Abstract
In contrast to commensal Escherichia coli, extraintestinal pathogenic E. coli (ExPEC) strains possess an array of virulence-associated genes. We sought to establish the feasibility of using the invertebrate Galleria mellonella (greater wax moth) for assessing ExPEC virulence and to investigate the correlation between genotypic determinants of virulence and in vivo pathogenicity. We observed a correlation between the number of virulence genes and larval survival, such that ExPEC isolates with higher virulence scores killed larvae significantly faster than isolates with lower virulence scores. By correlating genotypic and phenotypic virulence, we provide preliminary validation of this model for future studies investigating ExPEC virulence.
Introduction
Escherichia coli is a major human pathogen, and infections caused by E. coli are associated with significant morbidity and mortality.Citation1 Human-associated strains of E. coli can be broadly classified into three main pathotypes: (1) commensal E. coli strains, which exist as part of the normal gastrointestinal flora, (2) intestinal pathogenic E. coli strains, such as enterohemorrhagic E. coli (EHEC) and enteropathogenic E. coli (EPEC), and (3) extraintestinal pathogenic E. coli (ExPEC).Citation2 Although most commonly associated with uncomplicated urinary tract infections, ExPEC strains cause a range of serious infections, including meningitis, pneumonia, and bacteremia.Citation1
In contrast to commensal E. coli, ExPEC strains possess an array of virulence-associated genes including those encoding adhesins, invasins, toxins, siderophores, and polysaccharide capsules.Citation3,Citation4 These putative virulence factors, often encoded on mobile pathogenicity islands, enable ExPEC strains to successfully colonize, and in some instances invade, their human hosts. However, despite considerable characterization of the molecular epidemiology and virulence gene repertoire of ExPEC strains,Citation5-Citation7 studies assessing in vivo correlates of molecular virulence potential are less common. Such studies are important, not only for assessing previously identified virulence traits, but also for identifying new candidate virulence factors and potential therapeutic targets.
Recently, larvae of the greater wax moth Galleria mellonella have been increasingly utilized as an alternative model host to study microbial pathogenesis.Citation8 Previous studies have demonstrated the utility of this simple invertebrate model system in studying the virulence of several major human extraintestinal pathogens, including Candida albicans,Citation9 Acinetobacter baumannii,Citation10 Staphylococcus aureus,Citation11 and Pseudomonas aeruginosa.Citation12 Importantly, for some pathogens, virulence in G. mellonella has been shown to correlate with both molecular virulence characteristics and pathogenicity in mammalian model systems.Citation13 To date however, no studies have assessed the efficacy of the G. mellonella model for studies of ExPEC pathogenicity, although one previous study demonstrated the utility of this model for studying EPEC virulence.Citation14 Accordingly, we sought to: (1) to establish the feasibility and reproducibility of using G. mellonella as a host for assessing ExPEC virulence and (2) to investigate the comparative virulence of a collection of molecularly characterized ExPEC strains, with particular regard to correlation of molecularly inferred virulence and experimental in vivo pathogenicity.
Results and Discussion
Infection characteristics of E. coli strains in G. mellonella
To investigate the feasibility of using G. mellonella to study E. coli pathogenicity, we evaluated larval survival using increasing inocula of an E. coli reference strain (ATCC 25922). We observed a dose-dependent response on larval survival, such that increasing concentrations of bacterial cells killed larvae significantly faster (). At doses below 5 × 106 CFU, less than 30% of larvae were killed after 4 d. In contrast, at doses above 5 × 106 CFU, over 80% of larvae were killed within 24 h (P < 0.001). A dose of ~1 × 106 CFU consistently killed 30–40% of larvae by 72 h (), and was therefore considered to provide enough discriminatory power to determine comparative differences in ExPEC virulence in subsequent experiments.
In order to determine whether viable E. coli cells were required for larval killing, we inoculated larvae with heat-killed E. coli ATCC 25922 or the heat-killed clinical isolate UOAE8433. When followed for 125 h post-injection, no deaths occurred among larvae inoculated with either PBS or heat-killed E. coli (data not shown). We also assessed whether larval killing may be due to E. coli secretory products rather than live bacterial cells. However, when larvae were inoculated with filter-sterilized bacterial supernatants taken from stationary-phase growth cultures, no larval killing was observed after 125 h (data not shown). In keeping with other studies of bacterial pathogenesis in this model, these results indicate that viable E. coli cells are required for killing of G. mellonella.Citation14 Future work should attempt to elucidate the specific mechanism(s) of larval death as a result of E. coli infection.
We also determined the effect of administration of an antimicrobial agent (ciprofloxacin) on survival of G. mellonella infected with clinical isolates previously determined to be either susceptible or resistant to ciprofloxacin in vitro. We found that among larvae inoculated with a ciprofloxacin-susceptible isolate (UOAE8502), the ciprofloxacin-treated group had significantly improved survival at 4 d compared with the non-treated group (90% vs. 35%; P < 0.001) (). In contrast, when larvae were inoculated with a ciprofloxacin-resistant isolate (UOAE8433), ciprofloxacin did not improve larval survival, with no significant difference between the treated and non-treated groups (P = 0.97). Therefore, our data document a correlation of in vitro antimicrobial resistance with in vivo response in this model system, which suggest that G. mellonella may be a useful model for future studies assessing antimicrobial efficacy against ExPEC strains prior to mammalian experimentation. For example, screening of novel agents in G. mellonella could conceivably rapidly identify those that have poor in vivo efficacy, thereby reducing the extent of subsequent mammalian testing required.
Figure 2. Effect of ciprofloxacin (CIP) treatment against CIP-susceptible (CIP-S) isolate UOAE8502 and CIP-resistant (CIP-R) isolate UOAE8433. The difference in larval killing between the CIP-treated and control groups was statistically significant for isolate UOAE8502 (CIP-S) (P < 0.001), but not for isolate UOAE8433 (CIP-R).
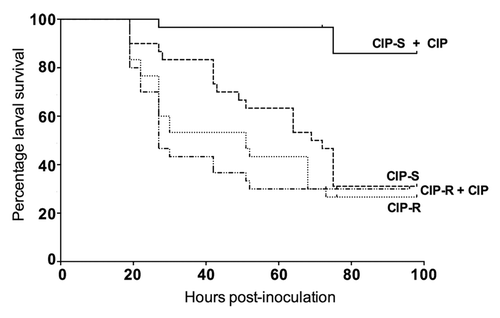
In vivo correlates of genotypic virulence determinants in G. mellonella
For invertebrate hosts such as G. mellonella to be useful extrapolative models for studies of in vivo pathogenicity, a correlation between genotypic determinants of virulence (as identified through human epidemiological studies and animal experimental studies) and phenotypic virulence (i.e., larval death) should exist. We therefore sought to use the G. mellonella model to assess the comparative virulence of a collection of clinical E. coli isolates that had previously been characterized for a broad range of virulence-associated genes, then to compare larval killing with virulence genotype.Citation15 Clinical and molecular characteristics of study strains are shown in and . The aggregate virulence gene scores of the clinical isolates ranged from 4 to 23.
Table 1. Sources and aggregate virulence scores of extra-instestinal Escherichia coli strains used in the Galleria mellonella assay
Table 2. Genotypic virulence determinants of Escherichia coli strains used in Galleria mellonella survival experiments as determined by multiplex PCR
After 125 h, no deaths were observed in larvae inoculated with PBS, or the reference strains MG1655 or DH10b (data not shown). However, we observed a significant association between the aggregate virulence gene score and larval killing at 125 h (R2 = 0.54, P = 0.02), such that ExPEC isolates with higher scores killed larvae significantly faster and in greater numbers than isolates with lower scores (). For example, ExPEC isolates (with their aggregate virulence scores listed parenthetically) UOAE6384 (13), UOAE8433 (19), and UOAE8502 (23) killed >65% of larvae at 125 h, whereas isolates UOAE3153 (4), UOAE1656 (7), and UOAE4027 (10) killed <10% of larvae (). This is in keeping with the association previously observed between molecularly inferred virulence score and lethality in a murine model.Citation16
Figure 3. Correlation between molecularly inferred virulence and in vivo pathogenicity in the Galleria mellonella model system with eight Escherichia coli bloodstream isolates. Experiments were performed on three independent occasions. Curves for each isolate are labeled according to aggregate virulence gene score (V), with actual doses used [expressed as x 106 CFU] as follows: UOAE3153 (V4) [1.50/1.08/1.29]; UOAE4027, (V10a) [1.42/1.00/1.63]; UOAE6392 (V10b) [1.00/1.79/1.13]; UOAE0212 (V11a) [1.33/1.33/1.79]; UOAE6384 (V13) [1.33/1.54/1.63]; UOAE8433 (V19) [1.63/1.93/1.29]; UOAE8502 (V23) [1.42/1.13/1.25]; UOAE0886 (V11b) [1.54/1.67/1.50].
![Figure 3. Correlation between molecularly inferred virulence and in vivo pathogenicity in the Galleria mellonella model system with eight Escherichia coli bloodstream isolates. Experiments were performed on three independent occasions. Curves for each isolate are labeled according to aggregate virulence gene score (V), with actual doses used [expressed as x 106 CFU] as follows: UOAE3153 (V4) [1.50/1.08/1.29]; UOAE4027, (V10a) [1.42/1.00/1.63]; UOAE6392 (V10b) [1.00/1.79/1.13]; UOAE0212 (V11a) [1.33/1.33/1.79]; UOAE6384 (V13) [1.33/1.54/1.63]; UOAE8433 (V19) [1.63/1.93/1.29]; UOAE8502 (V23) [1.42/1.13/1.25]; UOAE0886 (V11b) [1.54/1.67/1.50].](/cms/asset/b0222ec7-09bc-4106-ba8f-039195cfe9a9/kvir_a_10927912_f0003.gif)
The lack of larval deaths after inoculation with isolates UOAE3153 (4), UOAE1656 (7), and UOAE4027 (10a) suggests that the virulence genes possessed by these isolates are either not expressed during infection of G. mellonella larvae or, if expressed, do not significantly diminish larval survival. Further research investigating ExPEC virulence in this model could include mutagenesis or knockout studies assessing putative ExPEC virulence factors. Interestingly, strain UOAE0886, which had an aggregate virulence gene score of 11, demonstrated the fastest larval killing. It is therefore possible that virulence genes other than those assessed by the multiplex PCR assay we used play an important role in larval killing. In addition, the level and timing of gene expression, and presence of minor genotypic polymorphisms may also significantly influence the effect of certain genes on pathogenicity.
Conclusions
To our knowledge, this represents the first study to assess the utility of the wax moth G. mellonella as a model host for studying ExPEC pathogenicity and virulence. We found that larval killing was: (1) dependent on the challenge E. coli strain being alive, (2) different among different ExPEC strains, (3) prevented by administration of an appropriate antimicrobial, and (4) significantly associated with aggregate virulence gene score. By correlating genotypic and phenotypic virulence in this way, our proof-of-principle study provides preliminary validation of this simple, non-mammalian model for further investigation of the virulence of this major human pathogen. Future studies should correlate these findings with observed virulence in mammalian systems.
Methods
Strains and molecular characterization
The E. coli isolates used in this study are shown in . Clinical isolates were from patients with E. coli bloodstream infections; these isolates were obtained from a previous study performed in the Department of Clinical Microbiology, Auckland City Hospital, New Zealand.Citation17 Identification and antimicrobial susceptibility testing of these isolates was done as part of this prior study.Citation17 Two K12-derived E. coli laboratory reference strains, MG1655 and DH10b,Citation18 were used for comparison in larval survival experiments. In addition, an E. coli reference strain commonly used in antibiotic susceptibility testing (ATCC 25922) was used to establish the appropriate inocula for subsequent experiments and to determine whether viable E. coli cells were required for larval killing. Prior to use, all strains were cultured on Luria–Bertani (LB) agar or in LB broth and incubated aerobically at 37 °C. Growth kinetic curves were performed with all strains prior to use in the G. mellonella assay.
Molecular characterization of E. coli isolates was performed using previously described methodsCitation15,Citation19 (). Briefly, a multiplex PCR was used to detect 50 virulence-associated genes and their allelic variants,Citation15,Citation19 and an aggregate virulence gene score was assigned to each isolate as previously described,Citation15 depending on the number of virulence-associated genes detected, after adjusting for multiple detection of the following operons: pap (P fimbriae), sfa/foc (S and F1C fimbriae), and kps (group 2 capsule).
Galleria mellonella infection model
G. mellonella larvae in the fifth instar larval phase (Biosuppliers Ltd) were stored in the dark at room temperature and were used within seven days of receipt. All larvae were between 100 and 200 mg and displayed minimal melanization prior to use. For each experiment, larvae were infected by inoculating 20 uL of the prepared inoculum into the hemocoel through the last left proleg using an insulin syringe. Before inoculation, bacterial cells were washed in phosphate buffered saline (PBS) and re-suspended to give the appropriate inocula, which were confirmed by retrospective plating onto LB agar. All experimental groups contained 10 randomly selected larvae and each experiment was repeated at least three times using larvae from different batches. In all experiments, PBS was used as an inoculation control. Post-inoculation, larvae were housed without food in 24-well plates and incubated at 37 °C. Dead larvae were enumerated daily. Larvae were considered dead when they failed to respond to touch.
To determine whether viable E. coli cells were required for larval killing, heat-killed E. coli strains (ATCC 25922 and one of the clinical strains: UOAE8433 []) were used to infect larvae. Briefly, cells were heated at 80 °C for 30 min and plated onto LB agar to ensure that no viable cells were remaining. In addition, larvae were also inoculated with filter-sterilized supernatant obtained from these two strains in stationary phase growth cultures to assess the possible effect of bacterial secretory products on larval survival.
For antimicrobial efficacy testing, groups of larvae were inoculated with a lethal inoculum of E. coli clinical isolates that had been previously determined to be either susceptible (UOAE8502) or resistant to ciprofloxacin (UOAE8433) in vitro. Within 30 min of inoculation, ciprofloxacin was administered by injection into a different proleg. The PBS control group was injected twice, to control for the dual administration of both inoculum and antibiotic. Ciprofloxacin was administrated at 10 mg kg−1 of body weight, based on human dosing criteria.
Statistical analysis
Survival curves were generated using the Kaplan–Meier method, while the degree of association between the virulence gene score and larval survival was determined using the Pearson correlation coefficient. All statistical analysis was performed using GraphPad Prism (Version 6.0). A P value of < 0.05 was considered to indicate a statistically significant difference.
Disclosure of Potential Conflicts of Interests
J.R.J. has received grants or contracts from Merck, Rochester Medical, and Syntiron, and has patent applications regarding molecular tests for E. coli clonal groups. All other authors have no conflicts to report.
Acknowledgments
We thank the staff of the Clinical Microbiology laboratory at Auckland District Health Board, New Zealand for performing antimicrobial susceptibility tests. D.A.W. is supported by a Clinical Research Training Fellowship from the Health Research Council (HRC) of New Zealand and S.W. by an HRC Sir Charles Hercus Fellowship (09/099). This material is based in part upon work supported by Office of Research and Development, Medical Research Service, Department of Veterans Affairs (J.R.J.).
References
- Russo TA, Johnson JR. Medical and economic impact of extraintestinal infections due to Escherichia coli: focus on an increasingly important endemic problem. Microbes Infect 2003; 5:449 - 56; http://dx.doi.org/10.1016/S1286-4579(03)00049-2; PMID: 12738001
- Kaper JB, Nataro JP, Mobley HL. Pathogenic Escherichia coli.. Nat Rev Microbiol 2004; 2:123 - 40; http://dx.doi.org/10.1038/nrmicro818; PMID: 15040260
- Sannes MR, Kuskowski MA, Owens K, Gajewski A, Johnson JR. Virulence factor profiles and phylogenetic background of Escherichia coli isolates from veterans with bacteremia and uninfected control subjects. J Infect Dis 2004; 190:2121 - 8; http://dx.doi.org/10.1086/425984; PMID: 15551210
- Zhang L, Foxman B, Marrs C. Both urinary and rectal Escherichia coli isolates are dominated by strains of phylogenetic group B2. J Clin Microbiol 2002; 40:3951 - 5; http://dx.doi.org/10.1128/JCM.40.11.3951-3955.2002; PMID: 12409357
- Köhler CD, Dobrindt U. What defines extraintestinal pathogenic Escherichia coli?. Int J Med Microbiol 2011; 301:642 - 7; http://dx.doi.org/10.1016/j.ijmm.2011.09.006; PMID: 21982038
- Johnson JR, Russo TA. Molecular epidemiology of extraintestinal pathogenic (uropathogenic) Escherichia coli.. Int J Med Microbiol 2005; 295:383 - 404; http://dx.doi.org/10.1016/j.ijmm.2005.07.005; PMID: 16238015
- Johnson JR, Johnston B, Clabots C, Kuskowski MA, Pendyala S, Debroy C, Nowicki B, Rice J. Escherichia coli sequence type ST131 as an emerging fluoroquinolone-resistant uropathogen among renal transplant recipients. Antimicrob Agents Chemother 2010; 54:546 - 50; http://dx.doi.org/10.1128/AAC.01089-09; PMID: 19917759
- Glavis-Bloom J, Muhammed M, Mylonakis E. Of model hosts and man: using Caenorhabditis elegans, Drosophila melanogaster and Galleria mellonella as model hosts for infectious disease research. Adv Exp Med Biol 2012; 710:11 - 7; http://dx.doi.org/10.1007/978-1-4419-5638-5_2; PMID: 22127881
- Fuchs BB, O’Brien E, Khoury JB, Mylonakis E. Methods for using Galleria mellonella as a model host to study fungal pathogenesis. Virulence 2010; 1:475 - 82; http://dx.doi.org/10.4161/viru.1.6.12985; PMID: 21178491
- Peleg AY, Jara S, Monga D, Eliopoulos GM, Moellering RC Jr., Mylonakis E. Galleria mellonella as a model system to study Acinetobacter baumannii pathogenesis and therapeutics. Antimicrob Agents Chemother 2009; 53:2605 - 9; http://dx.doi.org/10.1128/AAC.01533-08; PMID: 19332683
- Peleg AY, Monga D, Pillai S, Mylonakis E, Moellering RC Jr., Eliopoulos GM. Reduced susceptibility to vancomycin influences pathogenicity in Staphylococcus aureus infection. J Infect Dis 2009; 199:532 - 6; http://dx.doi.org/10.1086/596511; PMID: 19125671
- Inglis RF, Gardner A, Cornelis P, Buckling A. Spite and virulence in the bacterium Pseudomonas aeruginosa.. Proc Natl Acad Sci U S A 2009; 106:5703 - 7; http://dx.doi.org/10.1073/pnas.0810850106; PMID: 19321425
- Chua KY, Seemann T, Harrison PF, Monagle S, Korman TM, Johnson PD, Coombs GW, Howden BO, Davies JK, Howden BP, et al. The dominant Australian community-acquired methicillin-resistant Staphylococcus aureus clone ST93-IV [2B] is highly virulent and genetically distinct. PLoS One 2011; 6:e25887; http://dx.doi.org/10.1371/journal.pone.0025887; PMID: 21991381
- Leuko S, Raivio TL. Mutations that impact the enteropathogenic Escherichia coli Cpx envelope stress response attenuate virulence in Galleria mellonella.. Infect Immun 2012; 80:3077 - 85; http://dx.doi.org/10.1128/IAI.00081-12; PMID: 22710873
- Williamson DA, Freeman JT, Porter S, Roberts S, Wiles S, Paterson DL, Johnson JR. Clinical and molecular correlates of virulence in Escherichia coli causing bloodstream infection following transrectal ultrasound-guided (TRUS) prostate biopsy. J Antimicrob Chemother 2013; 68:2898 - 906; http://dx.doi.org/10.1093/jac/dkt276; PMID: 23833184
- Johnson JR, Porter SB, Zhanel G, Kuskowski MA, Denamur E. Virulence of Escherichia coli clinical isolates in a murine sepsis model in relation to sequence type ST131 status, fluoroquinolone resistance, and virulence genotype. Infect Immun 2012; 80:1554 - 62; http://dx.doi.org/10.1128/IAI.06388-11; PMID: 22311928
- Williamson DA, Roberts SA, Paterson DL, Sidjabat H, Silvey A, Masters J, Rice M, Freeman JT. Escherichia coli bloodstream infection after transrectal ultrasound-guided prostate biopsy: implications of fluoroquinolone-resistant sequence type 131 as a major causative pathogen. Clin Infect Dis 2012; 54:1406 - 12; http://dx.doi.org/10.1093/cid/cis194; PMID: 22419681
- Blattner FR, Plunkett G 3rd, Bloch CA, Perna NT, Burland V, Riley M, Collado-Vides J, Glasner JD, Rode CK, Mayhew GF, et al. The complete genome sequence of Escherichia coli K-12. Science 1997; 277:1453 - 62; http://dx.doi.org/10.1126/science.277.5331.1453; PMID: 9278503
- Johnson JR, Menard M, Johnston B, Kuskowski MA, Nichol K, Zhanel GG. Epidemic clonal groups of Escherichia coli as a cause of antimicrobial-resistant urinary tract infections in Canada, 2002 to 2004. Antimicrob Agents Chemother 2009; 53:2733 - 9; http://dx.doi.org/10.1128/AAC.00297-09; PMID: 19398649