Abstract
Non-invasive imaging techniques in microbial disease models have delivered valuable insights in the intimate pathogen-host interplay during infection. Here we describe evaluation and validation of a transgenic bioluminescence reporter strain of the human-pathogenic mold Aspergillus fumigatus, one of the main fungal pathogens affecting immunocompromised individuals. Expression and surface display of the Gaussia princeps luciferase allowed sensitive and rapid detection of luminescence emitted from this strain after substrate addition, with photon fluxes strongly correlating to the amounts of fungal conidia or germlings. The reporter strain allowed spatio-temporal monitoring of infection in a cutaneous model of aspergillosis, where neutropenic mice maintained the fungal burden while immunocompetent ones were able to clear it entirely. Most importantly, antifungal therapy could be followed in this type of disease model making use of the bioluminescent A. fumigatus strain. In conclusion, combining sensitivity of the Gaussia luciferase with a surface display expression system in the fungal host allows longitudinal infection studies on cutaneous forms of aspergillosis, providing perspective on drug screening approaches at high-throughput.
Introduction
Fungal infections have gained increasing relevance for public health as well as in distinct clinical settings.Citation1,Citation2 Among the predominant fungal pathogens, the ubiquitous mold Aspergillus fumigatus has become of interest, based on the fact that the number of susceptible, immunocompromised patients has steadily risen over the past two decades.Citation3-Citation5 Hematological malignancies and prolonged neutropenia are the leading risk factors associated with Aspergillus infections, which primarily occur via the pulmonary route to possibly result in invasive or disseminated, systemic forms. Invasive aspergillosis (IA) is associated with a high mortality as it is often diagnosed late with therapeutic options being limited. Besides this prevalent type of the disease, several less frequent ones have been described, among them cutaneous forms of aspergillosis, which make up 5 to 10% of IA cases.Citation6,Citation7 Primary cutaneous infections with A. fumigatus conidia may occur after skin injuries or extensive burns; also, neonatal infections by contaminated dressings have been described.Citation8-Citation11 Additionally, systemic forms of IA may result in cutaneous localization of fungal lesions.
Investigations on factors relevant for aspergillosis pathogenesis or drug testing experiments strictly rely on suitable disease model, most commonly established in susceptible small animals like immunocompromised guinea pigs or mice.Citation12 For pulmonary aspergillosis, murine models have been validated extensively to cover distinct preconditioning aspects (e.g., neutropenia or corticosteriod treatment). A model for cutaneous aspergillosis, however, was just recently introduced using nude (nu/nu) mice rendered leukopenic by cyclophosphamide treatment.Citation13 Soft tissue infections with A. fumigatus were performed by subcutaneous injection of a conidial suspension in the animals’ thigh. From there, a skin lesion expanded, the area of which correlated with fungal burdens as estimated by galactomannan contents. In this setting, the attenuated virulence of distinct A. fumigatus mutants that had been characterized to be hypovirulent in pulmonary infections, as well as a reduction in tissue lesion size during antifungal treatment could be monitored, validating this type of disease model to a reasonable extent.
Monitoring fungal infections in real time and repeatedly in an animal enables longitudinal host-pathogen interaction studies. Non-invasive imaging allows the collection of spatial and temporal data during an infection with lesser animals required. Recent improvements in live imaging techniques had been achieved by the help of luminescent reporter enzymes and the use of highly sensitive charged-coupled device cameras.Citation14-Citation17 Among the former, luciferases from the firefly Photinus pyralis, the sea pansy Renilla reniliformis or the copepod Gaussia princeps are well established, each displaying distinct characteristics. With respect to fungal infections, latest progress has been made by establishment of recombinant, bioluminescent strains for the commensal Candida albicans as well as for A. fumigatus.Citation18 In the latter, a cytoplasmatically expressed firefly luciferase gene product allowed bioluminescence detection after addition of the substrate luciferin. This reporter strain proved useful in imaging early stages of pulmonary infection following different immunosuppressive regimens and also for antifungal drug efficiency testing.Citation19,Citation20 A C. albicans strain cells expressing the more luminescent G. princeps luciferase (GLuc) on its cell surface was extensively characterized for its imaging properties in several disease models that varied with respect to the site of infection.Citation21 Among the currently established bioluminescence reporters, the GLuc enzyme has emerged as most efficiently catalyzing light emission in an ATP-independent manner from its substrate coelenterazine, permitting highly sensitive imaging that is less dependent on the environmental context.Citation22 For aspergillosis and antifungal treatment of the disease, however, this particular luciferase reporter has not been evaluated to date.
In order to assess capacities of the luminescent G. princeps enzyme for monitoring infections by A. fumigatus, we here describe generation and characterization of a recombinant strain expressing a synthetic gene expression construct to result in surface display of the GLuc reporter. This strain allows sensitive and longitudinal infection studies by bioluminescence imaging, as validated in a cutaneous murine infection model of aspergillosis.
Results
Expression and surface display of Gaussia princeps luciferase in A. fumigatus
Pathogen detection via bioluminescent imaging requires proper and high-level expression of the according luciferase enzyme. Furthermore, suitable conditions for substrate turnover have to be taken into account, making the validation of a bioluminescent reporter strain a challenging task. In order to establish a recombinant A. fumigatus strain that would express the copepod GLuc luciferase, we assembled a synthetic construct (pSK478) harboring several functional elements for expression, secretion, and membrane anchoring. From this plasmid, transcription of the gluc gene is driven by a truncated version of the constitutively strong A. nidulans gpdA promoter, which had been validated before to be actively transcribed under in vitro and in vivo conditions.Citation19 In order to achieve targeting of the translation product into the secretory pathway, two secretion signals were fused in frame to the gluc coding sequence: the one from the A. oryzae tglA gene plus the Rhyzopus oryzae N28 region, which derives from a lipase-encoding gene.Citation23 To express the luminescent enzyme in a surface-exposed manner and allow facilitated substrate turnover, its coding sequence was furthermore fused to a putative glucosylphosphatidylinositol (GPI)-anchored protein, which serves as a carrier for cellular localization. There, the A. fumigatus ortholog of the A. oryzae cell wall protein MP1 was chosen, as this had already been successfully exploited in Aspergillus surface display studies.Citation24 The A. fumigatus expression vector pSK379 served as backbone for this modular gene to allow selection for integration into the A. fumigatus genome,Citation25,Citation26 finally resulting in the plasmid construct pSK481 ().
Figure 1. Surface display of the Gaussia princeps luciferase on Aspergillus fumigatus. (A) Schematic presentation of the integrative plasmid to express a synthetic, codon-optimized version of the gluc gene in A. fumigatus and to target its product to the cell surface by anchoring it to the membrane protein MP1. Functional elements of the expression cassette are the constitutively transcribed gpdA promoter (pgpdA) from A. nidulans and the his2A terminator region (his2At) from A. fumigatus. For secretion, the GLuc coding sequence (gluc) is preceded by a signal sequence (ss) from the A. oryzae tglA gene and the Rhyzopus oryzae N28 region. In-frame fusion via a (GA)5 linker region to the coding region of the GPI-anchored MP1 protein from A. fumigatus results in surface display of the bioluminescent reporter. (B) X-ray film autoradiography after addition of the GLuc substrate coelenterazine to mycelia of different transformants carrying the expression construct. Besides the wild-type recipient strain ATCC 46645, isolates carrying one, two and four copies, respectively, of the integrative plasmid pSK481 are displayed. (C) Light intensities emitted from GLuc reporter strains expressing varying doses of the bioluminescent reporter based on the indicated copy numbers. Photographs were taken before (upper panel) and after (lower panel) coelenterazine addition with a digital camera in the light and dark, respectively.
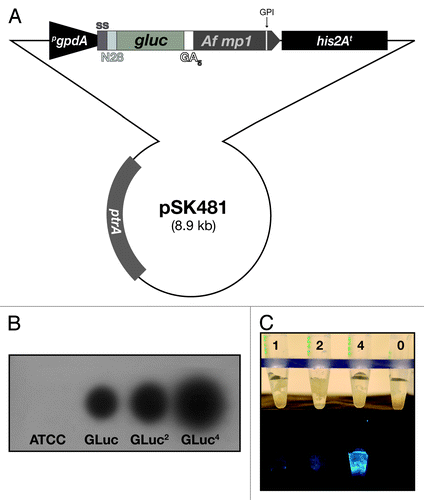
After transformation of the A. fumigatus wild-type strain ATCC 46645 with pSK481, several isolates recovered from selective plates were analyzed for bioluminescence in the presence of the GLuc substrate coelenterazine. First, conidial suspensions were allowed to germinate in black transparent-well plates to detect signals on X-ray film after substrate addition. From the pool of primary transformants, several isolates displaying varying intensities of bioluminescence were propagated further to become analyzed in more detail. Finally, three recombinant strains were picked that carried one, two and four copies of the expression module integrated in the fungal genome as assessed by qPCR on genomic DNA (data not shown). Autoradiographies from exposed X-ray films as well as light intensities emitted from these strains correlated well with increasing copy numbers (). Besides a pronounced bioluminescence upon addition of coelenterazine, no obvious phenotypes were associated with expression of the surface-exposed luciferase. For further studies, focus was set on the most bioluminescent strain AfS75.
In order to confirm exposed localization of the bioluminescent enzyme on the surface of the A. fumigatus host, indirect immunofluorescence studies using a monoclonal antibody raised against the G. luciferase protein were performed (). Whereas the recipient strain ATCC 46645 did not display any prominent fluorescence in this assay, a distinct surface-associated staining could be observed for the Gaussia luciferase-expressing strain. This was corroborated by partial trypsin digests on mycelia of AfS75, which resulted in a drastic decrease of measurable bioluminescence in a time-dependent manner () without affecting viabilities (not shown).
Figure 2. Exposure of the bioluminescent reporter GLuc on the surface of A. fumigatus AfS75. (A) Indirect immunofluorescence with a monoclonal antibody against the G. princeps luc gene product displays association of the fluorescent signal with the hyphal surface. (B) Partial tryptic digest of AfS75 germlings results in a decrease of bioluminescence emitted from this strain. Shown are photon fluxes derived from 1 × 108 germlings that had been digested with trypsin for the indicated periods of time.
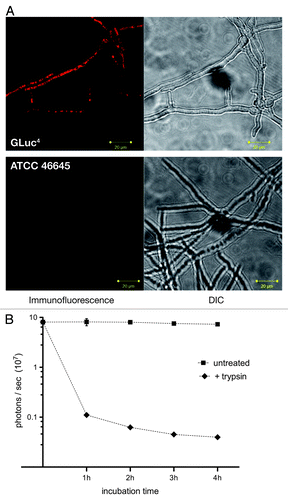
The reporter strain was further characterized with respect to the kinetic properties of its luminescence. After substrate addition, a rapid decline in photon emission was observed (), in agreement with the established flash kinetic of the G. princeps luciferase enzyme.Citation27 Accordingly, an estimated half-life of the bioluminescent signal of 38 sec could be deduced from this nonlinear decay. To determine the minimal number of fungal cells that would result in detectable bioluminescence signals, serial dilutions of conidia from AfS75 were prepared and allowed to swell, germinate, and eventually form hyphal filaments over an 8 h time period. Addition of the substrate coelenterazine generated a photon flux that was still detectable from 103 germlings or 104 conidia and that increased substantially when decimal multiplicities were assayed (). Prolonged cultivation, accompanied by further synthesis and hyphal exposure of the reporter, resulted in saturation of the bioluminescent signal. Over all time points of sampling, a statistically significant correlation between initial spore numbers and resulting luminescence signals was evident.
Figure 3. Bioluminescent properties of the reporter strain expressing surface-exposed GLuc. (A) AfS75 displays a flash kinetic of the bioluminescent signal. Emission of photons after substrate addition to 1 × 106 germinated conidia was monitored for approximately six minutes to result in a rapid, nonlinear decay of luminescence. (B) Bioluminescence can be detected from fungal structures formed from at least 1,000 conidia as early as 4 h. Increased spore numbers or extended incubation times result in increases of signal intensities. Subtracted background values from blank medium were in a range of 1–2 × 102 photons per second.
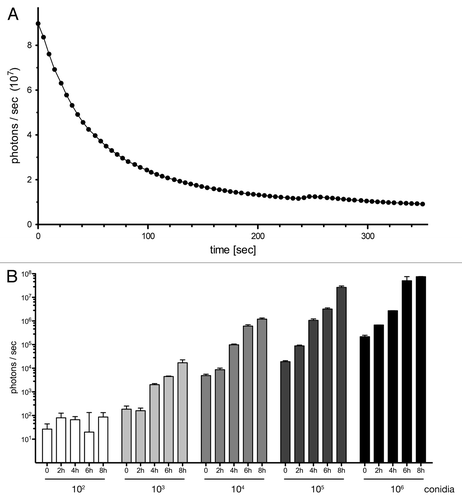
In view of that, we had successfully generated an A. fumigatus strain expressing the bioluminescent reporter GLuc and displaying it on its cell surface, allowing rapid and sensitive detection of luminescence after adding the substrate coelenterazine to swollen or germinated conidia as well as to hyphae.
The bioluminescence reporter strain allows non-invasive imaging in neutropenic mice
Given the high sensitivity of the Gaussia luciferase reporter combined with unhampered substrate accessibility when targeted to the fungal cell wall, we were interested in the bioluminescence imaging capacities of strain AfS75 in an animal model for aspergillosis. To that end, inbred BALB/c mice were immunosuppressed by the repetitive application of cyclophosphamide to result in prolonged neutropenia.Citation28 Pulmonary infections performed in these animals did, however, result in detectable photon fluxes only when high inocula of about 106 conidia were used and directly after the substrate coelenterazine had been applied intravenously (data not shown). Even then, signals could merely be detected after several days post-infection when animals already suffered severely from disease progression due to the high infection doses. In consequence, the rapid decline in photon emission activity by the GLuc enzyme accompanied by instability or limited bioavailability of its substrate does not allow for convenient in vivo imaging of pulmonary infections by the transgenic strain AfS75 in susceptible mice.
To test an alternative as well as relevant model of aspergillosis, cutaneous infections of neutropenic mice were performed following the experimental layout of Ben-Ami et al.Citation13 While these authors used nude (nu/nu) BALB/c mice infected with A. fumigatus strains and followed progression by external caliper measurements of lesion sizes during the course of infection, we reasoned that the bioluminescent strain AfS75 would allow a more sensitive and quantitative assessment of fungal growth during cutaneous aspergillosis. Mice were treated with cyclophosphamide to induce neutropenia and their dorsal fur was removed. Cutaneous aspergillosis was induced by injection of 5 × 105 conidia from AfS75 or its wild-type progenitor ATCC 46645 in the dorsal flanks, which did not result in pronounced weight loss, suffering or mortality over time. Already at one day post-infection, a clear and well-defined bioluminescent signal could be detected when injecting a solution of coelenterazine subcutaneously at the site of infection, but only for AfS75, while the ATCC 46645 wild-type-infected contralateral side did not display any luminescence above background levels (). Also mock-infected flanks did not emit luminescence after substrate injection. Notably, immunocompetent animals that had not been treated with cyclophosphamide were able to clear the infection entirely, as estimated from their dorsal bioluminescence signals and low (< 0.5) galactomannan indices determined from the corresponding tissues. Photon fluxes from the AfS75-infected sites of each neutropenic animal varied only to a limited degree over the course of infection, being followed for seven days post-infection, which is most likely attributed to the relatively high loads of fungal inocula that might result in a saturated bioluminescence signal. Between animals, differences in signal intensities were evident, reflecting the natural variance in infection series. Fungal burdens in the infected tissues (), as assessed by galactomannan immunoassays, rose over the course of infection (). However, no significant correlation of signal intensities with tissue weight-corrected galactomannan indices (cGMI) could be deduced from the bioluminescence images.
Figure 4. Longitudinal study of cutaneous aspergillosis in neutropenic and immune competent BALB/c mice. (A) Animals were infected subcutaneously with 5 × 105 conidia of bioluminescent strain AfS75 (GLuc4) or its wild-type progenitor ATCC 46645 (ATCC) at the contralateral side, and emission of photons after injection of the G. princeps luciferase substrate coelenterazine was followed for one minute up to seven days post-infection (dpi). Bioluminescent signals manifested only from the AfS75-infected areas, whereas the ATCC strain did not result in any background luminescence. Variations in signal intensity ranges were evident as indicated by the differing scales of luminescence. Immune competent animals were able to completely clear the infection within several days. (B) Histopathologic GMS specimen (100x) of representative dorsal tissue after three days post-infection with 5 × 105 conidia of AfS75, demonstrating subcutaneous localization of a hyphal cluster. (C) Fungal burdens increased over the course of cutaneous infection as estimated by corrected galactomannan indices (cGMIs); however, no statistically significant correlation of these to bioluminescence signal intensities could be deduced (not shown).
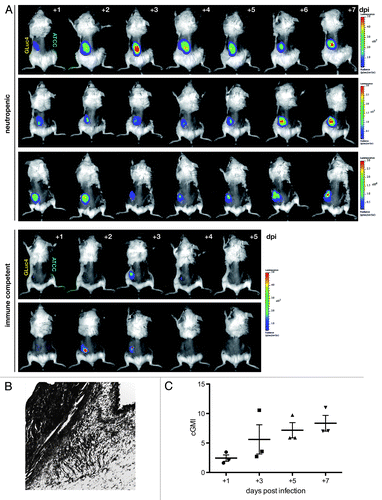
To estimate the minimal dose of infection that would result in a clear bioluminescent signal, decimal multiplicities of AfS75 conidia ranging from 102 to 106 were used in a further infection series. Light emissions from infected dorsal tissues were monitored for three days post-infection to reveal that as little as 103 conidia of strain AfS35 resulted in a detectable and stable bioluminescent signal over the course of infection (). Most lucid signals, however, could be detected from doses of 105 and 106 infectious propagules. A clear correlation with the infection dose and photon emission could be deduced for the early phase of infection when determining photon fluxes eight hours after infection (); at later time points, this correlation was lost because of considerable variations between the monitored animals, and also no correlations to cGMI values could be deduced (data not shown).
Figure 5. Non-invasive imaging of bioluminescence with increasing amounts of fungal spores. (A) Neutropenic animals were infected with the indicated numbers of conidia from reporter strain AfS75 and luminescent signals were monitored at the indicated time points post-infection. (B) Photon fluxes emitted from infected regions correlate to the initial infection doses, as determined from signal intensities sampled from two to three animals eight hours after infection.
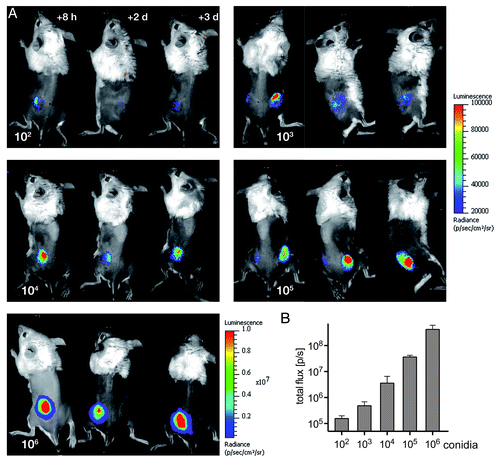
Most importantly, we assessed the possibility to monitor antifungal drug efficiency in this aspergillosis model by in vivo imaging of bioluminescence. For that purpose, cohorts of neutropenic animals were administered two established antifungal substances with different modes of action, amphothericin B (AmB) and posaconazole, from the day of infection with 5 × 105 AfS75 conidia on, and photon fluxes were measured directly after subcutaneous substrate injection. Imaging series clearly revealed that oral treatment with the antimycotic agent posaconazole resulted in effective resolution of the fungal infection within five days, while a clear bioluminescent signal could be detected for the AmB-treated animals (). The latter observation was most likely due to limited bioavailability of the drug at the cutaneous site of infection, as direct injection of AmB into the seat of infection resulted in rapid clearance of the bioluminescence, demonstrating the general susceptibility of the reporter strain to antifungal treatment with amphotericin B. In essence, this demonstrates the usefulness of the bioluminescence readout from transgenic strain AfS75 as an indicator for failed or successful antifungal therapy.
Figure 6. The bioluminescent reporter strain AfS75 allows in vivo monitoring of antifungal therapy. Shown are animals from an in vivo imaging series of neutropenic mice infected with 5 × 105 conidia of AfS75 (GLuc4) and the wild-type isolate ATCC 46645 (ATCC), respectively, that had been treated with amphothericin B deoxycholate (AmB, 5 mg/kg/day i.p.) or posaconazole (40 mg/kg/day per os), demonstrating failure of antifungal treatment by AmB or complete clearance of the fungal infection by posaconazole as evident from the persistence and absence, respectively, of bioluminescence. When applying AmB directly at the seat of infection (+ AmB s.c.), bioluminescence was resolved within two days.
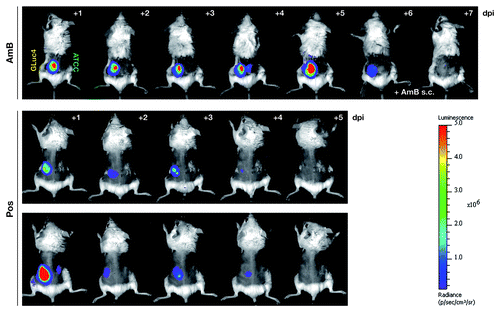
Discussion
Non-invasive imaging techniques have become a cornerstone in characterizing the intimate interplay between pathogens and their hosts, especially with respect to infection processes or mechanisms of immune defense.Citation14 Luminescent reporters derived from biological systems have proven extremely useful in such purposes, as they allow sensitive and dynamic imaging in various disease models, thereby reducing the numbers of required animals in such studies. In contrast to bacterial luminescence reporters that are based on the lux operons of Photorhabdus luminescens or Xenorhabdus luminescens and allow constant imaging due to the permanent emission of photons, current eukaryotic systems do require addition of the corresponding luciferase substrate, such as luciferin for the Photinus pyralis luciferase or coelenterazine for the G. princeps one. Substrate availability and stability in the respective infection model system is crucial for in vivo imaging, and limitations there might restrict the usefulness of one or the other bioluminescence system in different disease models. Here, we followed the approach of Enjalbert et al.Citation21 that localized the highly sensitive luciferase of the copepod G. princeps, encoded by a synthetic, codon-optimized gene, to the fungal cell surface, resulting in better substrate accessibility as a strict prerequisite for luminescence. In preliminary experiments expressing the GLuc enzyme cytoplasmatically in A. fumigatus, we were unable to detect any bioluminescence after coelenterazine addition (our unpublished results), which resembles data from the commensal C. albicans. In view of that does the fungal cell wall generally appear to exclude the substrate from the intracellular compartment. Fusing the encoding gene to signal sequences for secretion and linking it to a validated, GPI-anchored cell wall protein allowed surface exposure of the luciferase enzyme, as confirmed by two alternative experimental approaches. An evident advantage of targeting the Gaussia bioluminescence reporter to the fungal surface lies in its open substrate accessibility, which likely adds on the brightness of the resulting recombinant A. fumigatus strain. Yet, the apparent instability and limited bioavailability of the substrate coelenterazine combined with the recognized flash kinetic of GLuc luminescence hampered its use as imaging reporter in pulmonary or systemic infections, which are the most common courses of disease caused by this environmental mold. It will be of interest to assess the imaging capacities of alternative luciferases, such as firefly luciferase that uses luciferin as substrate and displays prolonged, glow-like bioluminescent kinetics as compared with GLuc,Citation27 in combination with the surface display system described in this study.
In the cutaneous aspergillosis model, however, the surface-exposed Gaussia luciferase proved to be a versatile bioluminescence reporter, allowing sensitive pathogen detection repeatedly from as little as 1,000 infectious propagules. This stresses site-specific differences in pathogenesis and performance of bioluminescence imaging of aspergillosis. Fungal infections could be followed by exogenous addition of the substrate to the site of infection, which bears the obstacle of proper positioning that might hamper quantitative analyses. The resulting animal-to-animal variations might furthermore be augmented by a general drawback of luciferase enzymes, which is their oxygen dependence. Limited oxygen availability is a general phenomenon to occur at later stages of infection and such hypoxic conditions are likely to interfere with bioluminescence. Also, stability of the expression module integrated in the fungal genome has to be taken into account. When analyzing clonal descendants from a conidial population cultured under non-selective conditions in vitro or in vivo, we did not observe a substantial decrease in numbers of colony forming units when plating again on selective culture medium (our unpublished results). Yet, we were able to monitor infections semi-quantitatively for several days, noticing increases in fungal load and, most importantly, the complete clearance upon antifungal treatment or in immunocompetent animals. As such, our system represents a significant and consistent further development of the recently introduced, pivotal model of cutaneous aspergillosis by Kontoyiannis and co-workers.Citation13 There, nude mice were further immunocompromised and infected in their thighs by injection of high inocula to result in skin lesions, the area of which correlated with the tissue fungal burden to a certain degree. Interestingly, we were unable to detect similar skin lesions when infecting our immunosuppressed BALB/c mice at the dorsal flanks, which might relate to the differing genetic background, the alternative site of infection, or the reduced infection doses. And indeed, when inoculating neutropenic BALB/c mice with 10-fold higher doses in the thigh regions, prominent lesions could be spotted after five days, but not when applying this inoculum of 5 × 106 conidia to the dorsal flanks (data not shown). In consequence, non-invasive imaging is the method of choice in this type of cutaneous aspergillosis model because fungal particles are directly visualized, so it represents an advantageous experimental approach for pathogen detection and surveillance of disease progression. Of particular interest, we were able detect complete elimination of the fungal pathogen over time when animals were treated with the established antifungal drug posaconazole. This is in sharp contrast to the skin lesions’ readout, dimensions of which were significantly reduced but did not disappear in the course of fungicidal treatment. Accordingly, the bioluminescent reporter allows dynamic and non-invasive monitoring of cutaneous A. fumigatus infections and their resolution either in the course of a proper immune response or during antibiosis. As a further aspect, this particular GLuc reporter strain appears as well suited for multiplex imaging studies employing alternative bioluminescence reporters that are expressed, for instance, by transgenic cells of the host immune system.
The use of a non-invasive imaging technique as experimental approach to follow fungal infection furthermore allows screening approaches in high-throughput, requiring lower numbers of animals. Moreover, assessing the virulence of fungal mutants, which correlates to growth at the site of infection, appears feasible and convenient in the bioluminescence reporter background, allowing to assess the contribution of distinct A. fumigatus genes and their products to virulence of this fungal pathogen.
Materials and Methods
Strains, media, transformations
The Escherichia coli strain DH5αCitation29 was used for cloning procedures. Plasmid-carrying E. coli strains were routinely grown in at 37°C LB liquid medium (1% peptone, 0.5% yeast extract, 0.5% NaCl) under selective conditions (100 μg/ml ampicillin).
The wild-type isolate ATCC 46645Citation30 served as recipient Aspergillus fumigatus strain and was generally cultured in nitrate-based minimal mediumCitation31 at 37°C. Integration of the expression vector carrying the ptrA marker geneCitation32 was selected for by the presence of pyrithiamine at a concentration of 0.1 μg/ml.
E. coli competent cells were prepared and transformed following the calcium chloride method.Citation33 Protoplast fusion-mediated transformation of A. fumigatus was performed following essentially the procedure of Punt and van den Hondel.Citation34
Construction of plasmids and recombinant A. fumigatus strains
Standard protocols of recombinant DNA technology were performed.Citation35 Phusion™ high-fidelity DNA polymerase was generally used in polymerase chain reactions (PCRs) and essential cloning steps were verified by sequencing.
The plasmid pSK481 was constructed by inserting a synthetic 1.53 kb DNA fragment acquired from the company GENEART AG (Regensburg, Germany) in the PmeI site of the Aspergillus expression vector pSK379.Citation25,Citation26 The coding sequence of the chimeric gene is based on the A. oryzae TglA signal sequence (GenBank accession number BAA92327.1), the Rhizopus oryzae triacylglycerol lipase preproprotein (BAG16821.1), the G. princeps luciferase (AAG54095.1), and the A. fumigatus MP1 protein (AFUA_4G03240) and was deposited at GenBank under accession number JN223418.
Luciferase assays for bioluminescence
The kinetic of bioluminescence emitted from AfS75 was determined in a Victor™ Light luminescence counter (PerkinElmer) as follows: In a white 96-well flat bottom plate, 1 × 106 conidia per well were incubated in Aspergillus minimal medium overnight at 30°C to allow germination. Culture supernatants were removed, germlings were washed three times with phosphate-buffered saline (PBS), 200 µl Gaussia-Glow-Juice (PJK GmbH) were added, and photon fluxes (in counts per second, cps) were determined every 5 sec for time periods of up to 6 min after substrate injection.
Localization studies
Cellular localization of the GLuc enzyme in AfS75 was assessed by indirect immunofluorescence following essentially the protocols of MomanyCitation36 and Oakley and Osmani.Citation37 In particular, 1 × 102 conidia in minimal medium were incubated in Lab-Tek™ Chamber Slides overnight at 37°C to allow germination, washed in PEM buffer (50 mM PIPES pH 6.7, 25 mM EGTA pH 7.0, 5 mM MgSO4), and fixed in IF fixer solution (3.7% formaldehyde, 5% DMSO, 25 mM EGTA pH 7.5, 5 mM MgSO4) for 30 min at room temperature. After washing with 0.1% PBSA (0.1% BSA in PBS) and pre-inbubation for 1 h at room temperature in 1% PBSA, 150 µl of a 1:200 dilution in 0.1% PBSA of a monoclonal anti-GLuc IgG 2a antibody (PJK GmbH) were added for 2 h at room temperature. The primary antibody was removed and five washing steps with 0.1% PBSA were performed, before 150 µl of the rhodamine-conjugated anti-mouse IgG2a (γ-chain) rabbit (Rockland Immunochemicals) secondary antibody, 1:2000 diluted in 0.1% PBSA, was added for 1 h at room temperature. Again, the antibody solution was removed and five washes with 0.1% PBSA were performed, before samples were mounted in DakoCytomation Fluroescent Mounting Medium (Dako GmbH) and analyzed by fluorescence microscopy.
Partial trypsin digests of germlings to release surface-exposed proteins were performed according to Herńez et al.Citation38 1 × 108 conidia from AfS75 were incubated in 30 ml complete medium for 8 h at 37°C and germination was validated by microscopic inspection. Germlings were washed after centrifugation with PBS for three times and resuspended in 10 ml of a 25 mM NH4HCO3 solution containing 20 µg/ml trypsin (Sigma, T4665) and 5 mM dithiothreitol (DTT). Aliquots of 1 ml were incubated at 37°C for 5, 10, 15 and 25 min, respectively. After incubation, germlings were washed again in PBS for three times and resuspended in 100 µl PBS. For triplicate luminescence measurements, 20 µl of the suspensions each, equaling approximately 2 × 106 germlings, were assayed in a black clear bottom 96-well plate after addition of 200 µl Gaussia-Glow-Juice in a luminescence counter. In parallel, survival rates were determined by quantifying colony forming units grown on minimal medium.
Murine model of cutaneous aspergillosis and in vivo imaging
Following the approach of Ben-Ami et al,Citation13 female BALB/c mice (Charles Rivers) weighting 18–20 g were rendered neutropenic by the repeated use of cyclophosphamide (150 mg/kg i.p. at days -3, -1, +2 and every other following day). Infections were performed with homogenous suspensions of freshly harvested conidia in PBS/0.005% Tween 80 by subcutaneous injection in dorsal sections after fur removal. For non-invasive imaging under ketamine/xylazine anesthesia, 100 µl of a 15.6 µM coelenterazine solution were injected subcutaneously at the site of infection and animals were immediately put in an IVIS® Lumina II imaging chamber (Caliper Life Sciences) for bioluminescence detection within an exposure time of one minute. Images were analyzed by the LivingImage® 3.1 software.
Tissue fungal burden by galactomannan assay
Skin tissue with underlying muscles was excised from infected areas, weighed, and homogenized in PBS. After centrifugation, supernatants of homogenates were diluted 1:250 and assayed at the Institute of Hygienics and Microbiology, University Clinic Würzburg, Germany with an enzyme immunoassay kit (Platelia Aspergillus EIA from Biorad) detecting galactomannan contents. The resulting galactomannan indices (GMI) were corrected for tissue weights.
Acknowledgments
We are indebted to Astrid Dylda, Axelle Degla, and Michaela Dümig for excellent technical assistance and to all other members of the Institute for Molecular Infection Biology for continuous support. Werner J. Heinz is thanked for providing posaconazole, Thomas Hartmann for qPCR analyses, Hilde Merkert for providing assistance with fluorescence microscopy, and Christian Erck from the HZI Braunschweig, Germany for typing the monoclonal α-GLuc antibody. Funding was received from the German José Carreras Leukaemia Foundation (Cooperation Project DJCLS 10/15), the Free State of Bavaria, and the University of Würzburg.
Disclosure of Potential Conflict of Interest
No potential conflicts of interest were disclosed.
References
- McNeil MM, Nash SL, Hajjeh RA, Phelan MA, Conn LA, Plikaytis BD, et al. Trends in mortality due to invasive mycotic diseases in the United States, 1980-1997. Clin Infect Dis 2001; 33:641 - 7; http://dx.doi.org/10.1086/322606; PMID: 11486286
- Warnock DW. Fungal diseases: an evolving public health challenge. Med Mycol 2006; 44:697 - 705; http://dx.doi.org/10.1080/13693780601009493; PMID: 17127626
- Ascioglu S, Rex JH, de Pauw B, Bennett JE, Bille J, Crokaert F, et al. Defining opportunistic invasive fungal infections in immunocompromised patients with cancer and hematopoietic stem cell transplants: an international consensus. Clin Infect Dis 2002; 34:7 - 14; http://dx.doi.org/10.1086/323335; PMID: 11731939
- Talbot GH, Bradley J, Edwards JE Jr., Gilbert D, Scheld M, Bartlett JG. Bad bugs need drugs: an update on the development pipeline from the Antimicrobial Availability Task Force of the Infectious Diseases Society of America. Clin Infect Dis 2006; 42:657 - 68; http://dx.doi.org/10.1086/499819; PMID: 16447111
- Warnock DW. Trends in the epidemiology of invasive fungal infections. Nihon Ishinkin Gakkai zasshi 2007; 48:1 - 12; http://dx.doi.org/10.3314/jjmm.48.1; PMID: 17287717
- Burgos A, Zaoutis TE, Dvorak CC, Hoffman JA, Knapp KM, Nania JJ, et al. Pediatric invasive aspergillosis: a multicenter retrospective analysis of 139 contemporary cases. Pediatrics 2008; 121:e1286 - 94; http://dx.doi.org/10.1542/peds.2007-2117; PMID: 18450871
- Patterson TF, Kirkpatrick WR, White M, Hiemenz JW, Wingard JR, Dupont B, et al. Invasive aspergillosis. Disease spectrum, treatment practices, and outcomes. I3 Aspergillus Study Group. Medicine 2000; 79:250 - 60; http://dx.doi.org/10.1097/00005792-200007000-00006; PMID: 10941354
- Etienne KA, Subudhi CP, Chadwick PR, Settle P, Moise J, Magill SS, et al. Investigation of a cluster of cutaneous aspergillosis in a neonatal intensive care unit. J Hosp Infect 2011; In press http://dx.doi.org/10.1016/j.jhin.2011.06.012; PMID: 21840084
- Murray CK, Loo FL, Hospenthal DR, Cancio LC, Jones JA, Kim SH, et al. Incidence of systemic fungal infection and related mortality following severe burns. Burns 2008; 34:1108 - 12; http://dx.doi.org/10.1016/j.burns.2008.04.007; PMID: 18691821
- van Burik JA, Colven R, Spach DH. Cutaneous aspergillosis. J Clin Microbiol 1998; 36:3115 - 21; PMID: 9774549
- Walsh TJ. Primary cutaneous aspergillosis—an emerging infection among immunocompromised patients. Clin Infect Dis 1998; 27:453 - 7; http://dx.doi.org/10.1086/514718; PMID: 9770139
- Zaas AK, Steinbach WJ. Mammalian models of aspergillosis. In: Goldman GH, Osmani SA, eds. The Aspergilli: Genomics, Medical Aspects, Biotechnology, and Research Methods. Boca Raton: CRC Press, 2007:401-12.
- Ben-Ami R, Lewis RE, Leventakos K, Latgé JP, Kontoyiannis DP. Cutaneous model of invasive aspergillosis. Antimicrob Agents Chemother 2010; 54:1848 - 54; http://dx.doi.org/10.1128/AAC.01504-09; PMID: 20145078
- Andreu N, Zelmer A, Wiles S. Noninvasive biophotonic imaging for studies of infectious disease. FEMS Microbiol Rev 2011; 35:360 - 94; http://dx.doi.org/10.1111/j.1574-6976.2010.00252.x; PMID: 20955395
- Contag CH, Contag PR, Mullins JI, Spilman SD, Stevenson DK, Benaron DA. Photonic detection of bacterial pathogens in living hosts. Mol Microbiol 1995; 18:593 - 603; http://dx.doi.org/10.1111/j.1365-2958.1995.mmi_18040593.x; PMID: 8817482
- Hutchens M, Luker GD. Applications of bioluminescence imaging to the study of infectious diseases. Cell Microbiol 2007; 9:2315 - 22; http://dx.doi.org/10.1111/j.1462-5822.2007.00995.x; PMID: 17587328
- Zinn KR, Chaudhuri TR, Szafran AA, O'Quinn D, Weaver C, Dugger K, et al. Noninvasive bioluminescence imaging in small animals. ILAR J 2008; 49:103 - 15; PMID: 18172337
- d'Enfert C, Vecchiarelli A, Brown AJ. Bioluminescent fungi for real-time monitoring of fungal infections. Virulence 2010; 1:174 - 6; http://dx.doi.org/10.4161/viru.1.3.11119; PMID: 21178436
- Brock M, Jouvion G, Droin-Bergere S, Dussurget O, Nicola MA, Ibrahim-Granet O. Bioluminescent Aspergillus fumigatus, a new tool for drug efficiency testing and in vivo monitoring of invasive aspergillosis. Appl Environ Microbiol 2008; 74:7023 - 35; http://dx.doi.org/10.1128/AEM.01288-08; PMID: 18820063
- Ibrahim-Granet O, Jouvion G, Hohl TM, Droin-Bergere S, Philippart F, Kim OY, et al. In vivo bioluminescence imaging and histopathopathologic analysis reveal distinct roles for resident and recruited immune effector cells in defense against invasive aspergillosis. BMC Microbiol 2010; 10:105; http://dx.doi.org/10.1186/1471-2180-10-105; PMID: 20377900
- Enjalbert B, Rachini A, Vediyappan G, Pietrella D, Spaccapelo R, Vecchiarelli A, et al. A multifunctional, synthetic Gaussia princeps luciferase reporter for live imaging of Candida albicans infections. Infect Immun 2009; 77:4847 - 58; http://dx.doi.org/10.1128/IAI.00223-09; PMID: 19687206
- Tannous BA. Gaussia luciferase reporter assay for monitoring biological processes in culture and in vivo. Nat Protoc 2009; 4:582 - 91; http://dx.doi.org/10.1038/nprot.2009.28; PMID: 19373229
- Hama S, Tamalampudi S, Shindo N, Numata T, Yamaji H, Fukuda H, et al. Role of N-terminal 28-amino-acid region of Rhizopus oryzae lipase in directing proteins to secretory pathway of Aspergillus oryzae. Appl Microbiol Biotechnol 2008; 79:1009 - 18; http://dx.doi.org/10.1007/s00253-008-1502-6; PMID: 18496686
- Adachi T, Ito J, Kawata K, Kaya M, Ishida H, Sahara H, et al. Construction of an Aspergillus oryzae cell-surface display system using a putative GPI-anchored protein. Appl Microbiol Biotechnol 2008; 81:711 - 9; http://dx.doi.org/10.1007/s00253-008-1687-8; PMID: 18813924
- Szewczyk E, Krappmann S. Conserved regulators of mating are essential for Aspergillus fumigatus cleistothecium formation. Eukaryot Cell 2010; 9:774 - 83; http://dx.doi.org/10.1128/EC.00375-09; PMID: 20348388
- Wagener J, Echtenacher B, Rohde M, Kotz A, Krappmann S, Heesemann J, et al. The putative α-1,2-mannosyltransferase AfMnt1 of the opportunistic fungal pathogen Aspergillus fumigatus is required for cell wall stability and full virulence. Eukaryot Cell 2008; 7:1661 - 73; http://dx.doi.org/10.1128/EC.00221-08; PMID: 18708564
- Tannous BA, Kim DE, Fernandez JL, Weissleder R, Breakefield XO. Codon-optimized Gaussia luciferase cDNA for mammalian gene expression in culture and in vivo.. Mol Ther 2005; 11:435 - 43; http://dx.doi.org/10.1016/j.ymthe.2004.10.016; PMID: 15727940
- Smith JM, Tang CM, Van Noorden S, Holden DW. Virulence of Aspergillus fumigatus double mutants lacking restriction and an alkaline protease in a low-dose model of invasive pulmonary aspergillosis. Infect Immun 1994; 62:5247 - 54; PMID: 7960101
- Woodcock DM, Crowther PJ, Doherty J, Jefferson S, DeCruz E, Noyer-Weidner M, et al. Quantitative evaluation of Escherichia coli host strains for tolerance to cytosine methylation in plasmid and phage recombinants. Nucleic Acids Res 1989; 17:3469 - 78; http://dx.doi.org/10.1093/nar/17.9.3469; PMID: 2657660
- Hearn VM, Mackenzie DW. Mycelial antigens from two strains of Aspergillus fumigatus: an analysis by two-dimensional immunoelectrophoresis. Mykosen 1980; 23:549 - 62; PMID: 6160400
- Käfer E. Meiotic and mitotic recombination in Aspergillus and its chromosomal aberrations. Adv Genet 1977; 19:33 - 131; http://dx.doi.org/10.1016/S0065-2660(08)60245-X; PMID: 327767
- Kubodera T, Yamashita N, Nishimura A. Pyrithiamine resistance gene (ptrA) of Aspergillus oryzae: cloning, characterization and application as a dominant selectable marker for transformation. Biosci Biotechnol Biochem 2000; 64:1416 - 21; http://dx.doi.org/10.1271/bbb.64.1416; PMID: 10945258
- Hanahan D, Jessee J, Bloom FR. Plasmid transformation of Escherichia coli and other bacteria. Methods Enzymol 1991; 204:63 - 113; http://dx.doi.org/10.1016/0076-6879(91)04006-A; PMID: 1943786
- Punt PJ, van den Hondel CA. Transformation of filamentous fungi based on hygromycin B and phleomycin resistance markers. Methods Enzymol 1992; 216:447 - 57; http://dx.doi.org/10.1016/0076-6879(92)16041-H; PMID: 1479914
- Sambrook J, Fritsch EF, Maniatis T. Molecular Cloning: A Laboratory Manual. Cold Spring Harbor, NY: Cold Spring Harbor Laboratory Press, 1989.
- Momany M. Cell biology of the duplication cycle in fungi. In: Talbot NJ, ed. Molecular and cellular biology of filamentous fungi: a practical approach. Oxford: Oxford University Press, 2001.
- Oakley BR, Osmani SA. Cell-cycle analysis using the filamentous fungus Aspergillus nidulans. In: Fantes P, Brooks R, eds. The cell cycle: a practical approach. Oxford: Oxford University Press, 1993.
- Herńez ML, Ximenez-Embun P, Martinez-Gomariz M, Gutierrez-Blazquez MD, Nombela C, Gil C. Identification of Candida albicans exposed surface proteins in vivo by a rapid proteomic approach. J Proteomics 2010; 73:1404 - 9; http://dx.doi.org/10.1016/j.jprot.2010.02.008; PMID: 20167299