Abstract
The reassortment of genetic segments from different host species and from different subtypes of influenza A viruses occurs frequently, which may generate new strains causing flu epidemic or pandemic. However, the underlined mechanisms of reassortment were less addressed from the viewpoint of protein variations. Recently, we used the amino-acid pair predictability as an indicator to convert eight types of influenza A virus proteins into predictable portion of amino-acid pairs, and then applied the models I and II ANOVA to estimate their differences in terms of subtypes and host species. In order to get a full picture, 2729 and 1063 non-structural 1 and 2 proteins of influenza A viruses were analyzed in this study. The results are consistent with those obtained from hemagglutinin, neuraminidase, nucleoprotein, polymerase acidic protein, polymerase basic proteins 1 and 2, and matrix proteins 1 and 2, indicating that inter-species/subtypes variations are smaller than intra-species/subtype ones. Our findings provide statistical evidence that can partially explains why cross-subtype mutation and cross-species infection easily occur during co-infecting of different strains.
Introduction
It is well known that unpredictable mutations of influenza viruses can trigger seasonal influenza epidemics and occasional pandemics, which have being threatened humans and other species worldwide. Among various mutation patterns, the reassortment of genetic segments from different host species and from different subtypes is more serious because such a mutation can form a new strain that may become a pathogen no longer sensitive to anti-infection drugs and induces the outbreak of influenza,Citation1 such as the last outbreak of influenza A/H1H1 pandemic that was due to a new strain resulting from the reassortant of swine, avian, and human influenza viruses.Citation2-Citation4
In general, influenza viruses are classified as different subtypes according to their two surface proteins and as different species according to their affected host, thus viral differences between subtypes and between species are remarkable, because they prevent the formation of cross-subtype mutation, which may come from multiple mutations leading to a virus to change from one subtype to another subtype, and cross-host infection. For example, H5N1 influenza A virus was considered to have higher virulence than other subtypes of influenza A viruses.Citation5,Citation6 Equine influenza viruses hardly affect humans because of substantial incompatibility among their gene products.Citation7 However, frequent emergence of the reassortment of genetic segments from different subtypes and from different species challenges the barriers between subtypes and between species of influenza viruses,Citation8 and many studies have been addressed this issue.Citation9-Citation12 If genetic segments of influenza viruses would be quite different between species/subtypes, a formation of reassortment from different species and subtypes would be easily prevented. Indeed, this reassortment implies smaller difference in genetic segments between species/subtypes. An intriguing question raised here is how to estimate the difference of viral proteins from different species and subtypes.
In statistics, the one-way ANOVA is widely used to determine difference of certain characteristic among different groups. However, this method works on numbers rather than alphabets, which represent amino acids in proteins. In order to overcome this obstacle, we have developed dynamic protein features, which can numerically present proteins and then can be used in various models.Citation13-Citation16 Recently, we applied our method to compare inter- and intra- species/subtype variations in eight proteins from influenza A viruses.Citation17-Citation24 The results showed that the inter-species/subtype variations were generally smaller than intra-species/subtype ones, indicating that reassortment is a tendency in influenza A viruses, which would form new strains leading cross-species infection and cross-subtype mutation.
The segmented genome of influenza A virus encodes 10 or 11 proteins depending on the strain. So far, we have yet to analyze the non-structural (NS) proteins that have multiple functions during viral infection.Citation25,Citation26 They can affect the host immune response through many virus and host-cell processes.Citation27,Citation28 Amino acid sequence analysis of the NS1 proteins revealed that some nonsynonymous substitutions play a critical role in shaping the genetic diversity.Citation29 Animal experiment showed that a single residue change in the highly conserved SH2bm within NS1 protein (Y89F) can strongly reduce virulence.Citation30 The combination of all these functions makes the NS1 protein a very potent inhibitor of immunity and allows influenza virus to efficiently escape the immune surveillance and to establish infection in the host.Citation31 As a nuclear export protein, the NS2 protein can change RNA levels by specific alteration of the viral transcription and replication machinery.Citation32,Citation33 Thus, non-structural proteins have the potential application benefits for drug target and vaccination against influenza viruses.Citation34-Citation37
In comparison with other eight analyzed proteins from influenza A viruses, the aim of this study is to estimate the inter-species/subtype and intra-species/subtype variations of non-structural proteins to see their role on reassortment and get whole insights into this issue from different proteins of influenza A viruses.
Results
and show the comparisons of NS1 and NS2 proteins, where the upper, middle and lower panels represent the results obtained from HA and NA subtypes, and species, respectively. For example, of various species there are 467 NS1 proteins in H1 subtype with the predictable portion of 39.1 ± 3.2% (the first bar in the upper panel of ). Statistical differences are found among subtypes and species in both NS1 and NS2 proteins tested by ANOVA (P < 0.001).
Figure 1. Comparison of NS1 difference among HA subtypes, among NA subtypes and among species. The data were presented as mean ± SD. The one-way ANOVA was used to compare the difference among subtypes/species.
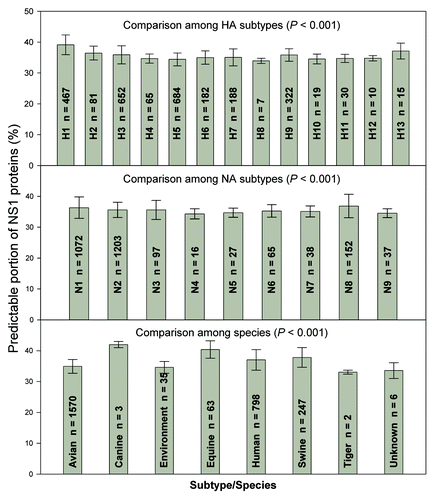
Figure 2. Comparison of NS2 difference among HA subtypes, among NA subtypes and among species. The data were presented as mean ± SD. The one-way ANOVA was used to compare the difference among subtypes/species.
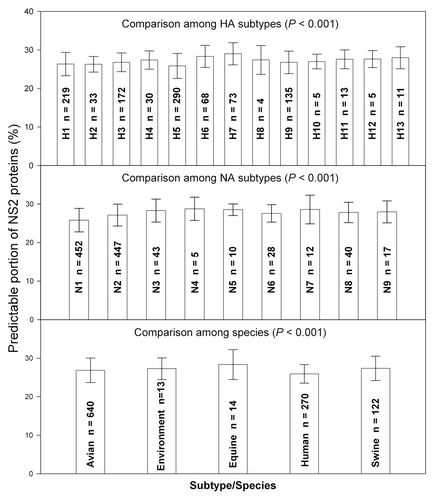
elucidates the results obtained from all paired comparisons. In this figure, the upper right and lower left triangles show the data from NS1 and NS2 proteins, respectively. Among 13 HA subtypes in the upper panel, difference and no difference are found in 43 and 35 pairs in NS1 proteins, and in 16 and 62 pairs in NS2 proteins; Among 9 NA subtypes in the middle panel, difference and no difference are found in 16 and 20 pairs in NS1 proteins, and in 9 and 27 pairs in NS2 proteins; In the lower panel, difference and no difference are found in 21 and 7 pairs among 8 species in NS1 proteins, while difference and no difference are found in 3 and 7 pairs among 5 species in NS2 proteins. Comparing both proteins, the number of pairs with statistical difference is larger in NS1 proteins than that of NS2 ones, for instance, NS1 proteins reveal P < 0.001 and P = 0.021 (Chi-square test) in HA subtypes and in species.
Figure 3. All paired comparisons in different HA subtypes (A), NA subtypes (B), and species (C). The upper right and lower left triangles show the data from NS1 and NS2 proteins, respectively. D and No indicated the difference with and without statistical significance (the Holm–Sidak comparison test).
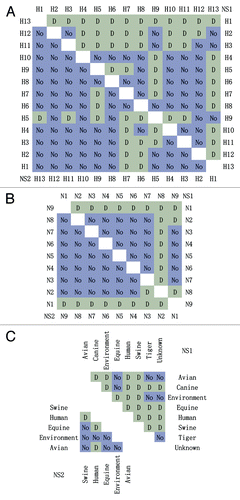
displays difference between 3 main host species (avian, human, and swine) among 9 subtypes in NS1 proteins and 6 subtypes in NS2 ones, which further reveal that NS2 proteins have a smaller species difference than NS1 ones. As can be seen, among 19 pairs for comparison there are 12 pairs with difference and 7 ones without difference in NS1 proteins (the upper panel), whereas among 14 pairs in NS2 proteins (the lower panel) there are only 4 pairs with difference but 10 ones without difference.
Figure 4. Comparison of species difference among different subtypes. The data were presented as mean ± SD. The one-way ANOVA and Holm–Sidak comparison test were used to compare the difference among and between species. # and * indicated the statistical significance from corresponding avian and human at P < 0.05 level.
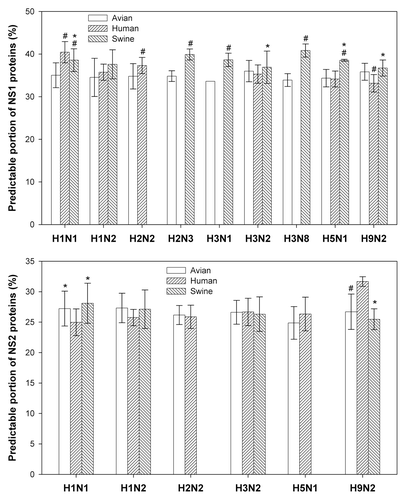
The above results suggest larger intra-subtype/species variations in comparison with inter-subtypes/species ones. This issue can be analyzed using the standard ANOVA table and the result is listed in , which demonstrates a tendency that inter-subtypes/species variation is general smaller than intra-subtype/species one, which appears in nine out of 13 objectives in NS1 proteins and in all nine objectives in NS2 proteins. Furthermore, inter- and intra-subtype/species variations can be presented by percentage instead of the sum of squares as our previous studies.Citation17-Citation24 displays such results calculated from NS1 and NS2 proteins in different subtypes and species, in comparing with other eight influenza A virus proteins. This figure highlights the fact that inter-subtypes/species variation is generally smaller than intra-subtype/species one in all of influenza A virus proteins. shows variation percentages of inter- and intra-species calculated from different subtypes, where dominant intra-species variations can be further found in H3N2, H5N1, and H9N2 subtypes of 10 viral proteins.
Table 1. Standard ANOVA table regarding subtype and species of NS1 and NS2 from influenza A viruses
Figure 5. Between species/subtype variations (black) and within species/subtype variations (gray) in ten proteins from influenza A viruses. HA, hemagglutinin; NA, neuraminidase; NP, nucleoprotein; PA, polymerase acidic protein; PB1 and PB2, polymerase basic protein 1 and 2; M1 and M2, matrix protein 1 and 2; NS1 and NS2, nonstructural protein 1 and 2.

Figure 6. Between subtypes variations (black) and within subtype variations (gray) in ten proteins from influenza A viruses. HA, hemagglutinin; NA, neuraminidase; NP, nucleoprotein; PA, polymerase acidic protein; PB1 and PB2, polymerase basic protein 1 and 2; M1 and M2, matrix protein 1 and 2; NS1 and NS2, nonstructural. protein 1 and 2.
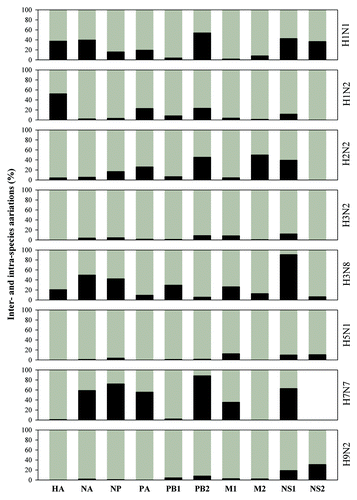
Discussion
Because influenza A viruses are negative-sense and have eight single-stranded RNA segments,Citation10 which is the foundation for genetic reassortment that provides genomic and phenotypic changes through genetic materials directly exchanging from different subtypes and species;Citation11,Citation12,Citation38 however, the effects of reassortment are still difficult to analyze. Our efforts pave the way to estimate the issue that why the genetic reassortment is so easily occur in influenza A viruses by means of statistical analysis. Hereby we analyzed the inter- and intra-subtype/species variations in NS1 and NS2 proteins using both models I and II ANOVA, and the results are consistence with our previous studies and show that small inter-subtype/species variations can be found in all of 10 proteins of influenza A viruses, which can partially explains why cross-subtype mutation and cross-species infection easily occur during co-infecting of different strains.
By means of specific deletions, truncations, and elongations, influenza viruses can synthesize their proteins that are variable in length, which relates to virulence and host adaptation.Citation39 Also, the genetic reassortment provides the way for fast changing viral host range and virulence through adaptive evolution, where influenza viruses reveal an extraordinary ability to diversify.Citation40 Phylogenetic analysis shows that the degree of variation is low within the alleles of non-structural protein 1. Bogoyavlenskiy et al. studied different combinations from 7 HA subtypes and 5 NA subtypes, and they found that the maximum of amino acid divergence in clade was 5% and 4% in allele A and B viruses.Citation41 Our studies provide statistical evidence that ten types of influenza A virus proteins have small inter-subtypes variations, indicating that the barrier between subtypes is general weaker to prevent cross-subtype mutation.
For transmission and maintenance in certain species, there may be a competitive advantage in some genetic segments over other combinations, although within a host multiple reassortments can take place.Citation42 Our results demonstrate that the intra-species variations are quite larger than the inter-species ones in H3N2, H5N1, and H9N2 subtypes, which suggests that the barrier between species is very weak in these subtypes. No differences are found between many paired comparisons, for example, human vs. swine in H1N2 subtype; human vs. avian in H1N2, H3N2, and H5N1 subtypes; and swine vs. avian in H1N2, H3N2, and H9N2 subtypes in NS1 proteins, proving theoretical reason for easy cross-species infection. Thus, reassortments potentially transmit the genetic diversity from the donor host to the recipient one, which may make influenza A viruses adapt to new host species, although it is originally perceived that transmission bottlenecks is narrow.Citation43
Host range variants or antigenic escape may be induced during the course of single infections, where potentially advantageous mutations can orrue.Citation44 Our findings can shed some lights on the strategy for designing anti-viral vaccines and drugs. The vaccines and drugs would easily suffer inefficacy if they are designed against single subtype/species because variations are generally small between subtypes and species. A large-scale analysis revealed that universally conserved residues can serve as candidates for protein-protein interactions, basing on the amino acid sequences of 2620 NS1 proteins.Citation45 Therefore the heterogeneity of anti-viral vaccines and drugs can benefit for pandemic preparedness.Citation46,Citation47
Many studies from various research laboratories around the world have indicated that mathematical analysis, computational modeling, and introducing novel physical concept to solve important problems in biology and medicine, such as protein structural class prediction,Citation48,Citation49 modeling 3D structures of targeted proteins for drug design,Citation50-Citation53 diffusion-controlled reaction simulation,Citation54,Citation55 cellular responding kinetics,Citation56 bio-macromolecular internal collective motion simulation,Citation57-Citation59 identification of proteases and their types,Citation60 membrane protein type prediction,Citation61 viral protein misfolding,Citation62,Citation63 and protein–protein interaction,Citation64,Citation65 can timely provide very useful information and insights for both basic and clinical research, and hence are widely welcome by science community. The present study is related to the fundamental problems in system biology, network biology, and structural biology of proteins. The relationship between these systems will be of use for the global research.
Materials and Methods
Data
In order to let this study be comparable with previous studies, 7826 full-length NS1 proteins of influenza A viruses sampled from 1918 to 2008 and 6635 NS2 sampled from 1931 to 2009 were obtained from the Influenza Virus Resources.Citation66 After excluded identical sequences,Citation67 2729 NS1 and 1063 NS2 proteins were actually used in this study.
Quantification of NS proteins
As mentioned in the Introduction, the one-way ANOVA deals with decimal data rather than alphabets that represent amino acids in proteins, thus all NS proteins must be converted into numbers, and the amino-acid pair predictability has been used to do so.Citation13-Citation24,Citation68-Citation73 Taking the ABB86874 NS2 protein (strain A/swine/Ontario/57561/03[H1N1]) as an example, it has 121 amino acids, which construct 120 adjacent amino-acid pairs. This NS2 protein has 15 leucines, “L”, and the appearance of amino-acid pair LL should be twice according to the permutation (15/121 × 14/120 × 120 = 1.74). There are 2 pairs of LL found in the NS2 protein, so the amino-acid pair LL is predictable. By contrast, there are 7 phenylalanines “F” and 14 glutamic acids “E” in this protein, and the appearance of amino-acid pair FE should be once (7/121 × 14/120 × 120 = 0.81). However, it appears 3 times in reality, so the amino-acid pair FE is unpredictable. In this way, all amino-acid pairs in this NS2 protein can be classified as predictable and unpredictable, and their portions are 31.11% and 68.89%, respectively. Thus, ABB86874 NS2 protein can be represented by either 31.11% or 68.89%. Another swine NS2 protein (accession number ABB86944) has only one amino acid different from ABB86874 NS2 protein at position 6, but its predictable and unpredictable portions are 30.77% and 69.23% so the ABB86944 NS2 protein can be represented by either 30.77% or 69.23%, which is different from ABB86874 NS2 protein.
Actually, such quantification is based on random principle, i.e., the issue that an amino acid joins into an amino-acid pair is probabilistically independent event. As predictable amino-acid pair requires the least time and energy for construction, and thus nature would intuitively build a protein with a maximal predictable portion, while unpredictable portion implies probabilistically unstable, which is more likely subject to mutations.Citation14-Citation16
Comparing variation between and within species/subtype
After converting all NS proteins into numerical data, their predictable portions were grouped according to their subtypes and species because influenza A viruses are characterized by their surface proteins and by the species affected host.Citation74 The one-way ANOVA (model I ANOVA) followed by the Holm–Sidak comparison test was used to compare the difference among subtypes/species and the Holm–Sidak comparison test was used to compare the difference between subtypes/species. The single classification model II ANOVA with unequal sample sizesCitation75,Citation76 was used to determine the inter- and intra-subtype/species variations. Analysis of variance requires samples to be normally distributed, sample size in our cases is large enough to grantee the normal distribution because data distribution is generally considered to be normal when data set is large than 50 as indicated by Statistica software. It turns out that the difference detected by ANOVA in terms of inter- and intra-subtype/species variations is how difference in protein sequences among subtypes/species, while the bigger the difference is, the more different in protein sequence is, the more difficult for mutations to lead cross-subtype mutation and cross-species infection is. Thus, the difference detected by ANOVA can be viewed as barriers between species and subtypes. The SigmaStat software was used to conduct all comparisons. P < 0.05 was considered statistically significant.
Disclosure of Potential Conflicts of Interest
No potential conflicts of interest were disclosed.
Acknowledgments
This study was partly supported by Guangxi Science Foundation (12237022, 13-051-08, 13-051-50, and 2013GXNSDA019007) and by BaGui Scholars Program Foundation.
References
- Yang JR, Lin YC, Huang YP, Su CH, Lo J, Ho YL, Yao CY, Hsu LC, Wu HS, Liu MT. Reassortment and mutations associated with emergence and spread of oseltamivir-resistant seasonal influenza A/H1N1 viruses in 2005-2009. PLoS One 2011; 6:e18177; http://dx.doi.org/10.1371/journal.pone.0018177; PMID: 21483816
- Christman MC, Kedwaii A, Xu J, Donis RO, Lu G. Pandemic (H1N1) 2009 virus revisited: an evolutionary retrospective. Infect Genet Evol 2011; 11:803 - 11; http://dx.doi.org/10.1016/j.meegid.2011.02.021; PMID: 21382522
- Ducatez MF, Hause B, Stigger-Rosser E, Darnell D, Corzo C, Juleen K, Simonson R, Brockwell-Staats C, Rubrum A, Wang D, et al. Multiple reassortment between pandemic (H1N1) 2009 and endemic influenza viruses in pigs, United States. Emerg Infect Dis 2011; 17:1624 - 9; PMID: 21892996
- Bokhari SH, Pomeroy LW, Janies DA. Reassortment Networks and the evolution of pandemic H1N1 swine-origin influenza. IEEE/ACM Trans Comput Biol Bioinform 2012; 9:214 - 27; http://dx.doi.org/10.1109/TCBB.2011.95; PMID: 22076498
- Michaelis M, Doerr HW, Cinatl J Jr.. Of chickens and men: avian influenza in humans. Curr Mol Med 2009; 9:131 - 51; http://dx.doi.org/10.2174/156652409787581565; PMID: 19275623
- Amendola A, Ranghiero A, Zanetti A, Pariani E. Is avian influenza virus A(H5N1) a real threat to human health?. J Prev Med Hyg 2011; 52:107 - 10; PMID: 22010536
- Li C, Hatta M, Watanabe S, Neumann G, Kawaoka Y. Compatibility among polymerase subunit proteins is a restricting factor in reassortment between equine H7N7 and human H3N2 influenza viruses. J Virol 2008; 82:11880 - 8; http://dx.doi.org/10.1128/JVI.01445-08; PMID: 18815312
- Mehle A, Dugan VG, Taubenberger JK, Doudna JA. Reassortment and mutation of the avian influenza virus polymerase PA subunit overcome species barriers. J Virol 2012; 86:1750 - 7; http://dx.doi.org/10.1128/JVI.06203-11; PMID: 22090127
- Noda T, Sugita Y, Aoyama K, Hirase A, Kawakami E, Miyazawa A, Sagara H, Kawaoka Y. Three-dimensional analysis of ribonucleoprotein complexes in influenza A virus. Nat Commun 2012; 3:639; http://dx.doi.org/10.1038/ncomms1647; PMID: 22273677
- Sarkar M, Chanda S, Chakrabarti S, Mazumdar J, Ganguly A, Chadha MS, Mishra AC, Chawla-Sarkar M. Surveillance in Eastern India (2007-2009) revealed reassortment event involving NS and PB1-F2 gene segments among co-circulating influenza A subtypes. Virol J 2012; 9:3; http://dx.doi.org/10.1186/1743-422X-9-3; PMID: 22217077
- Yurovsky A, Moret BM. FluReF, an automated flu virus reassortment finder based on phylogenetic trees. BMC Genomics 2011; 12:Suppl 2 S3; http://dx.doi.org/10.1186/1471-2164-12-S2-S3; PMID: 21989112
- Greenbaum BD, Li OT, Poon LL, Levine AJ, Rabadan R. Viral reassortment as an information exchange between viral segments. Proc Natl Acad Sci U S A 2012; 109:3341 - 6; http://dx.doi.org/10.1073/pnas.1113300109; PMID: 22331898
- Wu G, Yan S. Randomness in the primary structure of protein: methods and implications. Mol Biol Today 2002; 3:55 - 69
- Wu G, Yan SM. Mutation trend of hemagglutinin of influenza A virus: a review from a computational mutation viewpoint. Acta Pharmacol Sin 2006; 27:513 - 26; http://dx.doi.org/10.1111/j.1745-7254.2006.00329.x; PMID: 16626505
- Wu G, Yan S. Lecture Notes on Computational Mutation. New York: Nova Science Publishers, 2008:1-130.
- Yan S, Wu G. Creation and application of computational mutation. J Guangxi Acad Sci 2010; 17:145 - 50
- Yan SM, Wu G. Rationale for cross-species infection and cross-subtype mutation in hemagglutinins from influenza A virus. Interdiscip Sci 2009; 1:303 - 7; http://dx.doi.org/10.1007/s12539-009-0068-1; PMID: 20640809
- Yan S, Wu G. Determination of inter- and intra-subtype variations in polymerase acidic protein from influenza A virus using amino-acid pair predictability. J Biomed Sci Eng 2009; 2:273 - 9; http://dx.doi.org/10.4236/jbise.2009.24041
- Yan S, Wu G. Evidence obtained from ANOVA to reason cross-species infection and cross-subtype mutation in neuraminidases of influenza A viruses. Transbound Emerg Dis 2010; 57:254 - 61; PMID: 20545912
- Yan S, Wu G. Evidence for cross-species infections and cross-subtype mutations in influenza a matrix proteins. Viral Immunol 2010; 23:105 - 11; http://dx.doi.org/10.1089/vim.2009.0080; PMID: 20121408
- Yan SM, Zuo WP, Zhu QX, Huang YY, Pan LX, Wu G. Statistical evidence for cross-species infection and cross-subtype mutation in matrix protein 2 family of influenza A virus. J Guangxi Acad Sci 2010; 26:45 - 51
- Yan SM, Wu G. Reasons for cross-species infection and cross-subtype reassortment in nucleoproteins from influenza A virus. Proceedings of the 5th International Conference on Bioinformatics and Biomedical Engineering 2011; IEEE Xplore 978-1-4244-5089-3/11.
- Yan S, Wu G. Possible reason for cross-species and cross-subtype reassortment in polymerase basic protein 2 from influenza A virus. Protein Pept Lett 2011; 18:434 - 9; http://dx.doi.org/10.2174/092986611794927956; PMID: 21171947
- Yan S, Wu G. Small variations between species/subtypes attributed to reassortment evidenced from polymerase basic protein 1 with other seven proteins from influenza a virus. Transbound Emerg Dis 2013; 60:110 - 9; http://dx.doi.org/10.1111/j.1865-1682.2012.01323.x; PMID: 22462488
- Hale BG, Randall RE, Ortín J, Jackson D. The multifunctional NS1 protein of influenza A viruses. J Gen Virol 2008; 89:2359 - 76; http://dx.doi.org/10.1099/vir.0.2008/004606-0; PMID: 18796704
- Salahuddin P, Khan AU. Structural and functional analysis of NS1 and NS2 proteins of H1N1 subtype. Genomics Proteomics Bioinformatics 2010; 8:190 - 9; http://dx.doi.org/10.1016/S1672-0229(10)60021-6; PMID: 20970747
- Bavagnoli L, Dundon WG, Garbelli A, Zecchin B, Milani A, Parakkal G, Baldanti F, Paolucci S, Volmer R, Tu Y, et al. The PDZ-ligand and Src-homology type 3 domains of epidemic avian influenza virus NS1 protein modulate human Src kinase activity during viral infection. PLoS One 2011; 6:e27789; http://dx.doi.org/10.1371/journal.pone.0027789; PMID: 22110760
- Munir M, Zohari S, Belák S, Berg M. Double-stranded RNA-induced activation of activating protein-1 promoter is differentially regulated by the non-structural protein 1 of avian influenza A viruses. Viral Immunol 2012; 25:79 - 85; PMID: 22239235
- Pavesi A. Pattern of nucleotide substitution in the overlapping nonstructural genes of influenza A virus and implication for the genetic diversity of the H5N1 subtype. Gene 2007; 402:28 - 34; http://dx.doi.org/10.1016/j.gene.2007.07.013; PMID: 17825505
- Hrincius ER, Hennecke AK, Gensler L, Nordhoff C, Anhlan D, Vogel P, McCullers JA, Ludwig S, Ehrhardt C. A single point mutation (Y89F) within the non-structural protein 1 of influenza A viruses limits epithelial cell tropism and virulence in mice. Am J Pathol 2012; 180:2361 - 74; http://dx.doi.org/10.1016/j.ajpath.2012.02.029; PMID: 22525464
- Fernandez-Sesma A. The influenza virus NS1 protein: inhibitor of innate and adaptive immunity. Infect Disord Drug Targets 2007; 7:336 - 43; http://dx.doi.org/10.2174/187152607783018754; PMID: 18220965
- Robb NC, Smith M, Vreede FT, Fodor E. NS2/NEP protein regulates transcription and replication of the influenza virus RNA genome. J Gen Virol 2009; 90:1398 - 407; http://dx.doi.org/10.1099/vir.0.009639-0; PMID: 19264657
- Shimizu T, Takizawa N, Watanabe K, Nagata K, Kobayashi N. Crucial role of the influenza virus NS2 (NEP) C-terminal domain in M1 binding and nuclear export of vRNP. FEBS Lett 2011; 585:41 - 6; http://dx.doi.org/10.1016/j.febslet.2010.11.017; PMID: 21081124
- Schickli JH, Flandorfer A, Nakaya T, Martinez-Sobrido L, García-Sastre A, Palese P. Plasmid-only rescue of influenza A virus vaccine candidates. Philos Trans R Soc Lond B Biol Sci 2001; 356:1965 - 73; http://dx.doi.org/10.1098/rstb.2001.0979; PMID: 11779399
- De Clercq E, Neyts J. Avian influenza A (H5N1) infection: targets and strategies for chemotherapeutic intervention. Trends Pharmacol Sci 2007; 28:280 - 5; http://dx.doi.org/10.1016/j.tips.2007.04.005; PMID: 17481739
- Lee JH, Oh JY, Pascua PN, Kim EG, Choi YK, Kim HK. Impairment of the Staufen1-NS1 interaction reduces influenza viral replication. Biochem Biophys Res Commun 2011; 414:153 - 8; http://dx.doi.org/10.1016/j.bbrc.2011.09.042; PMID: 21945618
- Akarsu H, Iwatsuki-Horimoto K, Noda T, Kawakami E, Katsura H, Baudin F, Horimoto T, Kawaoka Y. Structure-based design of NS2 mutants for attenuated influenza A virus vaccines. Virus Res 2011; 155:240 - 8; http://dx.doi.org/10.1016/j.virusres.2010.10.014; PMID: 20970464
- Van Kerkhove MD, Mumford E, Mounts AW, Bresee J, Ly S, Bridges CB, Otte J. Highly pathogenic avian influenza (H5N1): pathways of exposure at the animal-human interface, a systematic review. PLoS One 2011; 6:e14582; http://dx.doi.org/10.1371/journal.pone.0014582; PMID: 21283678
- Dundon WG. Variability among the neuraminidase, non-structural 1 and PB1-F2 proteins in the influenza A virus genome. Virus Genes 2012; 44:363 - 73; http://dx.doi.org/10.1007/s11262-012-0714-0; PMID: 22261818
- Pepin KM, Lass S, Pulliam JR, Read AF, Lloyd-Smith JO. Identifying genetic markers of adaptation for surveillance of viral host jumps. Nat Rev Microbiol 2010; 8:802 - 13; http://dx.doi.org/10.1038/nrmicro2440; PMID: 20938453
- Bogoyavlenskiy A, Berezin V, Prilipov A, Korotetskiy I, Zaitseva I, Kydyrmanov A, Karamedin K, Ishmukhametova N, Asanova S, Sayatov M, et al. Phylogenetic analysis of the non-structural (NS) gene of influenza A viruses isolated in Kazakhstan in 2002-2009. Virol Sin 2011; 26:376 - 85; http://dx.doi.org/10.1007/s12250-011-3208-7; PMID: 22160937
- Ma W, Lager KM, Lekcharoensuk P, Ulery ES, Janke BH, Solórzano A, Webby RJ, García-Sastre A, Richt JA. Viral reassortment and transmission after co-infection of pigs with classical H1N1 and triple-reassortant H3N2 swine influenza viruses. J Gen Virol 2010; 91:2314 - 21; http://dx.doi.org/10.1099/vir.0.021402-0; PMID: 20484565
- Hoelzer K, Murcia PR, Baillie GJ, Wood JL, Metzger SM, Osterrieder N, Dubovi EJ, Holmes EC, Parrish CR. Intrahost evolutionary dynamics of canine influenza virus in naive and partially immune dogs. J Virol 2010; 84:5329 - 35; http://dx.doi.org/10.1128/JVI.02469-09; PMID: 20219907
- Murcia PR, Baillie GJ, Daly J, Elton D, Jervis C, Mumford JA, Newton R, Parrish CR, Hoelzer K, Dougan G, et al. Intra- and interhost evolutionary dynamics of equine influenza virus. J Virol 2010; 84:6943 - 54; http://dx.doi.org/10.1128/JVI.00112-10; PMID: 20444896
- Darapaneni V, Prabhaker VK, Kukol A. Large-scale analysis of influenza A virus sequences reveals potential drug target sites of non-structural proteins. J Gen Virol 2009; 90:2124 - 33; http://dx.doi.org/10.1099/vir.0.011270-0; PMID: 19420157
- Kreijtz JH, Fouchier RA, Rimmelzwaan GF. Immune responses to influenza virus infection. Virus Res 2011; 162:19 - 30; http://dx.doi.org/10.1016/j.virusres.2011.09.022; PMID: 21963677
- Qiu C, Huang Y, Wang Q, Tian D, Zhang W, Hu Y, Yuan Z, Zhang X, Xu J. Boosting heterosubtypic neutralization antibodies in recipients of 2009 pandemic H1N1 influenza vaccine. Clin Infect Dis 2012; 54:17 - 24; http://dx.doi.org/10.1093/cid/cir753; PMID: 22052887
- Zhou GP, Assa-Munt N. Some insights into protein structural class prediction. Proteins: Struct, Func. Genet 2001; 44:57 - 9
- Chou KC, Zhang CT. Prediction of protein structural classes. Crit Rev Biochem Mol Biol 1995; 30:275 - 349; http://dx.doi.org/10.3109/10409239509083488; PMID: 7587280
- Zhou GP, Troy FA 2nd. NMR studies on how the binding complex of polyisoprenol recognition sequence peptides and polyisoprenols can modulate membrane structure. Curr Protein Pept Sci 2005; 6:399 - 411; http://dx.doi.org/10.2174/138920305774329377; PMID: 16248792
- Chou KC. Structural bioinformatics and its impact to biomedical science. Curr Med Chem 2004; 11:2105 - 34; http://dx.doi.org/10.2174/0929867043364667; PMID: 15279552
- Sharma AK, Zhou GP, Kupferman J, Surks HK, Christensen EN, Chou JJ, Mendelsohn ME, Rigby AC. Probing the interaction between the coiled coil leucine zipper of cGMP-dependent protein kinase Ialpha and the C terminus of the myosin binding subunit of the myosin light chain phosphatase. J Biol Chem 2008; 283:32860 - 9; http://dx.doi.org/10.1074/jbc.M804916200; PMID: 18782776
- Zhou GP, Surks HK, Schnell JR, Chou JJ, Michael E, Mendelsohn ME, Rigby AC. The three-dimensional structure of the cGMP-dependent protein kinase I-α leucine zipper domain and its interaction with the myosin binding subunit. Blood 2004; 104:963a
- Chou KC, Zhou GP. Role of the protein outside active site on the diffusion-controlled reaction of enzyme. J Am Chem Soc 1982; 104:1409 - 13; http://dx.doi.org/10.1021/ja00369a043
- Zhou GP, Li TT, Chou KC. The flexibility during the juxtaposition of reacting groups and the upper limits of enzyme reactions. Biophys Chem 1981; 14:277 - 81; http://dx.doi.org/10.1016/0301-4622(81)85028-4; PMID: 7326350
- Qi JP, Shao SH, Li DD, Zhou GP. A dynamic model for the p53 stress response networks under ion radiation. Amino Acids 2007; 33:75 - 83; http://dx.doi.org/10.1007/s00726-006-0454-3; PMID: 17072789
- Zhou GP. Biological functions of soliton and extra electron motion in DNA structure. Phys Scr 1989; 40:698 - 701; http://dx.doi.org/10.1088/0031-8949/40/5/021
- Chou KC. Low-frequency collective motion in biomacromolecules and its biological functions. Biophys Chem 1988; 30:3 - 48; http://dx.doi.org/10.1016/0301-4622(88)85002-6; PMID: 3046672
- Chou KC. The biological functions of low-frequency vibrations (phonons). VI. A possible dynamic mechanism of allosteric transition in antibody molecules. Biopolymers 1987; 26:285 - 95; http://dx.doi.org/10.1002/bip.360260209; PMID: 3828475
- Zhou GP, Cai YD. Predicting protease types by hybridizing gene ontology and pseudo amino acid composition Proteins Struct, Func. Bioinformat 2006; 63:681 - 4
- Cai YD, Zhou GP, Chou KC. Support vector machines for predicting membrane protein types by using functional domain composition. Biophys J 2003; 84:3257 - 63; http://dx.doi.org/10.1016/S0006-3495(03)70050-2; PMID: 12719255
- Zhou GP, Huang RB. The pH-triggered conversion of the PrP(c) to PrP(sc.). Curr Top Med Chem 2013; 13:1152 - 63; http://dx.doi.org/10.2174/15680266113139990003; PMID: 23647538
- Bjorndahl TC, Zhou GP, Liu XH, Perez-Pineiro R, Semenchenko V, Saleem F, Acharya S, Bujold A, Sobsey CA, Wishart DS. Detailed biophysical characterization of the acid-induced PrP(c) to PrP(β) conversion process. Biochemistry 2011; 50:1162 - 73; http://dx.doi.org/10.1021/bi101435c; PMID: 21189021
- Zhou GP. The disposition of the LZCC protein residues in wenxiang diagram provides new insights into the protein-protein interaction mechanism. J Theor Biol 2011; 284:142 - 8; http://dx.doi.org/10.1016/j.jtbi.2011.06.006; PMID: 21718705
- Zhou GP. The structural determinations of the leucine zipper coiled-coil domains of the cGMP-dependent protein kinase Iα and its interaction with the myosin binding subunit of the myosin light chains phosphase. Protein Pept Lett 2011; 18:966 - 78; http://dx.doi.org/10.2174/0929866511107010966; PMID: 21592084
- National Center for Biotechnology Information. Influenza virus resources. 2013; http://www.ncbi.nlm.nih.gov/genomes/FLU/Database/multiple.cgi.
- Furuse Y, Suzuki A, Kamigaki T, Oshitani H. Evolution of the M gene of the influenza A virus in different host species: large-scale sequence analysis. Virol J 2009; 6:67 - 79; http://dx.doi.org/10.1186/1743-422X-6-67; PMID: 19476650
- Wu G, Yan S. Fate of influenza A virus proteins. Protein Pept Lett 2006; 13:377 - 84; http://dx.doi.org/10.2174/092986606775974474; PMID: 16712514
- Wu G, Yan S. Prediction of mutations engineered by randomness in H5N1 neuraminidases from influenza A virus. Amino Acids 2008; 34:81 - 90; http://dx.doi.org/10.1007/s00726-007-0579-z; PMID: 17721674
- Wu G, Yan S. Prediction of mutations in H3N2 hemagglutinins of influenza A virus from North America based on different datasets. Protein Pept Lett 2008; 15:144 - 52; http://dx.doi.org/10.2174/092986608783489571; PMID: 18289106
- Wu G, Yan S. Three sampling strategies to predict mutations in H5N1 hemagglutitins from influenza A virus. Protein Pept Lett 2008; 15:731 - 8; http://dx.doi.org/10.2174/092986608785133735; PMID: 18782070
- Yan SM, Wu G. Prediction of mutation position, mutated amino acid and timing in hemagglutinins from North America H1 influenza A virus. J Biomed Sci Eng 2009; 2:117 - 22; http://dx.doi.org/10.4236/jbise.2009.22021
- Yan S, Wu G. Prediction of mutation positions in H5N1 neuraminidases from influenza A virus by means of neural network. Ann Biomed Eng 2010; 38:984 - 92; http://dx.doi.org/10.1007/s10439-010-9907-7; PMID: 20336836
- Spackman E. A brief introduction to the avian influenza virus. Methods Mol Biol 2008; 436:1 - 6; PMID: 18370034
- Sokal RR, Rohlf FJ. Biometry: The Principles and Practices of Statistics in Biological Research. 3nd ed. New York: W. H. Freeman, 1995:203-17.
- Wu G, Baraldo M, Furlanut M. Inter-patient and intra-patient variations in the baseline tapping test in patients with Parkinson’s disease. Acta Neurol Belg 1999; 99:182 - 4; PMID: 10544726