014509107; PMID: 21041645
- Döcke WD, Prösch S, Fietze E, Kimel V, Zuckermann H, Klug C, et al. Cytomegalovirus reactivation and tumour necrosis factor. Lancet 1994; 343:268 - 9; http://dx.doi.org/10.1016/S0140-6736(94)91116-9; PMID: 7905100
- Kutza AS, Muhl E, Hackstein H, Kirchner H, Bein G. High incidence of active cytomegalovirus infection among septic patients. Clin Infect Dis 1998; 26:1076 - 82; http://dx.doi.org/10.1086/520307; PMID: 9597229
- Asadullah K, Prösch S, Audring H, Büttnerova I, Volk HD, Sterry W, et al. A high prevalence of cytomegalovirus antigenaemia in patients with moderate to severe chronic plaque psoriasis: an association with systemic tumour necrosis factor alpha overexpression. Br J Dermatol 1999; 141:94 - 102; http://dx.doi.org/10.1046/j.1365-2133.1999.02926.x; PMID: 10417521
- Gredmark S, Söderberg-Nauclér C. Human cytomegalovirus inhibits differentiation of monocytes into dendritic cells with the consequence of depressed immunological functions. J Virol 2003; 77:10943 - 56; http://dx.doi.org/10.1128/JVI.77.20.10943-10956.2003; PMID: 14512544
- Carlier J, Martin H, Mariamé B, Rauwel B, Mengelle C, Weclawiak H, et al. Paracrine inhibition of GM-CSF signaling by human cytomegalovirus in monocytes differentiating to dendritic cells. Blood 2011; 118:6783 - 92; http://dx.doi.org/10.1182/blood-2011-02-337956; PMID: 22031867
- Gredmark S, Tilburgs T, Söderberg-Nauclér C. Human cytomegalovirus inhibits cytokine-induced macrophage differentiation. J Virol 2004; 78:10378 - 89; http://dx.doi.org/10.1128/JVI.78.19.10378-10389.2004; PMID: 15367604
- Söderberg C, Giugni TD, Zaia JA, Larsson S, Wahlberg JM, Möller E. CD13 (human aminopeptidase N) mediates human cytomegalovirus infection. J Virol 1993; 67:6576 - 85; PMID: 8105105
- Gredmark S, Britt WB, Xie X, Lindbom L, Söderberg-Nauclér C. Human cytomegalovirus induces inhibition of macrophage differentiation by binding to human aminopeptidase N/CD13. J Immunol 2004; 173:4897 - 907; PMID: 15470031
- Mutimer D, Mirza D, Shaw J, O’Donnell K, Elias E. Enhanced (cytomegalovirus) viral replication associated with septic bacterial complications in liver transplant recipients. Transplantation 1997; 63:1411 - 5; http://dx.doi.org/10.1097/00007890-199705270-00007; PMID: 9175802
- van den Berg AP, Klompmaker IJ, Haagsma EB, Peeters PM, Meerman L, Verwer R, et al. Evidence for an increased rate of bacterial infections in liver transplant patients with cytomegalovirus infection. Clin Transplant 1996; 10:224 - 31; PMID: 8664524
- Paya CV, Wiesner RH, Hermans PE, Larson-Keller JJ, Ilstrup DM, Krom RA, et al. Risk factors for cytomegalovirus and severe bacterial infections following liver transplantation: a prospective multivariate time-dependent analysis. J Hepatol 1993; 18:185 - 95; http://dx.doi.org/10.1016/S0168-8278(05)80245-4; PMID: 8409334
- Riegler S, Hebart H, Einsele H, Brossart P, Jahn G, Sinzger C. Monocyte-derived dendritic cells are permissive to the complete replicative cycle of human cytomegalovirus. J Gen Virol 2000; 81:393 - 9; PMID: 10644837
- Sinzger C, Digel M, Jahn G. Cytomegalovirus cell tropism. Curr Top Microbiol Immunol 2008; 325:63 - 83; http://dx.doi.org/10.1007/978-3-540-77349-8_4; PMID: 18637500
- Sinzger C, Hahn G, Digel M, Katona R, Sampaio KL, Messerle M, et al. Cloning and sequencing of a highly productive, endotheliotropic virus strain derived from human cytomegalovirus TB40/E. J Gen Virol 2008; 89:359 - 68; http://dx.doi.org/10.1099/vir.0.83286-0; PMID: 18198366
- Gerna G, Percivalle E, Lilleri D, Lozza L, Fornara C, Hahn G, et al. Dendritic-cell infection by human cytomegalovirus is restricted to strains carrying functional UL131-128 genes and mediates efficient viral antigen presentation to CD8+ T cells. J Gen Virol 2005; 86:275 - 84; http://dx.doi.org/10.1099/vir.0.80474-0; PMID: 15659746
- Hahn G, Revello MG, Patrone M, Percivalle E, Campanini G, Sarasini A, et al. Human cytomegalovirus UL131-128 genes are indispensable for virus growth in endothelial cells and virus transfer to leukocytes. J Virol 2004; 78:10023 - 33; http://dx.doi.org/10.1128/JVI.78.18.10023-10033.2004; PMID: 15331735
- Halary F, Amara A, Lortat-Jacob H, Messerle M, Delaunay T, Houlès C, et al. Human cytomegalovirus binding to DC-SIGN is required for dendritic cell infection and target cell trans-infection. Immunity 2002; 17:653 - 64; http://dx.doi.org/10.1016/S1074-7613(02)00447-8; PMID: 12433371
- Plazolles N, Humbert JM, Vachot L, Verrier B, Hocke C, Halary F. Pivotal advance: The promotion of soluble DC-SIGN release by inflammatory signals and its enhancement of cytomegalovirus-mediated cis-infection of myeloid dendritic cells. J Leukoc Biol 2011; 89:329 - 42; http://dx.doi.org/10.1189/jlb.0710386; PMID: 20940323
- Haspot F, Lavault A, Sinzger C, Laib Sampaio K, Stierhof YD, Pilet P, et al. Human cytomegalovirus entry into dendritic cells occurs via a macropinocytosis-like pathway in a pH-independent and cholesterol-dependent manner. PLoS One 2012; 7:e34795; http://dx.doi.org/10.1371/journal.pone.0034795; PMID: 22496863
- Raftery MJ, Schwab M, Eibert SM, Samstag Y, Walczak H, Schönrich G. Targeting the function of mature dendritic cells by human cytomegalovirus: a multilayered viral defense strategy. Immunity 2001; 15:997 - 1009; http://dx.doi.org/10.1016/S1074-7613(01)00239-4; PMID: 11754820
- Beck K, Meyer-König U, Weidmann M, Nern C, Hufert FT. Human cytomegalovirus impairs dendritic cell function: a novel mechanism of human cytomegalovirus immune escape. Eur J Immunol 2003; 33:1528 - 38; http://dx.doi.org/10.1002/eji.200323612; PMID: 12778470
- Moutaftsi M, Mehl AM, Borysiewicz LK, Tabi Z. Human cytomegalovirus inhibits maturation and impairs function of monocyte-derived dendritic cells. Blood 2002; 99:2913 - 21; http://dx.doi.org/10.1182/blood.V99.8.2913; PMID: 11929782
- Mezger M, Bonin M, Kessler T, Gebhardt F, Einsele H, Loeffler J. Toll-like receptor 3 has no critical role during early immune response of human monocyte-derived dendritic cells after infection with the human cytomegalovirus strain TB40E. Viral Immunol 2009; 22:343 - 51; http://dx.doi.org/10.1089/vim.2009.0011; PMID: 19951172
- Sénéchal B, Boruchov AM, Reagan JL, Hart DN, Young JW. Infection of mature monocyte-derived dendritic cells with human cytomegalovirus inhibits stimulation of T-cell proliferation via the release of soluble CD83. Blood 2004; 103:4207 - 15; http://dx.doi.org/10.1182/blood-2003-12-4350; PMID: 14962896
- Wagner CS, Walther-Jallow L, Buentke E, Ljunggren HG, Achour A, Chambers BJ. Human cytomegalovirus-derived protein UL18 alters the phenotype and function of monocyte-derived dendritic cells. J Leukoc Biol 2008; 83:56 - 63; http://dx.doi.org/10.1189/jlb.0307181; PMID: 17898320
- Moutaftsi M, Brennan P, Spector SA, Tabi Z. Impaired lymphoid chemokine-mediated migration due to a block on the chemokine receptor switch in human cytomegalovirus-infected dendritic cells. J Virol 2004; 78:3046 - 54; http://dx.doi.org/10.1128/JVI.78.6.3046-3054.2004; PMID: 14990723
- Varani S, Frascaroli G, Homman-Loudiyi M, Feld S, Landini MP, Söderberg-Nauclér C. Human cytomegalovirus inhibits the migration of immature dendritic cells by down-regulating cell-surface CCR1 and CCR5. J Leukoc Biol 2005; 77:219 - 28; http://dx.doi.org/10.1189/jlb.0504301; PMID: 15522919
- Kotenko SV, Saccani S, Izotova LS, Mirochnitchenko OV, Pestka S. Human cytomegalovirus harbors its own unique IL-10 homolog (cmvIL-10). Proc Natl Acad Sci USA 2000; 97:1695 - 700; http://dx.doi.org/10.1073/pnas.97.4.1695; PMID: 10677520
- Jenkins C, Abendroth A, Slobedman B. A novel viral transcript with homology to human interleukin-10 is expressed during latent human cytomegalovirus infection. J Virol 2004; 78:1440 - 7; http://dx.doi.org/10.1128/JVI.78.3.1440-1447.2004; PMID: 14722299
- Jenkins C, Garcia W, Godwin MJ, Spencer JV, Stern JL, Abendroth A, et al. Immunomodulatory properties of a viral homolog of human interleukin-10 expressed by human cytomegalovirus during the latent phase of infection. J Virol 2008; 82:3736 - 50; http://dx.doi.org/10.1128/JVI.02173-07; PMID: 18216121
- Avdic S, Cao JZ, Cheung AK, Abendroth A, Slobedman B. Viral interleukin-10 expressed by human cytomegalovirus during the latent phase of infection modulates latently infected myeloid cell differentiation. J Virol 2011; 85:7465 - 71; http://dx.doi.org/10.1128/JVI.00088-11; PMID: 21593144
- Chang WL, Baumgarth N, Yu D, Barry PA. Human cytomegalovirus-encoded interleukin-10 homolog inhibits maturation of dendritic cells and alters their functionality. J Virol 2004; 78:8720 - 31; http://dx.doi.org/10.1128/JVI.78.16.8720-8731.2004; PMID: 15280480
- Raftery MJ, Wieland D, Gronewald S, Kraus AA, Giese T, Schönrich G. Shaping phenotype, function, and survival of dendritic cells by cytomegalovirus-encoded IL-10. J Immunol 2004; 173:3383 - 91; PMID: 15322202
- Raftery MJ, Hitzler M, Winau F, Giese T, Plachter B, Kaufmann SH, et al. Inhibition of CD1 antigen presentation by human cytomegalovirus. J Virol 2008; 82:4308 - 19; http://dx.doi.org/10.1128/JVI.01447-07; PMID: 18287231
- Hertel L, Lacaille VG, Strobl H, Mellins ED, Mocarski ES. Susceptibility of immature and mature Langerhans cell-type dendritic cells to infection and immunomodulation by human cytomegalovirus. J Virol 2003; 77:7563 - 74; http://dx.doi.org/10.1128/JVI.77.13.7563-7574.2003; PMID: 12805456
- Lee AW, Hertel L, Louie RK, Burster T, Lacaille V, Pashine A, et al. Human cytomegalovirus alters localization of MHC class II and dendrite morphology in mature Langerhans cells. J Immunol 2006; 177:3960 - 71; PMID: 16951359
- Lee AW, Wang N, Hornell TM, Harding JJ, Deshpande C, Hertel L, et al. Human cytomegalovirus decreases constitutive transcription of MHC class II genes in mature Langerhans cells by reducing CIITA transcript levels. Mol Immunol 2011; 48:1160 - 7; http://dx.doi.org/10.1016/j.molimm.2011.02.010; PMID: 21458073
- Kvale EO, Dalgaard J, Lund-Johansen F, Rollag H, Farkas L, Midtvedt K, et al. CD11c+ dendritic cells and plasmacytoid DCs are activated by human cytomegalovirus and retain efficient T cell-stimulatory capability upon infection. Blood 2006; 107:2022 - 9; http://dx.doi.org/10.1182/blood-2005-05-2016; PMID: 16269620
- Chang WL, Barry PA, Szubin R, Wang D, Baumgarth N. Human cytomegalovirus suppresses type I interferon secretion by plasmacytoid dendritic cells through its interleukin 10 homolog. Virology 2009; 390:330 - 7; http://dx.doi.org/10.1016/j.virol.2009.05.013; PMID: 19524994
- Cederarv M, Söderberg-Nauclér C, Odeberg J. HCMV infection of PDCs deviates the NK cell response into cytokine-producing cells unable to perform cytotoxicity. Immunobiology 2009; 214:331 - 41; http://dx.doi.org/10.1016/j.imbio.2008.10.009; PMID: 19152985
- Schneider K, Meyer-Koenig U, Hufert FT. Human cytomegalovirus impairs the function of plasmacytoid dendritic cells in lymphoid organs. PLoS One 2008; 3:e3482; http://dx.doi.org/10.1371/journal.pone.0003482; PMID: 18941519
- Kurts C, Robinson BW, Knolle PA. Cross-priming in health and disease. Nat Rev Immunol 2010; 10:403 - 14; http://dx.doi.org/10.1038/nri2780; PMID: 20498667
- Arrode G, Boccaccio C, Lulé J, Allart S, Moinard N, Abastado JP, et al. Incoming human cytomegalovirus pp65 (UL83) contained in apoptotic infected fibroblasts is cross-presented to CD8(+) T cells by dendritic cells. J Virol 2000; 74:10018 - 24; http://dx.doi.org/10.1128/JVI.74.21.10018-10024.2000; PMID: 11024130
- Tabi Z, Moutaftsi M, Borysiewicz LK. Human cytomegalovirus pp65- and immediate early 1 antigen-specific HLA class I-restricted cytotoxic T cell responses induced by cross-presentation of viral antigens. J Immunol 2001; 166:5695 - 703; PMID: 11313411
- Mandron M, Martin H, Bonjean B, Lulé J, Tartour E, Davrinche C. Dendritic cell-induced apoptosis of human cytomegalovirus-infected fibroblasts promotes cross-presentation of pp65 to CD8+ T cells. J Gen Virol 2008; 89:78 - 86; http://dx.doi.org/10.1099/vir.0.83278-0; PMID: 18089731
- Magri G, Muntasell A, Romo N, Sáez-Borderías A, Pende D, Geraghty DE, et al. NKp46 and DNAM-1 NK-cell receptors drive the response to human cytomegalovirus-infected myeloid dendritic cells overcoming viral immune evasion strategies. Blood 2011; 117:848 - 56; http://dx.doi.org/10.1182/blood-2010-08-301374; PMID: 21030563
- Romo N, Magri G, Muntasell A, Heredia G, Baía D, Angulo A, et al. Natural killer cell-mediated response to human cytomegalovirus-infected macrophages is modulated by their functional polarization. J Leukoc Biol 2011; 90:717 - 26; http://dx.doi.org/10.1189/jlb.0311171; PMID: 21742939
- Zanghellini F, Boppana SB, Emery VC, Griffiths PD, Pass RF. Asymptomatic primary cytomegalovirus infection: virologic and immunologic features. J Infect Dis 1999; 180:702 - 7; http://dx.doi.org/10.1086/314939; PMID: 10438357
- Naniche D, Oldstone MB. Generalized immunosuppression: how viruses undermine the immune response. Cell Mol Life Sci 2000; 57:1399 - 407; http://dx.doi.org/10.1007/PL00000625; PMID: 11078019
- Carney WP, Rubin RH, Hoffman RA, Hansen WP, Healey K, Hirsch MS. Analysis of T lymphocyte subsets in cytomegalovirus mononucleosis. J Immunol 1981; 126:2114 - 6; PMID: 6262407
- Carney WP, Hirsch MS. Mechanisms of immunosuppression in cytomegalovirus mononucleosis. II. Virus-monocyte interactions. J Infect Dis 1981; 144:47 - 54; http://dx.doi.org/10.1093/infdis/144.1.47; PMID: 6267142
- Varani S, Rossini G, Mastroianni A, Tammik C, Frascaroli G, Landini MP, et al. High TNF-alpha and IL-8 levels predict low blood dendritic cell counts in primary cytomegalovirus infection. J Clin Virol 2012; 53:360 - 3; http://dx.doi.org/10.1016/j.jcv.2011.12.028; PMID: 22257833
- Kunitani H, Shimizu Y, Murata H, Higuchi K, Watanabe A. Phenotypic analysis of circulating and intrahepatic dendritic cell subsets in patients with chronic liver diseases. J Hepatol 2002; 36:734 - 41; http://dx.doi.org/10.1016/S0168-8278(02)00062-4; PMID: 12044522
- Dillon SM, Robertson KB, Pan SC, Mawhinney S, Meditz AL, Folkvord JM, et al. Plasmacytoid and myeloid dendritic cells with a partial activation phenotype accumulate in lymphoid tissue during asymptomatic chronic HIV-1 infection. J Acquir Immune Defic Syndr 2008; 48:1 - 12; http://dx.doi.org/10.1097/QAI.0b013e3181664b60; PMID: 18300699
- Sallusto F, Schaerli P, Loetscher P, Schaniel C, Lenig D, Mackay CR, et al. Rapid and coordinated switch in chemokine receptor expression during dendritic cell maturation. Eur J Immunol 1998; 28:2760 - 9; http://dx.doi.org/10.1002/(SICI)1521-4141(199809)28:09<2760::AID-IMMU2760>3.0.CO;2-N; PMID: 9754563
- Duperray A, Mantovani A, Introna M, Dejana E. Endothelial cell regulation of leukocyte infiltration in inflammatory tissues. Mediators Inflamm 1995; 4:322 - 30; http://dx.doi.org/10.1155/S0962935195000524; PMID: 18475659
- Frascaroli G, Varani S, Mastroianni A, Britton S, Gibellini D, Rossini G, et al. Dendritic cell function in cytomegalovirus-infected patients with mononucleosis. J Leukoc Biol 2006; 79:932 - 40; http://dx.doi.org/10.1189/jlb.0905499; PMID: 16501053
- Toro AI, Ossa J. PCR activity of CMV in healthy CMV-seropositive individuals: does latency need redefinition?. Res Virol 1996; 147:233 - 8; http://dx.doi.org/10.1016/0923-2516(96)89654-3; PMID: 8837231
- Heininger A, Jahn G, Engel C, Notheisen T, Unertl K, Hamprecht K. Human cytomegalovirus infections in nonimmunosuppressed critically ill patients. Crit Care Med 2001; 29:541 - 7; http://dx.doi.org/10.1097/00003246-200103000-00012; PMID: 11373417
- Limaye AP, Kirby KA, Rubenfeld GD, Leisenring WM, Bulger EM, Neff MJ, et al. Cytomegalovirus reactivation in critically ill immunocompetent patients. JAMA 2008; 300:413 - 22; http://dx.doi.org/10.1001/jama.300.4.413; PMID: 18647984
- Cook CH, Trgovcich J. Cytomegalovirus reactivation in critically ill immunocompetent hosts: a decade of progress and remaining challenges. Antiviral Res 2011; 90:151 - 9; http://dx.doi.org/10.1016/j.antiviral.2011.03.179; PMID: 21439328
- Razonable RR. Cytomegalovirus infection after liver transplantation: current concepts and challenges. World J Gastroenterol 2008; 14:4849 - 60; http://dx.doi.org/10.3748/wjg.14.4849; PMID: 18756591
- Nichols WG, Corey L, Gooley T, Davis C, Boeckh M. High risk of death due to bacterial and fungal infection among cytomegalovirus (CMV)-seronegative recipients of stem cell transplants from seropositive donors: evidence for indirect effects of primary CMV infection. J Infect Dis 2002; 185:273 - 82; http://dx.doi.org/10.1086/338624; PMID: 11807708
- Fishman JA, Rubin RH. Infection in organ-transplant recipients. N Engl J Med 1998; 338:1741 - 51; http://dx.doi.org/10.1056/NEJM199806113382407; PMID: 9624195
- Sun Q, Hall EC, Huang Y, Chen P, Dibadj K, Murawski M, et al. Pre-transplant myeloid dendritic cell deficiency associated with cytomegalovirus infection and death after kidney transplantation. Transpl Infect Dis 2012; http://dx.doi.org/10.1111/j.1399-3062.2012.00750.x; PMID: 22672201
- Varani S, Frascaroli G, Gibellini D, Potena L, Lazzarotto T, Lemoli RM, et al. Impaired dendritic cell immunophenotype and function in heart transplant patients undergoing active cytomegalovirus infection. Transplantation 2005; 79:219 - 27; http://dx.doi.org/10.1097/01.TP.0000147359.63158.29; PMID: 15665771
- Mezger M, Steffens M, Semmler C, Arlt EM, Zimmer M, Kristjanson GI, et al. Investigation of promoter variations in dendritic cell-specific ICAM3-grabbing non-integrin (DC-SIGN) (CD209) and their relevance for human cytomegalovirus reactivation and disease after allogeneic stem-cell transplantation. Clin Microbiol Infect 2008; 14:228 - 34; http://dx.doi.org/10.1111/j.1469-0691.2007.01902.x; PMID: 18076668
- Fishman JA, Emery V, Freeman R, Pascual M, Rostaing L, Schlitt HJ, et al. Cytomegalovirus in transplantation - challenging the status quo. Clin Transplant 2007; 21:149 - 58; http://dx.doi.org/10.1111/j.1399-0012.2006.00618.x; PMID: 17425738
- Dzabic M, Rahbar A, Yaiw KC, Naghibi M, Religa P, Fellström B, et al. Intragraft cytomegalovirus protein expression is associated with reduced renal allograft survival. Clin Infect Dis 2011; 53:969 - 76; http://dx.doi.org/10.1093/cid/cir619; PMID: 21960711
- Varani S, Landini MP. Cytomegalovirus-induced immunopathology and its clinical consequences. Herpesviridae 2011; 2:6; http://dx.doi.org/10.1186/2042-4280-2-6; PMID: 21473750
- Soderberg C, Sumitran-Karuppan S, Ljungman P, Moller E. CD13-specific autoimmunity in cytomegalovirus-infected immunocompromised patients. Transplantation 1996; 61:594 - 600; http://dx.doi.org/10.1097/00007890-199602270-00014; PMID: 8610387
- Rahbar A, Boström L, Söderberg-Naucler C. Detection of cytotoxic CD13-specific autoantibodies in sera from patients with ulcerative colitis and Crohn’s disease. J Autoimmun 2006; 26:155 - 64; http://dx.doi.org/10.1016/j.jaut.2006.02.003; PMID: 16584867
- Jego G, Palucka AK, Blanck JP, Chalouni C, Pascual V, Banchereau J. Plasmacytoid dendritic cells induce plasma cell differentiation through type I interferon and interleukin 6. Immunity 2003; 19:225 - 34; http://dx.doi.org/10.1016/S1074-7613(03)00208-5; PMID: 12932356
- Carvalho A, Cunha C, Carotti A, Aloisi T, Guarrera O, Di Ianni M, et al. Polymorphisms in Toll-like receptor genes and susceptibility to infections in allogeneic stem cell transplantation. Exp Hematol 2009; 37:1022 - 9; http://dx.doi.org/10.1016/j.exphem.2009.06.004; PMID: 19539691
- Nahum A, Dadi H, Bates A, Roifman CM. The L412F variant of Toll-like receptor 3 (TLR3) is associated with cutaneous candidiasis, increased susceptibility to cytomegalovirus, and autoimmunity. J Allergy Clin Immunol 2011; 127:528 - 31; http://dx.doi.org/10.1016/j.jaci.2010.09.031; PMID: 21093032
- Varani S, Mastroianni A, Frascaroli G, Tammik C, Rahbar A, Christensson M, et al. Generalized Wegener’s granulomatosis in an immunocompetent adult after cytomegalovirus mononucleosis and bacterial urinary tract infection. Arthritis Rheum 2009; 60:1558 - 62; http://dx.doi.org/10.1002/art.24487; PMID: 19404959
- Hsieh AH, Jhou YJ, Liang CT, Chang M, Wang SL. Fragment of tegument protein pp65 of human cytomegalovirus induces autoantibodies in BALB/c mice. Arthritis Res Ther 2011; 13:R162; http://dx.doi.org/10.1186/ar3481; PMID: 21989080
- Chang M, Pan MR, Chen DY, Lan JL. Human cytomegalovirus pp65 lower matrix protein: a humoral immunogen for systemic lupus erythematosus patients and autoantibody accelerator for NZB/W F1 mice. Clin Exp Immunol 2006; 143:167 - 79; http://dx.doi.org/10.1111/j.1365-2249.2005.02974.x; PMID: 16367948
- Baccala R, Hoebe K, Kono DH, Beutler B, Theofilopoulos AN. TLR-dependent and TLR-independent pathways of type I interferon induction in systemic autoimmunity. Nat Med 2007; 13:543 - 51; http://dx.doi.org/10.1038/nm1590; PMID: 17479100
- Kono DH, Haraldsson MK, Lawson BR, Pollard KM, Koh YT, Du X, et al. Endosomal TLR signaling is required for anti-nucleic acid and rheumatoid factor autoantibodies in lupus. Proc Natl Acad Sci USA 2009; 106:12061 - 6; http://dx.doi.org/10.1073/pnas.0905441106; PMID: 19574451
- Xu X, Bergman P, Willows T, Tammik C, Sund M, Hökfelt T, et al. CMV-associated encephalitis and antineuronal autoantibodies - a case report. BMC Neurol 2012; 12:87; http://dx.doi.org/10.1186/1471-2377-12-87; PMID: 22947340
- Zucchini N, Bessou G, Traub S, Robbins SH, Uematsu S, Akira S, et al. Cutting edge: Overlapping functions of TLR7 and TLR9 for innate defense against a herpesvirus infection. J Immunol 2008; 180:5799 - 803; PMID: 18424698
- Rahbar A, Boström L, Lagerstedt U, Magnusson I, Söderberg-Naucler C, Sundqvist VA. Evidence of active cytomegalovirus infection and increased production of IL-6 in tissue specimens obtained from patients with inflammatory bowel diseases. Inflamm Bowel Dis 2003; 9:154 - 61; http://dx.doi.org/10.1097/00054725-200305000-00002; PMID: 12792220
- Baumgart DC, Metzke D, Schmitz J, Scheffold A, Sturm A, Wiedenmann B, et al. Patients with active inflammatory bowel disease lack immature peripheral blood plasmacytoid and myeloid dendritic cells. Gut 2005; 54:228 - 36; http://dx.doi.org/10.1136/gut.2004.040360; PMID: 15647187
- Geist LJ, Monick MM, Stinski MF, Hunninghake GW. The immediate early genes of human cytomegalovirus upregulate tumor necrosis factor-alpha gene expression. J Clin Invest 1994; 93:474 - 8; http://dx.doi.org/10.1172/JCI116995; PMID: 8113386
- Yamazaki S, Steinman RM. Dendritic cells as controllers of antigen-specific Foxp3+ regulatory T cells. J Dermatol Sci 2009; 54:69 - 75; http://dx.doi.org/10.1016/j.jdermsci.2009.02.001; PMID: 19286352
- Ngoma AM, Ikeda K, Hashimoto Y, Mochizuki K, Takahashi H, Sano H, et al. Impaired regulatory T cell reconstitution in patients with acute graft-versus-host disease and cytomegalovirus infection after allogeneic bone marrow transplantation. Int J Hematol 2012; 95:86 - 94; http://dx.doi.org/10.1007/s12185-011-0976-7; PMID: 22160825
- Pastore D, Delia M, Mestice A, Perrone T, Carluccio P, Gaudio F, et al. Recovery of CMV-specific CD8+ T cells and Tregs after allogeneic peripheral blood stem cell transplantation. Biol Blood Marrow Transplant 2011; 17:550 - 7; http://dx.doi.org/10.1016/j.bbmt.2010.04.011; PMID: 20457268
- Nguyen VH, Shashidhar S, Chang DS, Ho L, Kambham N, Bachmann M, et al. The impact of regulatory T cells on T-cell immunity following hematopoietic cell transplantation. Blood 2008; 111:945 - 53; http://dx.doi.org/10.1182/blood-2007-07-103895; PMID: 17916743
- Bruinsma M, Wils EJ, Löwenberg B, Cornelissen JJ, Braakman E. The impact of CD4+Foxp3+ Treg on immunity to murine cytomegalovirus after bone marrow transplantation depends on the peripheral or thymic source of T cell regeneration. Transpl Immunol 2010; 24:9 - 16; http://dx.doi.org/10.1016/j.trim.2010.08.003; PMID: 20801217
- Li YN, Liu XL, Huang F, Zhou H, Huang YJ, Fang F. CD4+CD25+ regulatory T cells suppress the immune responses of mouse embryo fibroblasts to murine cytomegalovirus infection. Immunol Lett 2010; 131:131 - 8; http://dx.doi.org/10.1016/j.imlet.2010.03.011; PMID: 20381532
- Streblow DN, Orloff SL, Nelson JA. Do pathogens accelerate atherosclerosis?. J Nutr 2001; 131:2798S - 804S; PMID: 11584110
- Libby P. Inflammation in atherosclerosis. Nature 2002; 420:868 - 74; http://dx.doi.org/10.1038/nature01323; PMID: 12490960
- Bobryshev YV, Lord RS, Pärsson H. Immunophenotypic analysis of the aortic aneurysm wall suggests that vascular dendritic cells are involved in immune responses. Cardiovasc Surg 1998; 6:240 - 9; http://dx.doi.org/10.1016/S0967-2109(97)00168-3; PMID: 9705095
- Millonig G, Malcom GT, Wick G. Early inflammatory-immunological lesions in juvenile atherosclerosis from the Pathobiological Determinants of Atherosclerosis in Youth (PDAY)-study. Atherosclerosis 2002; 160:441 - 8; http://dx.doi.org/10.1016/S0021-9150(01)00596-2; PMID: 11849669
- Yilmaz A, Lochno M, Traeg F, Cicha I, Reiss C, Stumpf C, et al. Emergence of dendritic cells in rupture-prone regions of vulnerable carotid plaques. Atherosclerosis 2004; 176:101 - 10; http://dx.doi.org/10.1016/j.atherosclerosis.2004.04.027; PMID: 15306181
- Pryshchep O, Ma-Krupa W, Younge BR, Goronzy JJ, Weyand CM. Vessel-specific Toll-like receptor profiles in human medium and large arteries. Circulation 2008; 118:1276 - 84; http://dx.doi.org/10.1161/CIRCULATIONAHA.108.789172; PMID: 18765390
- Niessner A, Sato K, Chaikof EL, Colmegna I, Goronzy JJ, Weyand CM. Pathogen-sensing plasmacytoid dendritic cells stimulate cytotoxic T-cell function in the atherosclerotic plaque through interferon-alpha. Circulation 2006; 114:2482 - 9; http://dx.doi.org/10.1161/CIRCULATIONAHA.106.642801; PMID: 17116765
- Melnick JL, Hu C, Burek J, Adam E, DeBakey ME. Cytomegalovirus DNA in arterial walls of patients with atherosclerosis. J Med Virol 1994; 42:170 - 4; http://dx.doi.org/10.1002/jmv.1890420213; PMID: 8158112
- Melnick JL, Petrie BL, Dreesman GR, Burek J, McCollum CH, DeBakey ME. Cytomegalovirus antigen within human arterial smooth muscle cells. Lancet 1983; 2:644 - 7; http://dx.doi.org/10.1016/S0140-6736(83)92529-1; PMID: 6136795
- Gredmark S, Jonasson L, Van Gosliga D, Ernerudh J, Söderberg-Nauclér C. Active cytomegalovirus replication in patients with coronary disease. Scand Cardiovasc J 2007; 41:230 - 4; http://dx.doi.org/10.1080/14017430701383755; PMID: 17680510
- Roberts ET, Haan MN, Dowd JB, Aiello AE. Cytomegalovirus antibody levels, inflammation, and mortality among elderly Latinos over 9 years of follow-up. Am J Epidemiol 2010; 172:363 - 71; http://dx.doi.org/10.1093/aje/kwq177; PMID: 20660122
- Simanek AM, Dowd JB, Pawelec G, Melzer D, Dutta A, Aiello AE. Seropositivity to cytomegalovirus, inflammation, all-cause and cardiovascular disease-related mortality in the United States. PLoS One 2011; 6:e16103; http://dx.doi.org/10.1371/journal.pone.0016103; PMID: 21379581
- Prösch S, Staak K, Stein J, Liebenthal C, Stamminger T, Volk HD, et al. Stimulation of the human cytomegalovirus IE enhancer/promoter in HL-60 cells by TNFalpha is mediated via induction of NF-kappaB. Virology 1995; 208:197 - 206; http://dx.doi.org/10.1006/viro.1995.1143; PMID: 11831701
- van Leeuwen EM, Remmerswaal EB, Vossen MT, Rowshani AT, Wertheim-van Dillen PM, van Lier RA, et al. Emergence of a CD4+CD28- granzyme B+, cytomegalovirus-specific T cell subset after recovery of primary cytomegalovirus infection. J Immunol 2004; 173:1834 - 41; PMID: 15265915
- Liuzzo G, Goronzy JJ, Yang H, Kopecky SL, Holmes DR, Frye RL, et al. Monoclonal T-cell proliferation and plaque instability in acute coronary syndromes. Circulation 2000; 101:2883 - 8; http://dx.doi.org/10.1161/01.CIR.101.25.2883; PMID: 10869258
- Looney RJ, Falsey A, Campbell D, Torres A, Kolassa J, Brower C, et al. Role of cytomegalovirus in the T cell changes seen in elderly individuals. Clin Immunol 1999; 90:213 - 9; http://dx.doi.org/10.1006/clim.1998.4638; PMID: 10080833
- Hazzan M, Labalette M, Noel C, Lelievre G, Dessaint JP. Recall response to cytomegalovirus in allograft recipients: mobilization of CD57+, CD28+ cells before expansion of CD57+, CD28- cells within the CD8+ T lymphocyte compartment. Transplantation 1997; 63:693 - 8; http://dx.doi.org/10.1097/00007890-199703150-00014; PMID: 9075840
- Fasth AE, Dastmalchi M, Rahbar A, Salomonsson S, Pandya JM, Lindroos E, et al. T cell infiltrates in the muscles of patients with dermatomyositis and polymyositis are dominated by CD28null T cells. J Immunol 2009; 183:4792 - 9; http://dx.doi.org/10.4049/jimmunol.0803688; PMID: 19752224
- Tovar-Salazar A, Patterson-Bartlett J, Jesser R, Weinberg A. Regulatory function of cytomegalovirus-specific CD4+CD27-CD28- T cells. Virology 2010; 398:158 - 67; http://dx.doi.org/10.1016/j.virol.2009.11.038; PMID: 20034645
- Appay V, Dunbar PR, Callan M, Klenerman P, Gillespie GM, Papagno L, et al. Memory CD8+ T cells vary in differentiation phenotype in different persistent virus infections. Nat Med 2002; 8:379 - 85; http://dx.doi.org/10.1038/nm0402-379; PMID: 11927944
- Fletcher JM, Vukmanovic-Stejic M, Dunne PJ, Birch KE, Cook JE, Jackson SE, et al. Cytomegalovirus-specific CD4+ T cells in healthy carriers are continuously driven to replicative exhaustion. J Immunol 2005; 175:8218 - 25; PMID: 16339561