ABSTRACT
“Let food be thy medicine and medicine be thy food” was expressed by Hippocrates more than 2000 years ago and the health benefits of natural food products have been considered for different goals since the ancient times. As natural phytochemicals, phenolic compounds (PCs) are a major class of semi-water-soluble compounds (from fruit and vegetable sources) with one or more benzene rings that are generally found in nature as glycosides. According to a review of the literature, food scientists have studied the many health benefits of these compounds against pernicious human diseases (HDs). Today, choosing a healthy diet has become an essential part of healthy living and fitness. Many studies have shown that people who follow a specific diet (especially polyphenol-rich diets) are at a low risk for a range of chronic diseases, such as obesity, diabetes, cancer, heart disease, etc. The present research is a comprehensive review of studies on PCs with a focus on HDs that seeks to find out why some PCs have received such considerable attention all over the world. Data were collected from the Scopus database and a hierarchical cluster analysis was used with Ward’s method to analyze the data. The results showed that three countries most involved in research on PCs are USA, China, and India, and also found that quercetin, catechin, and kaempferol are the three most studied PCs. Cardiovascular diseases, cancer, obesity, diabetes, and infectious diseases are the major treatment targets for PCs. The review of the literature showed that these compounds share a common molecular mode of action against HDs. The Journal of Agriculture and Food Chemistry, Food Chemistry, PLOS ONE, and Planta Medica are also the most popular journals for covering original research on PCs. However, this study seeks to facilitate future studies on PCs through its findings.
Abbreviations: ROS: Reactive oxygen species; DPPH: 2,2-diphenyl-1-picrylhydrazyl; ABTS: 2,2′-Azino-bis (3-ethylbenzthiazoline-6-sulfonic acid); DMPD: Dimethyl-4-phenylenediamine; COX: Cyclooxygenase; PI3K: phosphoinositide 3-kinase; ERK: extracellular signal-regulated kinase; GalR: galanin receptor; PKC: protein kinase C delta type; Bcl-2: B-cell lymphoma 2; PPARγ: peroxisome proliferator-activated receptor gamma; LDL: Low-density lipoprotein; HIV: human Immunodeficiency Virus; HDACs; histone deacetylases; SREBP-1c; sterol regulatory element-binding proteins; GLUT: glucose transferase; RAGE: receptor for advanced glycation end products; TRAIL: tumor necrosis factor-related apoptosis-inducing ligand; TNF-α: tumor necrosis factor α; PKC: protein kinase C; IRS: insulin receptor substrate; NFκB: nuclear factor-κB; JNK: c-jun amino terminal kinase; MAPK: mitogen activated protein kinase; PEPCK: phosphoenolpyruvatecarboxykinase; G6Pase: glucose-6-phosphatase; RNS: reactive nitrogen species; VLDL: very low-density lipoprotein; HDL: high-density lipoprotein; CaMK II: calmodulin kinase II; MCP: Monocyte Chemoattractant Protein; JAK: Janus kinase; STAT: signal transducers and activators of transcription; EGFR: epidermal growth factor receptor; VEGF: vascular endothelial growth factor; VEGFR: vascular endothelial growth factor receptor; PKA: protein kinase A; PTK: protein-tyrosine kinase;AP-1: activator protein 1; Her2/neu: human epidermal growth factor receptor 2;mTOR: the mechanistic target of rapamycin; IL: interleukin; COMT: cathecol-o-methyl transferase; UGT: uridine-5ʹ-diphosphate glucuronosyltransferase; MMP-2: matrix metalloproteinase-2; AhR: the aryl hydrocarbon receptor; CYP: cytochrome P450; DR: death receptor; TGL: triglycerides; TC: total cholesterol; EGCG: (-)-epigallocatechingallate; EGC: (-)-epigallocatechin; ECG: (-)-epicatechingallate; EC: (-)-epicatechin; ELK1: ETS domain-containing protein Elk-1; MAPK: Mitogen-activated protein kinase kinase; HGFR: hepatocyte growth factor receptor; HDAC: histone deacetylase; DNMT: DNA methyl transferase; HAT; histone acetyltransferase; C/EBP-α: CCAAT-enhancer-binding proteins; LPL: Lipoprotein lipase; iNOS: inducible nitric oxide synthase.
Introduction
“Let food be thy medicine and medicine be thy food” was expressed by Hippocrates more than 2000 years ago and the health benefits of natural food products have been considered since ancient times.[Citation1] Plant foods such as vegetables and fruits provide calories and other essential nutrients for the human body and play a crucial role in human health.[Citation2–Citation5] Studies on plant secondary metabolites have been increasing over the last 16 years and these compounds have been seriously considered for their capacity to improve human health.[Citation6] These phytochemicals are known to play a critical role in the adaptation of plants to their environment[Citation7], but also represent an important source of active pharmaceuticals.[Citation8] Almost 200 years of modern chemistry and biology have explained the role of primary metabolites in basic life functions such as growth and development, respiration, storage, and reproduction.[Citation9–Citation11] Kossel[Citation12] was the first scientist who defined secondary metabolites in comparison to primary ones. Thanks to the improvement of biochemical assays in the middle of the 20th century, Czapek[Citation13] was the second person who described new roles for phytochemicals and coined the term “end products” for these compounds. PCs are a diverse, bioactive, and pervasive category of plant secondary metabolites that comprise an essential part of the human diet and are of considerable interest due to their biological properties.[Citation14–Citation17] During the last few decades, several studies have examined the health benefits of PCs.[Citation18–Citation20] The regular consumption of polyphenol-rich foods may help decrease the incidence of cardiovascular diseases[Citation21], colon cancer[Citation22], liver disorders[Citation23], obesity[Citation24,Citation25], diabetes[Citation26], etc. In plants, these compounds are commonly synthesized as defense agents against physiological and environmental stimulators.[Citation18,Citation27,Citation28] Regarding the health benefits of PCs for humans[Citation29] (), these compounds have received more attention in recent years and many aspects of their chemical and biological activities have been identified and evaluated.[Citation30,Citation31]
The advantages of PCs include their accessibility, the specificity of their response, and their low toxicity, while the main problems with these compounds are their rapid metabolism and low bioavailability.[Citation28,Citation32] Foods contain complex mixtures of PCs and numerous factors may affect the concentrations of PCs in plants and food items[Citation20], including environmental (e.g. sun exposure, rainfall, different type of cultivation, the fruit yield of trees, etc.) and biochemical (e.g. the degree of ripeness, storage, and the method of culinary preparation) factors.[Citation33,Citation34]
Taken together, PCs, especially their glycosides, are the most important plant secondary metabolites in human diet that have wide-ranging health benefits for humans.[Citation35] Compounds from natural sources seem to have found a special place in the pharmaceutical industry in the last few decades and are now being used for the design and development of new pharmacological drugs.[Citation36] In addition, these compounds are widely used in pharmaceutical experiments in order to improve the bio-efficacy and bioavailability of conventional drugs. A survey of the literature on PCs, covering over 20000 published papers in this field, showed that more than half of these molecules have antibacterial, antifungal, anti-inflammatory, and antitumor activities[Citation37], which reflects the extensive benefits of PCs for human societies.[Citation38] The large numbers of studies conducted on PCs throughout the world and published and indexed in different scientific databases pose three questions. First, which PCs are the most studied in the world? Second, which countries study which PCs the most? And finally, which signaling pathways are affected by PCs that give them these health benefits for humans? This review will mainly focus on the most-studied PCs and the countries most involved in research on PCs and will also provide a comprehensive overview of the biological effects of the most-studied dietary PCs and their health benefits.
Table 1. The list of critical keywords and setting selected for document searching in Scopus database (2000–2016)
Table 2. Frequency of the most studied PCs per country (2000–2016)
Table 3. The role of dietary catechins in epigenetics events
Table 4. The total number of documents published regarding research on PCs for HD per country (2000–2016)
Figure 4. Number of published papers per country for (A) polyphenols; (B) flavonoids; (C) lignans; and (D) stilbenes.
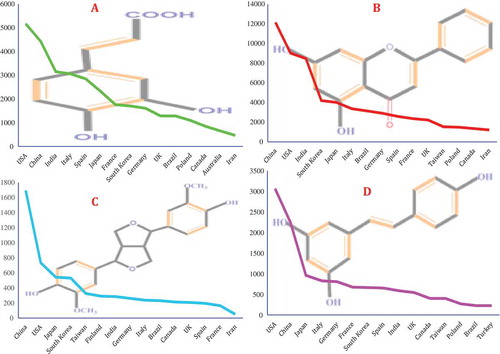
Figure 7. Possible pathway of metabolic conversion of quercetin, an onion flavonoid, during intestinal absorption and inflammation. First, Q4ʹG converts to quercetin by mediating the β-glucosidase enzyme. Second, UGT changes quercetin to Q3GA. In the next step, during inflammation by the mediating of β-glucuronidase and COMT enzymes, Q3GA converts to quercetin and isorahamentin.
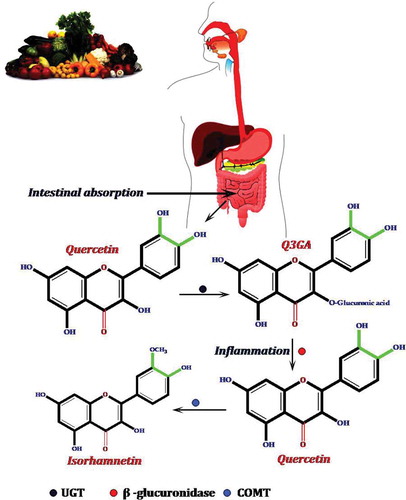
Methodology
This study is an extensive review of the global literature on PCs through a search of Scopus with the keywords detailed in . A total of 128,710 articles were indexed in this database from “2000 to 2016” and the researchers extracted relevant articles using the keywords and analyzed the data obtained using a statistic method. The generated results were classified and ranked for each subject based on the queries. A hierarchical cluster analysis was performed is SPSS 16.0[Citation39] using Ward’s method.[Citation40] This 16-year report can help researchers plan further studies on PCs and also provides a brief overview of the chemical structure of these compounds.
Chemical structure of PCs
PCs can be divided into two main groups: (1) flavonoids and (2) non-flavonoids.[Citation41,Citation42] A distinctive feature of PCs is having one or more aromatic rings with hydroxyl groups attached to them. The characteristics of each group and subgroups have been addressed in the author’s previous paper[Citation14] and in other papers as well.[Citation18,Citation26,Citation27,Citation30,Citation31,Citation43–Citation45]
Extraction strategies
Owing to the recent advances in chemical approaches, a variety of methods currently exist for the extraction, purification, quantification, and identification of PCs. There is a positive correlation between the type of PCs and the strategies used to the extract them from natural sources. Basically, there are five modern methods for the extraction of PCs[Citation46], including (1) solvent extraction; (2) pressurized liquid extraction; (3) ultrasonic-assisted extraction; (4) microwave-assisted extraction; and (5) supercritical extraction. Similar to that in modern methods, conventional liquid–liquid and solid–liquid extraction procedures are frequently used in these methods.[Citation28] Polyphenols structures are such that each method used for their extraction has a specific separation protocol. The time required for extraction, the chemical structure, and the organic solvents all play a crucial role in the extraction procedure. Since PCs are attached to the cell-wall matrix through a glycosidic/ester linkage, their water solubility may be reduced. Studies showed that several extraction approaches require an antioxidant as a basic stabilizer. Alcohols are other suitable solvents that have been used for extraction.[Citation47] For example, methanol and ethanol are the most-used solvents for the separation of Stilbenes from natural sources. These solvents have also been used for the extraction of other polyphenol families. According to some studies, supercritical CO2 and polar/nonpolar co-solvents are the most-used solutions for the accurate extraction of tannin from plant tissues.[Citation28] Alcoholic solvent systems have been used to extract lignans from natural sources.[Citation48] Because lignans collaborate in plant cell-wall formation, alcohols thus appear to be good solvents for the extraction of these compounds. High-performance liquid chromatography and/or gas chromatography/MS spectrometry can also be used for the high-quality extraction of lignans.[Citation28] Studies suggest that the extraction of flavonoids also has both conventional and modern extraction strategies. As noted earlier, given their structure, solubility, and reason for extraction, different separation methods can be used to extract PCs, and the advantages and disadvantages of these methods have been well-documented in a large body of literature.[Citation28,Citation46]
Polyphenol databases
Computer programs (especially online tools) play a crucial role in the advancement of biological sciences in the modern world. Recently, biological databases have been developed for plant secondary metabolites, which describe the chemistry, biochemistry, and biosynthesis of plant chemicals; however, no such database exists that focuses on foods and more precisely on PCs as the major classes of phytochemicals. Phenol-Explorer (http://www.phenol-explorer.eu) is a free web portal and database for exploring, visualizing, and downloading plant polyphenols[Citation49] ().
This database provides biochemical data generated from different analytical platforms from multiple laboratories along with a downloadable 2D structure of PCs in the SDF format. Phenol-Explorer database contains more than 37,000 original data points collected from 638 scientific articles published in peer-reviewed journals. Overall, 502 polyphenols found in 452 foods are indexed in this database; the database is one of the best sources of information on PCs (because of the applicable and attributable information it yields) and how it offers an original resource for clinical and academic research. The USDA Nutrient Data Laboratory Flavonoid Database (https://www.ars.usda.gov/northeast-area/beltsville-md/beltsville-human-nutrition-research-center/nutrient-data-laboratory/) is another powerful database for information on PCs (), which contains values for 500 food items and 28 predominant monomeric dietary flavonoids belonging to five subclasses such as flavonols, flavones, flavanones, flavan-3-ols, and anthocyanidins.[Citation50] Nonetheless, it seems that reserving and evaluating polyphenol data need more databases and the development of these databases can help biologists advance their work on PCs.
Countries’ contributions
According to published articles including the selected keywords in Scopus, a significant difference was observed between the countries that published these reports. All the documents indexed in Scopus were not exactly dedicated to PCs, but included the selected keywords in their title, abstract, full text, etc. shows 15 countries with the largest number of articles published on PCs. As shown in , USA and China are the two top countries for publishing research on PCs, followed by India, South Korea, and Japan.
The three countries with the largest number of published articles containing the keywords “polyphenols”, “flavonoids”, “lignans”, and “stilbenes” were, respectively, the USA, China, India; China, the USA, India; China, the USA, Japan; and the USA, China, Japan. This wide range of research on PCs demonstrates the importance of these compounds in human nutrition research. It seems that the most-developed countries have conducted the largest number of research on these compounds. This finding suggests that PCs have gained the interest of the entire world and studies on these compounds have been increasing in the past decades.
Which compounds received more attention?
Many of the published articles have discussed the most-studied PCs[Citation26,Citation27,Citation31] and can be accessed through databases. To identify how many times the most-studied PCs had been addressed in research, we screened all the retrieved papers from Scopus and only the articles that dealt with research on the 20 most important PCs were selected for further analysis. The results showed that, in most areas, the USA and China are the two top countries in terms of conducting research on PCs; however, most studies on p-coumaric acid were conducted in India, followed by Spain and Italy. Moreover, only a few of the published papers pertained to neobavaisoflavone (34 documents published from 2000 to 2016), followed by China, Poland, and Cameroon. Spain gained the first place for research on syringic acid. presents the total number of documents published on different classes of PCs as indexed in Scopus for each country.
As depicted in , quercetin, catechin, kaempferol, resveratrol, apigenin, and luteolin may be the most-studied PCs in the world. Of the family of polyphenols, flavonoids received the most attention. Flavonoids are a major class of PCs that are distributed in nature and consumed as part of the human diet in considerable amounts. These compounds are recognized for their antioxidant properties, and their potential benefits have been largely studied. The search carried out in this study showed that quercetin is one of the most important flavonoids considered for research. A substantial body of evidence has accumulated over the last few decades on the pharmacokinetic profile of this compound. Data suggests that people following a diet rich in quercetin and other flavonoids are at a lower risk of many chronic diseases, such as cancer, diabetes, and heart disease.[Citation51,Citation52]
Why are PCs so highly esteemed?
There is a popular idiom saying “You Are What You Eat”; there is a close and positive relationship between a healthy diet and better health, and this fact has been documented in a number of studies.[Citation18,Citation53–Citation56] There are various reasons why there may be a relationship between the consumption of PCs and the reduced risk of HDs, including the modulation/regulation or inhibition of cell signaling pathways, the reduction of platelet aggregation, the effect on cholesterol synthesis, the effect on blood pressure and hormone metabolism, and antioxidant effects.[Citation57,Citation58] As noted in the previous sections, quercetin, kaempferol, catechins, resveratrol, rutin, and their derivatives are known for their health benefits in humans and the prevention of various HDs (especially cancer, cardiovascular diseases, diabetes, obesity, liver disorder, and infectious diseases).[Citation17,Citation27,Citation31,Citation38,Citation59–Citation66] Since fruits, especially apples, pears, apricots, cherries, berries, and grape, and vegetables, such as carrot, tomato, onion, garlic, cabbage, and celery, contain up to 200–300 mg of PCs per 100 g of their fresh weight, they can be used as dietary supplements of PCs.[Citation67] The presence of these compounds in food and pharmaceutical industries has improved the quality of food and drugs. Furthermore, these compounds have a low toxicity in the human body, and can therefore be considered a safe dietary element. Because of their antioxidant properties, these compounds play a critical role in the oxidative stability of foods. PCs are currently used in traditional and modern medicine in the design and development of new medicinal agents and for their extensive health benefits. The antimicrobial effect of PCs is another reason for regarding these compounds as major phytochemicals.[Citation68] Flavonoids have recently been used for the treatment of epilepsy.[Citation69] Epilepsy is the most common neurological disorder, affecting 1–2% of the population worldwide and significantly deteriorating many aspects of quality of life.[Citation70] Experimental studies have shown that, due to their structural similarity with benzodiazepines, flavonoids exhibit antiepileptic effects by modulating the GABAA-Cl-channel complex.[Citation71,Citation72] Overall, avoiding unhealthy diets and adhering to the consumption of flavonoid-rich foods can help people have a healthier body. PCs are beneficial nutrients that protect human health by fighting against the free radicals produced in the body cells and preventing oxidative damage. Further studies are required to identify flavonoids’ precise mode of action in the human body.
Link between the mechanism of action of PCs and human diseases
The important role of PCs in the treatment of HDs has been recognized in many studies and the use of polyphenol supplements has been examined too.[Citation52] Evidence suggests that PCs can modulate/regulate or inhibit many cell signaling pathways in the human body. Large bodies of studies have been published about the molecular mode of action of PCs. This paper summarizes the most relevant targets significantly affected by PCs. The search revealed many studies on the role of dietary PCs (in particular, flavonoids) in the prevention of inflammatory signaling pathways (e.g. the arachidonic acid pathway). PCs have been found to inhibit inflammatory enzymes such as PLA2, COX-1/2, and LOX.[Citation73] These compounds are mainly able to decrease cell proliferation, angiogenesis, and leukocyte immobilization and reduce 5-lipoxygenase enzyme activities. Tea polyphenols are able to regulate/modulate COX-2 enzyme activity and gene expression in different types of cancer cell.[Citation74] Quercetin also inhibits COX and LOX enzymes in mice models as the most-studied flavonoid[Citation75], and can thus reduce the formation of inflammatory metabolites.[Citation76] This compound is known for its iron-chelating/stabilizing potentials.[Citation52] Two key reports published by Hou et al. showed that pro-delphinidin B-4 3ʹ-O-gallate and pro-delphinidin B2 3,3ʹ di-O-gallate have inhibitory effects against the COX-2 enzyme and the release of PGE2 in LPS-induced murine macrophage RAW264 cells.[Citation77,Citation78] PCs can oxidize free radicals and also prevent injuries caused by free radicals through their direct radical scavenging capacity. PCs thus act as terminators for free radicals.[Citation14] These compounds also chelate metal ions[Citation79], and thus reduce their pro-oxidant activity.[Citation80]
A large body of evidence speculates that PCs have protective effects against age-related degenerative diseases.[Citation81] Animal studies and in vitro studies have generally reported the beneficial activities of PCs against HDs, but further explorations are still necessary to determine the accurate mode of action of PCs. The inconsistency between experimental studies and clinical studies may be partly due to the many technical problems with human studies. The review of literature showed a significant correlation between the consumption of PCs (especially flavonoids) and the prevention of HDs as per the finding of the experimental studies. Diets rich in fruits and vegetables with a consistently high consumption of PCs can help prevent many HDs. Schneider et al. speculated that diets poor in PCs are responsible for 7% and 10% of esophageal cancers in women and men in South Africa.[Citation82] Although many chemical analyses have been conducted on PCs, the mechanisms of action of these compounds in the human body are still not fully understood. Studies indicated that a series of PCs selectively accumulate in the epithelial cells of the upper digestive tract of rats with an ATP- and -dependent organic anion transport mechanism[Citation83], and because of the great reactivity of OH groups in PCs, radicals can be inactive as per the following equation[Citation14,Citation84]:
where is a free radical and
is a reactive oxygen species. Given the natural antioxidant activity of PCs, these compounds can be said to play a significant role during oxidative stress both in plants[Citation14] and in animals.[Citation85,Citation86] PCs, in particular luteolin, are reported to be potent inhibitors for xanthine oxidase in damaged tissues.[Citation87] Silibinin is also a flavonoid that can inhibit the formation of free radicals in chronic liver injury.[Citation88] The oral administration of a purified micronized flavonoid fraction was reported to decrease the number of immobilized leukocytes during reperfusion.[Citation89] Some studies showed that PCs can block the receptor for advanced glycation end products (RAGE) and its associated inflammatory cascade.[Citation90] Several studies have reported the expression of RAGE in some organs such as the kidney[Citation91], blood vessels[Citation92], and adipose tissues.[Citation93] PCs, in particular curcumin, may also interfere with inflammatory states and improve the physiological status in obesity (in a dose-dependent manner).[Citation94]
A rodent model study demonstrated the osteo-protective effects of green tea PCs by showing how they reduced oxidative stress, increased antioxidant enzyme activities, and reduced the expression of pro-inflammatory mediators.[Citation95] These compounds were reported to inhibit cell growth by inducing cell-cycle arrest/apoptosis and inhibiting cell proliferation and angiogenesis.[Citation96] Flavonoids, in particular, quercetin, have been reported to improve diabetic status.[Citation97] PCs can also inhibit glucose and fructose transport by the inhibition of the GLUT2 enzyme.[Citation98] PCs alone appear to not have a significant effect on the prevention of diseases in animal models. In a report by Jung et al., the benefits of quercetin and foods rich in quercetin were examined and compared in animal models and the finding showed that foods rich in quercetin have sharp effects on oral glucose tolerance in rats that show type 2 diabetes.[Citation99]
The administration of apigenin (4 mg/kg of body weight) to Streptozotocin or streptozocin-induced (STZ-induced) induced diabetic rats shows a significant anti-hyperglycemic effect.[Citation100] Dietary supplementation with genistein and daidzein (0.02% of the diet) has been shown to prevent the incidence of diabetes and improve glucose homeostasis in non-obese diabetic rats by preserving the pancreatic β-cell function.[Citation101] Dietary PCs are used as anti-obesity agents, due to their ability to inhibit the growth of adipose tissues, suppress the differentiation of preadipocytes, stimulate lipolysis, and induce apoptosis in exiting adipocytes, through adipose tissue size.[Citation102] Finally, PCs have a remarkable potential to regulate various molecular targets, including the transcription factors of NF-κB, STAT3, and PPARγ, growth factors, tumor necrosis factor (TNF), IL-1, IL-6 inflammatory cytokines, mTOR, MAPK, and Akt protein kinases and the related enzymes, and can also modulate other important signaling pathways related to Bcl-2, Bcl-XL, AP-1, epidermal growth factor receptor (EGFR), HER2, c-jun amino terminal kinase (JNK), Wnt/β-catenin, and caspases.[Citation103,Citation104]
In general, animal studies and epidemiological studies support the protective effects of many dietary PCs. These compounds exert their effects by targeting different cellular and molecular pathways in different parts of the human body (e.g. the liver, pancreas, heart, skeletal muscles, etc.). This section is a review of the literature on the most-studied PCs and seeks to present a comprehensive overview of the main reason for the significant interest in these compounds and discusses the major molecular signaling pathways regulated by these most-studied PCs.
Quercetin
Quercetin (3,3ʹ,4ʹ,5,7-pentahydroxyflavone) has been mentioned in 21,663 articles indexed in Scopus and is likely important due to its relatively high bioavailability[Citation105] compared with other PCs. This molecule is widely present in fruits, vegetables, and many dietary supplements and herbal remedies in the form of quercetin glycosides[Citation106] (). In most cases, one or more sugar groups (glucose molecules) are frequently bound to the 3-position of quercetin.[Citation105,Citation107,Citation108] Onion is one of the main sources of quercetin glycosides[Citation109,Citation110], and quercetin-4ʹ-O-β-D-glucoside (Q4ʹG) and quercetin-3,4ʹ-β-O-D-diglucoside (Q3,4ʹdiG) are solely found in onion.[Citation109,Citation111,Citation112]
The present study found that cancer, diabetes, and digestive diseases are the top three diseases treated with quercetin. Over the past 5 years, obesity has also become the target of treatments with quercetin in a dose-dependent manner. According to reports, a quercetin-rich diet has significant effects on obesity. This compound may become an optimal way for losing weight and fats in the near future. In and by itself, quercetin has no significant effects on HD, but it can be used as a dietary supplement for the treatment of obesity, diabetes, and other extreme HDs. As for the antibacterial effects of quercetin, this compound has recently received more attention for the treatment of oral diseases. According to the results obtained through cell cultures or animal models, quercetin is a good candidate for the development of plant-based drugs. The unavailability of data derived from human studies on this compound means that the use of quercetin as a drug needs further investigations and validation. This section of the present article discusses the most-studied molecular and cellular pathways that can be affected by quercetin.
Quercetin is a flavonoid that has been promoted to an excellent antioxidant in many studies[Citation113]and also possesses anti-inflammatory properties[Citation114], can prevent cardiovascular disease[Citation115], fights influenza A virus[Citation116], has antiulcer, antiallergy, and anti-proliferative effects[Citation117], and regulates gene expression.[Citation118] Quercetin also possesses key structural features for effective free radical scavenging, namely ortho-dihydroxy substitution in the B-ring (or the catechol structure) and enol moiety in the A-ring, which leads to the formation of an additional H-bond with the 4-keto group.[Citation98,Citation119] Numerous studies have shown that quercetin and its derivatives act as a powerful in vitro free radical scavenger.[Citation107,Citation120,Citation121] In general, these compounds prolong the lag time of copper-induced LDL oxidation in the following order: Quercetin-7-glucuronide > quercetin > quercetin-3-glucuronide = quercetin-3-glucoside > catechin > quercetin-4ʹ-glucurnoide > isorhamentin-3-glucuronide > quercetin-3ʹ-sulfate.[Citation122] Nonetheless, its in vivo direct scavenging of common small free radicals such as ,
, and
, produced by cell metabolic processes, and the endogenous and exogenous impacts and its biological relevance are yet to be established.[Citation107]
The daily intake of quercetin is estimated to be around 5–40 mg/day[Citation123], and these levels can increase to 200–500 mg/day with the consumption of high quantities of fruits and vegetables rich in flavonoids.[Citation124] The maximum concentration of quercetin in the human plasma after the ingestion of 500 mg of quercetin was approximately 1–8 ng/ml.[Citation125] shows the possible pathway of the metabolic conversion of quercetin during intestinal absorption and inflammation.
According to studies, the factors affecting quercetin absorption include: 1) the nature of the attached sugar[Citation126], and 2) the chemical properties of the body cells.[Citation127] Studies on quercetin suggest that the absorption of quercetin aglycone differs from the absorption of quercetin glycosides.[Citation111] For example, in rats, quercetin aglycone is partly absorbed through the stomach, whereas quercetin glycosides (e.g. isoquercetin and rutin) are not.[Citation61] Several studies have shown that quercetin glucosides are efficiently hydrolyzed in the small intestine by beta-glucosidases into the aglycone form, much of which is then absorbed.[Citation43,Citation128,Citation129] Meng et al.[Citation130] concluded that the aglycone form of quercetin appears to be better absorbed than the glycoside forms upon the consumption of grape juice. Once absorbed by the small intestine, quercetin is converted to glucuronide or sulfate conjugates and only conjugated metabolites are found in the blood stream afterwards.[Citation111]
Several in vitro and in vivo animal studies have shown that quercetin can be considered an option for the treatment of cancer by inducing cell death or cell-cycle arrest in the cancer cells.[Citation58,Citation124,Citation131]Many studies have also reported that this compound contributes to the prevention of cardiovascular diseases.[Citation115,Citation132] The increased incidence of cardiovascular diseases such as atherosclerosis, hypertension, stroke, ischemic heart diseases, and heart failure can lead to an expected global rate of cardiovascular-related deaths, which will exceed 23.6 million by 2030.[Citation133] According to the review of literature, this compound has been shown by researchers to be a potent inhibitor of EGFR tyrosine kinase pathway, which plays a key role in cell proliferation.[Citation134,Citation135] Basically, quercetin may inhibit cell migration and invasion through the deactivation of MMP-2 and/or MMP-9.[Citation136] Aryl hydrocarbon receptor (AhR) is another candidate target for the chemopreventive effects of quercetin. This receptor regulates the expression of the CYP1 family (CYPIA1 and CYP1A2) and CYP1B1, which can induce pro-carcinogens.[Citation137] When quercetin ( 1.5 µM) binds to AhR, bothCYP1 and B1 become incapable of the biotransformation of polycyclic aromatic hydrocarbons to carcinogenic metabolites.[Citation111]
Chen et al.[Citation138] reported that quercetin increases the expression of DR5 in non-small lung cancer cells and causes the repartition of DR4 and DR5 into lipid rafts in colon adenocarcinoma cells by the simulation of tumor necrosis factor-related apoptosis-inducing ligand (TRAIL).[Citation139] Lipid rafts are located in the external leaflet of the plasma membrane and consist of tightly packed domains of cholesterol and saturated sphingomyelin that float in melted and loosely packed unsaturated lipids.[Citation140,Citation141] presents a potential mechanism of quercetin action in the prevention of cancer.
Other possible inhibitory mechanisms of quercetin for the prevention of cancer have been reviewed in detail by Kelly[Citation98], Lamson and Brignall[Citation142], and Lee et al.[Citation143] The cardioprotective activity of quercetin in patients is one of the most popular subjects of research into this compound.[Citation144] The role of this compound in preventing cardiovascular diseases[Citation145] has been largely associated with its anti-inflammatory and antioxidant properties[Citation146] (). Quercetin also appears to be effective in improving blood pressure and might have an effect on cholesterol levels in humans.[Citation147]
Evidence suggests that quercetin is equally or even more effective than trans-resveratrol in attenuating TNF-α-mediated inflammation and insulin resistance in primary human adipocytes.[Citation148]
Many studies speculated that quercetin might show a significant role in the regulation of the blood lipid profile.[Citation149] The consumption of quercetin-rich foods can be said to significantly decrease the serum concentrations of glucose and total cholesterol (TC) and increase the serum concentrations of high-density lipoprotein (HDL).[Citation150] In animal models, quercetin is effective in preventing the total loss of β-cell mass[Citation51] and may ameliorate the symptoms of streptozotocin-induced diabetes by decreasing oxidative stress.[Citation151] Quercetin has also been shown to have a major role in the protection of the cardiovascular system from the effects of aging.[Citation18] This compound can reduce the risk of cataract formation, which is the most common age-related eye condition[Citation152], through affecting multiple pathways related to eye lens opacification, including oxidative stress[Citation153], nonenzymatic glycation[Citation154], the polyol pathway, calpain proteases, and epithelial cell signaling.[Citation155,Citation156]
Several critical points must be taken into account when considering the potential therapeutic effects of quercetin including (1) the pharmacological versus the nutraceutical doses used, (2) the specificity of its mechanism of action compared with other phytochemicals, and (3) the identification of direct cellular targets.[Citation124,Citation131] As discussed, due to its significant role in human health, quercetin has received more attention from clinical studies in recent years. Given the multiple benefits of quercetin, this compound is available in the market in various forms and dosages for the treatment of lifestyle-related diseases in humans[Citation157] (). Several studies have reported quercetin as the most favorable flavonoid for drug-based studies compared with other PCs[Citation158] because of its favorable biological activities.[Citation159]
Rutin
Rutin is another most studied polyphenol[Citation62] that is the main glycoside (3-O-rhamnoglucoside) form of quercetin, and is the most abundant flavonol in vegetables and fruits.[Citation160] This compound is an important flavonoid that is consumed as part of people’s daily diet.[Citation161] It is also known as vitamin P and quercetin-3-O-rutinoside.[Citation52] Rutin has been studied extensively for its various pharmacokinetic parameters using different animal as well as volunteer human models.[Citation161] Given the multiple benefits of rutin, this compound is available in the market in various forms and dosages for the treatment of lifestyle-related diseases in humans ().
Rutin is deemed to have some health benefits. The review of the literature showed that this compound has a significant antioxidant activity. Cancer, hypertension, and hypercholesterolemia are the three top chronic diseases that can be treated with rutin. Rutin is a stable polyphenol and shows low adverse effects for animal cells. The anti-obesity activity of this compound has been reported in a large body of literature over the past few decades. In a clinical study conducted among 50 diabetic patients over a period of 120 days, evidence suggested that rutin can increase HDL, very low-density lipoprotein (VLDL), triglycerides (TGL), and TC levels and decrease LDL.[Citation162] This and similar studies have shown that this compound has a significant role in the treatment of obesity and diabetes. The review of the published papers containing the keyword “rutin” showed that selection of this compound as a drug is a prerequisite to improving its bioavailability through novel approaches.[Citation62,Citation163,Citation164]
Hollman et al.[Citation61]reported that quercetin glucosides are more efficiently absorbed than quercetin alone, whereas rutin is less efficiently and less rapidly absorbed. The optimal dose of rutin that can act as an antioxidant is 1000 mg/day.[Citation165] As a capillary and blood vessel protectant, the dose range of this compound is 400–1000 mg/day.[Citation166] The bioavailability of rutin is ~20% as that of quercetin glucosides.[Citation167] The main reason for the poor bioavailability of rutin is its poor aqueous solubility.[Citation160] To improve the bioavailability of quercetin and rutin, numerous approaches have been undertaken, involving the use of promising drug delivery systems such as inclusion complexes, liposomes, nanoparticles, or micelles, which appear to provide a higher solubility and bioavailability.[Citation168–Citation170] Gallic acid and isoflavones are shown to be the most well-absorbed PCs, followed by catechins, flavanones, and quercetin glucosides, which have different kinetics.[Citation167]
Many studies have shown that rutin is a powerful antioxidant against free radicals and this capacity is concentration-dependent[Citation160,Citation171], and the antioxidant activity of flavonoids increases with their concentration.[Citation86] At a concentration of 50 mg/kg, rutin decreases the glucose levels by increasing insulin secretion in hyperglycemic rats and thus affects uptake in rat pancreatic islets by increasing the
influx via the L-type voltage-dependent calcium channel.[Citation172] Other studies have also shown the protective effects of rutin on various targets such as the heart[Citation173], lung[Citation174], pancreas[Citation175], kidney[Citation176], skeletal muscles[Citation177], and sciatic nerve.[Citation174]
Catechins
Catechins ((2R,3S)-2-(3,4-dihydroxyphenyl)-3,4-dihydro-2H-chromene-3,5,7-triol) are another group of PCs generally found in the leaves of Camellia sinensis.[Citation178–Citation180] In addition to green tea, apples, grapes, pears, and cherries are some of the main sources of catechins[Citation181] (). About 10% of the dry weight of green tea leaves consists of catechins, including 50% (-)-epigallocatechingallate (EGCG), 19–30% (-)-epigallocatechin (EGC), 10–13% (-)-epicatechingallate (ECG), and 6–10% EC.[Citation19,Citation179,Citation180] EGCG is the most dominant catechin form in green tea.[Citation180] The daily intake of catechin has been estimated to be 18–50 mg/day.[Citation167] Although catechins are not necessary to human nutrition, they help in improving human health by preventing various diseases.[Citation182]
According to both epidemiological and experimental studies, cancer, cardiovascular diseases, and aging are the three top illnesses treated with catechins. Similar to other PCs, these compounds have a great potential for antioxidant activity. Although in vitro studies have well established the antioxidant activity of these compounds, clinical studies seem to be limited on these compounds. Nonetheless, the review of the literature showed that catechins can help eliminate a wide range of diseases in animal models. This section of the article introduces some of the most popular effects of catechins.
In vitro studies have shown that catechins have protective effects against degenerative diseases[Citation182] and there is a strong inverse correlation between the intake of catechins and the risk of mortality due to coronary heart disease.[Citation183] Catechins appear to have greater antibacterial activities against Gram-positive than Gram-negative bacteria.[Citation68,Citation184,Citation185] Evidence is emerging that catechins may be useful in the control of common oral infections, such as dental caries and periodontal diseases.[Citation68,Citation186] Kivits et al.[Citation187] reported that the catechins in green and black tea are rapidly absorbed. In another key study, Zhu et al.[Citation188] observed the low systemic availability of tea catechins and attributed it to a slow absorption, high first-pass effect, and wide tissue distribution. Tea catechins are capable of binding to a variety of enzymes and inhibiting their activities.[Citation180] The inhibition of enzyme activity could be due to either direct binding or indirect actions.[Citation189] For example, EGCG (at a concentration of 5–20 µM) can inhibit the phosphorylation of JNK, c-Jun, MEK1/2, ERK1/2, and ELK1.[Citation190]
Dihydrofolatereductase, glucose-6-phosphate dehydrogenase, glyceraldehyde-3-phosphate dehydrogenase, and carbonyl reductase 1 are the other enzymes inhibited by EGCG.[Citation191–Citation194] Studies have shown that EGCG changes the lipid organization in the plasma membrane, thus affecting the protein distribution; these behaviors are likely associated with the inhibition of EGFR[Citation195], hepatocyte growth factor receptor (HGFR)[Citation196], and 67LR[Citation197] functions. In animal models, EGCG decreases liver damage, liver inflammation, liver triglyceride, TC, and inflammatory cytokines (e.g. MCP-1 and CRP/IL-6).[Citation198] The activity of NF-κB and C/EBP-α transcription factors regulates the expression of iNOS and COX-2.[Citation199,Citation200] The consequences of the high levels of iNOS and COX-2 include the overproduction of NO and eicosanoids through the initiation of the COX-prostanoid pathway[Citation201], which causes cellular inflammation and necrosis.[Citation202] Treatment with EGCG leads to a reduction in the expression of both iNOS and COX-2, thereby increasing the anti-inflammatory effects of EGCG in the liver cells.[Citation203]
Evidence suggests that EGCG modifies DNA methylation and its effects on histone modifiers.[Citation204] EGCG interferes with the enzymatic activities of HAT, HDAC, and DNMT.[Citation205] Fang et al. found that EGCG acts as a competitive inhibitor of DNA methyl transferase (Ki = 6.89 µM), which binds to the catalytic pocket and inhibits DNA methyl transferase activity in a dependent manner.[Citation206] summarizes the potential epigenetic effects of catechins.
EGCG and other tea catechins appear to be very effective scavengers of ROS and RNS both in vitro and in vivo.[Citation179,Citation208] Animal model studies have shown that EGCG decreases lipid peroxidation and protein carbonylation in old rats, but not in young rats.[Citation209] Moreover, albumin has a synergistic increasing effect on the antioxidant activity of green tea catechins in oil-in-water emulsions.[Citation210] The antioxidant activity of catechin in emulsions containing BSA follows the pattern ECG ≈ EGCG > EC > EGC.[Citation122,Citation210] Recent studies have shown that catechins have a relatively high binding affinity to human serum albumin (HAS). Catechins with a binding constant to HAS include: EGCG GCG (gallocatechingallate) > ECG ≫ EC
EGC
catechin.[Citation122,Citation211,Citation212] EGCG has also been reported to have an inhibitory effect on Acetyl-CoA carboxylase, which is essential for the in vitro biosynthesis of fatty acid[Citation213] and has anti-obesity effects in rats at high doses.[Citation214–Citation216]Given the multiple benefits of catechins, these compounds are available in the market in various forms and dosages. The evaluation of catechins as metabolic regulators pave the way for the development of new drugs.[Citation217]
Kaempferol
Kaempferol (3,5,7-trihydroxy-2-(4-hydroxyphenyl) chromen-4-one) is another class of PCs found in fruits and vegetables[Citation65] (). This compound has often been associated with the quality of grapes and wines and is considered an important source of antioxidant in proportion to the dietary requirements.[Citation218] The most common flavonols found in foods include quercetin and kaempferol.[Citation63] The present finding showed that kaempferol is one of the most-studied PCs.
Similar to other PCs, kaempferol has a great potential for the prevention of HDs. The findings of this study suggest that cancer, diabetes, neurodegenerative diseases, and obesity are the most common diseases treated with this compound. Kaempferol and most other dietary PCs show a common property by which they reduce HDs. The consumption of sources rich in PCs (e.g. vegetables and fruits) can help promote public health. The consumers of PCs should bear in mind that these compounds are able to increase the risks of certain other diseases and researchers must further examine this duality. This section of the article focuses on the most common molecular mechanisms of kaempferol.
The health benefits of dietary kaempferol are well documented in reducing the risk of chronic diseases, especially cancer.[Citation219,Citation220] The total average intake of kaempferol in humans is 23–25 mg/day[Citation64,Citation221] and kaempferol-3-glucuronide is the major compound detected in blood plasma and urine.[Citation222] The bioavailability of kaempferol varies depending on factors such as chemical structure, lipophilicity, molecular weight, gut microbial metabolism, food matrix, and possibly other components of the diet.[Citation222]
As noted, kaempferol is a good target for cancer therapy.[Citation223] It is evidently able to scavenge free radicals and modulate several signaling pathways that ultimately lead to cancer cell apoptosis.[Citation59] The potential mechanisms of chemoprevention by kaempferol include the inhibition of carcinogen-activating genes and enzymes and enhancing carcinogen detoxification by getting phase II detoxifying genes and enzymes involved.[Citation221] Kaempferol (at 10 μM concentration) induces significant anti-viability effects in pancreatic cancer cells through apoptosis.[Citation224] Studies have reported that kaempferol strongly inhibits the activation of the EGFR/p38 signaling pathways, the up-regulation of p21 expression, and the down-regulation of cyclin B1 expression in cancer cells, thus leading to the activation of PARP cleavage, the induction of apoptotic death, and the inhibition of cell growth.[Citation225,Citation226] P38 is an MAPK that can regulate cell proliferation while EGFR can mediate p38 activation.[Citation227,Citation228] Studies have also examined the potential link between the consumption of foods containing kaempferol and the reduction of the risk of developing disorders such as cardiovascular diseases[Citation229], obesity[Citation230,Citation231], inflammation[Citation232], and type 2 diabetes.[Citation230,Citation233] Kaempferol and its glycosides were reported to reduce the accumulation of adipose tissue and improve hyperlipidemia and diabetes in obese mice by increasing lipid metabolism through the down-regulation of PPARγ and SREBP-1c.[Citation230,Citation234] Berger et al. reported that a 50 µM concentration of kaempferol has a distinct epigenetic activity by inhibiting HDACs.[Citation235] This compound is able to induce apoptosis by decreasing the expression of Bcl-2 and increasing the expression of Bax.[Citation236] At a 70 µM concentration, kaempferol has a crucial role in the activation of caspase-3 and 9 cascades.[Citation237] Overall, the epidemiological data on this compound suggest that a high intake of kaempferol-rich foods may reduce the risk of developing several types of cancers (e.g. lung, gastric, pancreatic, and ovarian cancer) and cardiovascular diseases.[Citation65,Citation238] Many studies have shown that kaempferol and its glycosides are the most popular for their antibacterial properties.[Citation239,Citation240] The antiviral activity of this compound against the influenza virus[Citation241], herpes simplex virus[Citation242], and HIV[Citation243,Citation244] has been documented in the literature. Given its ability to inhibit LDL oxidation, kaempferol can be said to play a critical role in the prevention of atherosclerosis.[Citation245] Moreover, the anti-inflammatory activity of kaempferol is caused by its significant contribution to the inhibition of both inflammatory cell function and the expression of pro-inflammatory cytokines and chemokines.[Citation246,Citation247] Further studies are required for evaluating the benefits of kaempferol for clinical trials and the treatment of HDs.[Citation225,Citation248]
Resveratrol
5-[(E)-2-(4-hydroxyphenyl)ethenyl]benzene-1,3-diol (resveratrol) is a dietary polyphenol, belonging to the stilbenes group and naturally occurring in fruits and food products[Citation249–Citation251]in response to injury, fungal attack, or other environmental stress.[Citation252,Citation253] presents the chemical structure and major sources of reseveratrol. This compound was first isolated from the roots of Veratrum grandiflorum O. Loes in 1940, and later from the roots of Polygonum cuspidatum.[Citation36] As a dietary supplement, it is a powerful antioxidant[Citation254] with benefits for muscle strength[Citation255], the treatment of inflammation[Citation256], the regulation of body metabolism[Citation257], and the treatment of neurodegenerative diseases[Citation258], diabetes[Citation259], cardiovascular diseases[Citation260], and cancer.[Citation261] Many studies assume that this compound has a wide range of biological activities. Due to its abundance in nuts, grapes, and grape products (in particular red wine), this compound is one of the main targets for food and pharmaceutical industries. Similar to the other PCs, resveratrol has aromatic groups in its structure and thus exhibits antioxidant activities. Researchers have recently come to emphasize its anti-obesity potential. The present study found that this compound can be used for the treatment of certain types of cancer, such as pancreatic, colorectal, lymphoma, breast and prostate cancer, and leukemia. The anti-UV activity of this compound has also received more attention than other PCs in recent years.[Citation262] Although resveratrol shows significant anticancer properties, it also increases the risk of cardiovascular disorders in rats.[Citation263] Regardless of these benefits, it is important to also note the minimal side effects of PCs and administering their best doses for consumption. This section of the article introduces the health benefits and the molecular mechanism of action of resveratrol.
This compound exists as cis and trans forms, with the trans-isomeric form being stable at −20°C for over five days and at 4°C for up to two days when protected from exposure to light.[Citation264,Citation265] Similar to other PCs, resveratrol shows a high absorption, a low bioavailability, and a rapid clearance from the blood stream.[Citation266,Citation267] Based on in vitro studies, this compound can inhibit cell proliferation[Citation268], induce apoptosis[Citation269], and block cell-cycle progression[Citation270] in various types of human cancer cell lines, such as colon, skin, breast, lung, prostate, liver, and pancreatic cancer cell lines.[Citation266] As depicted in , resveratrol can be said to have two aromatic groups; these rings can act as antioxidants by forming stable radicals via resonance structures, thereby inhibiting continued oxidation. In vitro antioxidant assays such as DPPH, ABTS, DMPD, and H2O2 and their scavenging activities have shown that resveratrol has higher radical scavenging capacity compared to vitamin C, vitamin E, and propyl gallate.[Citation85] Several studies have attributed the anticancer activity of resveratrol to its ability to inhibit COX-1 activity[Citation271] and the prevention of the PI3K/Akt signaling pathway.[Citation272]
Resveratrol has a significant role in decreasing obesity and its associated abnormalities.[Citation253,Citation273]Animal studies have reported that this compound significantly regulates signaling molecules such as GalR1/2, PKC, Cyc-D, E2F1, and p-ERK and key adipogenic genes (e.g. PPARγ2, C/EBPα, SREBP-1c, FAS, LPL, aP2, and leptin) in the epididymal adipose tissues of mice.[Citation273] More recently, resveratrol has been reported to improve glycemic control in animals and humans with insulin resistance or diabetes.[Citation274,Citation275] Resveratrol also inhibits TNF, IL-1β, NO, and NF-κB in peritoneal macrophages.[Citation276] This compound also shows a dose-dependent antiestrogenic activity similar to genistein and quercetin.[Citation28] Sharma et al. reported that resveratrol (at a 20 µM concentration) inhibits the release of cytokines by peritoneal macrophages and stimulates IL-10 expression.[Citation277] The inhibitory activity of resveratrol against iNOS is also well documented in the literature.[Citation278] Given the health benefits of resveratrol for hypertension, obesity, inflammation, diabetes, and dyslipidemia, this compound can offer an interesting pharmacological approach for the treatment of the metabolic syndrome, which is associated with an increased risk of cardiovascular diseases.[Citation279] The main part of the dietary intake of resveratrol is in the trans-resveratrol form.[Citation265] Modifying the stability, chemical structure, and metabolism of resveratrol could change its cellular and molecular targets and thereby improve or decrease its chemopreventive properties.[Citation265] The aqueous solubility of resveratrol has been reported as <1 mg/ml, which is a major drawback of the drug.[Citation36,Citation280] Resveratrol stability appears to involve redox reactions and biotransformation, which influence its antioxidant properties.[Citation265] As noted, resveratrol was reported to act as an inhibitor of carcinogenesis.[Citation281] presents a list of the mechanism of actions proposed for resveratrol.
Many studies have focused on in vitro and in vivo animal studies, with very few trials being conducted on human subjects.[Citation36,Citation282] Nonetheless, the emerging popularity of resveratrol and its potential to improve health and quality of life have led to a market rich in resveratrol supplements and an interest from pharmaceutical companies for developing resveratrol-based drugs.[Citation283]According to the data obtained, this compound shows an inhibitory effect on abnormal cells acting on MAPK, and NF-κB as well as PPAR-γ, although the final outcome depends on the situation and doses administered.
Frequency of research areas
As depicted in , most of the studies on PCs can be categorized into six research areas including (1) Biochemistry, (2) Medicine, (3) Agriculture, (4) Pharmacology, (5) Chemistry, and (6) Chemical engineering. From 2000 to 2016, flavonoids have received more attention from these areas of research. These studies have been conducted by more than a thousand researchers throughout the world and further studies are required on PCs in order to achieve a better understanding of how the expression of PCs can be increased in plants[Citation284,Citation285] and how these compounds can help protect the human body against certain disorders.[Citation286] PCs have a lot more uncovered details[Citation287] and researchers should try to find the physiological role of these compounds in plants[Citation14] and their mechanism of action in the human body.[Citation286]
Polyphenols and the treatment of chronic diseases in humans
The use of PCs for the treatment of HDs was also studied via articles indexed in Scopus. presents the total number of documents published on PCs for various HDs by country. The contribution of PCs to the treatment of HDs in different countries showed that, in most fields, the USA, India, and China are at the top of the list, occupying three of the top 24 places. South Korea is another country ranked second for cancer, in fifth for heart disease, third for anti-infectious diseases, sixth for liver disorders, fourth for inflammation, fifth for viral diseases, fifth for Alzheimer’s, fourth for Parkinson’s disease, second for Obesity, tenth for hypertension, and fifth for diabetes.
According to these rankings, the UK is the first in the top 24 countries for the use of PCs in the treatment of liver disorders. India, Brazil, and South Korea also occupy three of the top 24 places for the use of PCs to treat infectious diseases. Russia and Belgium are two countries placed toward the bottom of the list in all the research areas. As shown in , a hierarchical cluster analysis was performed using Ward’s method[Citation40] to generate a dendrogram for estimating the number of likely clusters within the studied diseases by country (). Cluster analysis is an explorative method of analysis that seeks to identify structures within a set of data.[Citation288,Citation289]
As shown in this figure, there are two groups of countries that are very close to each other, with the first group consisting of Belgium, Russia, Poland, Canada, Nigeria, Pakistan, Iran, Malaysia, Turkey, Egypt, Portugal, and Australia, and the second group consisting of Italy, Japan, Germany, Spain, France, Taiwan, and Brazil. India and the UK, however, are not categorized into any of these two groups and are separated from the entire world in this area. The USA, South Korea, and China are also largely similar and form a third group. Research on PCs in different countries is thus very wide ranging in terms of HDs. Regarding the potential health benefits of dietary PCs[Citation60], these compounds can be said to be good candidates for the treatment of HDs.
Popular journals
Peer-reviewed journals play a crucial role in scientific advancement, and articles published in these journals are likely to remain a very important means of distributing research findings for use in the near future.[Citation290,Citation291]The journals contributing the most to research on PCs are identified and ranked based on the number of papers they have published over a recent 16-year period in four top areas of research ().
The ranking obtained for the seven top journals contributing to research on PCs showed a series of different journals. The Journal of Agriculture and Food Chemistry (J.Agric.FoodChem.) occupies the first of seven places for covering research on polyphenols (1720 published papers), flavonoids (2343 published papers), and stilbenes (290 published papers), and dropping three places for covering research on lignans (148 published papers). Planta Medica occupies the first of seven places for covering research on lignans (191 published papers). Food Chemistry (Food Chem) is another journal that covers studies on PCs, covering 1348 papers on polyphenols, 1845 on flavonoids, and 104 on stilbenes from 2000 to 2016. Journal of Natural Product (J.Nat.Prod.) specializes in research on lignans (173 published papers). Phytochemistry (Phytochem) is another journal that covers both flavonoids (594 published papers) and lignans (154 published papers). This ranking shows a significant correlation between research on PCs and peer-reviewed journals. Better studies on PCs should be published in peer-reviewed journals to reflect the overall quality of research into this area and the efficiency of journals for covering the research. Researchers can use this ranking for selecting a good journal for publishing their papers on PCs.
Basic challenges of research into polyphenols
As already noted, PCs, in particular, flavonoids, are present in the highest concentration in the human diet. Genistein, quercetin, kaempferol, daidzein, lutelin, apigenin, myricetin, and biochanin A are the most abundant phytochemicals in human diet.[Citation292] Although these compounds are naturally found in plants, the total concentration of PCs in the human diet might vary significantly. For example, the concentration of quercetin in the edible sections of fruits and vegetables is below 10 mg/kg.[Citation293] This amount is 5–17 mg/kg[Citation292] for kaempferol. Since different methods are used for the preparation and processing of foods, it might be that these methods decrease the concentration of PCs in foods. Cooking, frying, and freezing have remarkable effects on the total concentration of PCs in plant-based foods. The mere consumption of polyphenol-rich foods is not enough and several factors, such as glucuronidation, sulfation, methylation, and metabolization to other phenolic compounds (PCs), can change the bioavailability of PCs in the human body.[Citation27] Food industries should therefore pay further attention to the use of modern approaches for preparing polyphenol-rich foods and the optimal doses of these compounds used in the diet. Various in vitro and in vivo studies have reported that PCs are the best therapeutic target for the market; however, there are several problems with the use of these compounds.[Citation294] One of the most important questions to ask oneself is the dose of PCs that should be used in order to achieve their health benefits[Citation275], and whether or not PCs have side-effects in humans. The answer to these questions can facilitate further research into PCs. For example, quercetin may be a good compound for treating various diseases, but it also reduces the life-span of mice when added to their long-term diet.[Citation295] presents the basic challenges of research into PCs. The use of molecular modeling to predict interactions, large-scale screens for toxic or other common effects, affinity-based methods to identify drug-interacting proteins, and a better synthesis of the existing data, including legislation to promote the release of clinical trial results and tracking voluntary supplement consumption, are major factors that must be considered to improve basic research on PCs.[Citation275]
Understanding polyphenol interactions with other food ingredients/elements, such as proteins or dietary fibers, is important because complex reactions can take place during food processing.[Citation296]Many studies have shown that PCs have health benefits for humans[Citation16–Citation18,Citation20,Citation21,Citation28,Citation43,Citation179,Citation205,Citation206,Citation296–Citation298], and ensuring that their optimal doses are used in a diet is crucial.[Citation294] There is a great paradox about PCs; although these compounds are considered potent antioxidants, they also show a pro-oxidant (oxygen radical generating) behavior, which is cause for remarkable concern about the consumption of these phytochemicals.
In some sources, PCs have been found to exhibit antioxidant properties in vitro[Citation26,Citation85,Citation105,Citation120,Citation142,Citation254]; however, the in vivo concentrations of these compounds are not high enough to show any antioxidant activities.[Citation299] PCs concentrations in the gastrointestinal tract are high enough to act as pro-oxidants for the in vivo generation of ROS.[Citation300] Professor Barry Halliwell labeled PCs as “pro-oxidants” and speculated that the overconsumption of these compounds can down-regulate endogenous antioxidants.[Citation301]
Duarte and Lunec reported that vitamin C is also a potent antioxidant that can as well become a pro-oxidant. When combined with iron and copper, it can reduce Fe3+ to Fe2+ and Cu3+ to Cu2+, and in turn reduce hydrogen peroxide to hydroxyl radicals.[Citation302] Yordi et al. reported that, under certain conditions, PCs can behave as pro-oxidants.[Citation303] Dietary PCs can also act as pro-oxidants in systems that contain redox-active metals.[Citation304] The presence of O2 and transition metals such as iron and copper catalyzes the redox cycling of PCs and may lead to the formation of ROS and phenoxyl radicals that damage DNA, lipids, and other biological molecules.[Citation305]In some degenerative diseases, such as cancer, Alzheimer’s disease, diabetes (especially type II), and heart disease, ROS are produced in large amounts; in patients with polyphenol-rich diets, the potential pro-oxidant activity of PCs must be considered and a good diet with set doses of PCs can be used to prevent the advancement of the disease in early stages. The pharmacological potential of PCs is so diverse and complex that further studies are necessary in this area in order to increase the knowledge of PCs and their biological activities and health benefits for humans.
Future of polyphenols
Although there is a wide range of studies on PCs, it seems that the present knowledge about these compounds is at the lowest level possible and further studies with an emphasis on in vivo approaches are required to determine the health benefits of these compounds for humans and to develop a better understanding of their biological activities. Genomic and proteomic studies are required to find the exact role of these phytochemicals in the prevention of serious HDs.
Phytochemical screening will be essential for identifying the most favorable PCs both for market consumption and for clinical usage. High-throughput, standardized, chemical assays (e.g. chromatography, Gas chromatography–mass spectrometry (GC-MS), Capillary Electrophoresis - Mass Spectrometry (CE-MS), and novel gene profiling) are essential for the development of drugs containing PCs and their commercialization.[Citation299]
Bioinformatics and systems biology approaches are helpful modern tools used to evaluate a large body of data associated with these phytochemicals.[Citation4,Citation306] Methods such as molecular docking, virtual screening, and molecular dynamics simulation, meanwhile, can provide a critical basis for understanding the complex behavior of these compounds in combination with animal enzymes.
To conclude, the characterization of their biological pathways is an important piece of the puzzle about polyphenols in many studies examining these compounds. The use of phytochemicals, especially PCs, for the prevention and treatment of disastrous HDs is a popular strategy and the medicinal efficacy of many PCs as an antibacterial, anti-inflammatory, anticancer, and anti-obesity is well documented in the literature on the subject. Nonetheless, the therapeutic use of PCs must be validated through the use of specific biochemical assays. Further investigations of PCs will be necessary for achieving newer insights about these compounds and entering a new era of polyphenol-based drugs use for treating pernicious infectious and degenerative diseases.
Concluding remarks
As secondary plant metabolites, PCs are the most abundant class of substances with at least 10,000 different compounds that have become a focus of research over the past 16 years. These compounds are basically nontoxic and efficient phytochemicals with a diverse range of beneficial biological activities reaching from plants to humans. According to the present finding, all PCs have a common molecular mechanism of action and are able to inhibit cell proliferation and angiogenesis, down-regulate endogenous antioxidants, modulate transcription factors and their associated kinases, and prevent many signaling pathways. Regarding their molecular targets, a general molecular target was observed in this study for these compounds (). Although does not cover all of their molecular effects, it provides a comprehensive overview about their effects. Clinical studies are required on the concentration, bioavailability, efficacy, safety, and mechanism of action of PCs for therapeutic purposes. The absorption, distribution, metabolism, excretion, and biological activity of PCs in the human body must be further examined through research. The use of advanced and modern methods (e.g. nanotechnology) to increase the bioavailability of PCs and enhance their stability in the human body is actively growing. The results of this review study along with the remarkable body of literature about polyphenols demonstrate the therapeutic benefits of these compounds and suggest that PCs, in particular quercetin, rutin, kaempferol, catechins, and resveratrol, are able to prevent lipid overproduction, reduce the incidence of cancer, normalize insulin sensitivity, decrease tissue inflammation, and reduce the incidence of liver disorders and infectious diseases. Overall, PCs are the best targets of research into secondary metabolites, although their study poses certain challenges. Further agronomical, biochemical, and chemical studies should be conducted to elucidate the role of these compounds reaching from plants to humans. A long-term study of these compounds to investigate the direct physiological role of PCs in the human body is strongly recommended.
Acknowledgments
Hereby, the authors would like to express their gratitude to the Research Council of Kermanshah University of Medical Sciences (KUMS) for their financial support of this study (Grant No. 96300). The instructive comments and invaluable help provided by the anonymous reviewers are also greatly appreciated. The authors certify that no actual or potential conflict of interest in relation to this article exists.
References
- Wegener, G. ‘Let Food Be Thy Medicine, and Medicine Be Thy Food’: Hippocrates Revisited. Acta Neuropsychiatr. 2014, 26(01), 1–3. 10.1017/neu.2014.3.
- Clydesdale, F. M.; Francis, F. J. Food Nutrition and Health; Springer Science & Business Media, Netherlands: 1985.
- Bøhn, S. K.; Croft, K. D.; Burrows, S.; Puddey, I. B.; Mulder, T. P.; Fuchs, D.; Woodman, R. J.; Hodgson, J. M. Effects of Black Tea on Body Composition and Metabolic Outcomes Related to Cardiovascular Disease Risk: A Randomized Controlled Trial. Food Funct. 2014, 5(7), 1613–1620. 10.1039/C4FO00209A.
- Rasouli, H.; Hosseini-Ghazvini, S. M.-B.; Adibi, H. Differential Α-Amylase/Α-Glucosidase Inhibitory Activities of Plant-Derived Phenolic Compounds: A Virtual Screening Perspective for the Treatment of Obesity and Diabetes. Food Funct. 2017, 8, 1942–1954. 10.1039/C7FO00220C.
- Akbari, M.; Rasouli, H.; Bahdor, T. Physiological and Pharmaceutical Effect of Fenugreek: A Review. IOSR J. Pharm. 2012, 2(4), 49–53. 10.9790/3013-24204953.
- Valdés, L.; Cuervo, A.; Salazar, N.; Ruas-Madiedo, P.; Gueimonde, M.; González, S. The Relationship between Phenolic Compounds from Diet and Microbiota: Impact on Human Health. Food Funct. 2015, 6(8), 2424–2439. 10.1039/C5FO00322A.
- Oh, -M.-M.; Trick, H. N.; Rajashekar, C. Secondary Metabolism and Antioxidants are Involved in Environmental Adaptation and Stress Tolerance in Lettuce. J. Plant Physiol. 2009, 166(2), 180–191. 10.1016/j.jplph.2008.04.015.
- Yue, W.; Ming, Q.-L.; Lin, B.; Rahman, K.; Zheng, C.-J.; Han, T.; Qin, L.-P. Medicinal Plant Cell Suspension Cultures: Pharmaceutical Applications and High-Yielding Strategies for the Desired Secondary Metabolites. Crit. Rev. Biotechnol. 2016, 36(2), 215–232. 10.3109/07388551.2014.923986.
- Bourgaud, F.; Gravot, A.; Milesi, S.; Gontier, E. Production of Plant Secondary Metabolites: A Historical Perspective. Plant Sci. 2001, 161(5), 839–851. 10.1016/S0168-9452(01)00490-3.
- Montero, R.; Pérez‐Bueno, M. L.; Barón, M.; Florez‐Sarasa, I.; Tohge, T.; Fernie, A. R.; Flexas, J.; Bota, J. Alterations in Primary and Secondary Metabolism in Vitis Vinifera ‘Malvasía De Banyalbufar’upon Infection with Grapevine Leafroll‐Associated Virus 3. Physiol. Plant. 2016. 10.1111/ppl.12440.
- Okumoto, S.; Funck, D.; Trovato, M.; Forlani, G. Editorial: Amino Acids of the Glutamate Family: Functions beyond Primary Metabolism. Front. Plant Sci. 2016, 7, 1–3. 10.3389/fpls.2016.00318.
- Kossel, A. Ueber Die Chemische Zusammensetzung Der Zelle. Du Bois-Reymond’s Archiv/Arch Anat. Physiol. Physiol. Abt. 1891, 278,181–186.
- Czapek, F. Biochemie Der Pflanzen. G. Fischer, Germany: 1925; Vol. 2.
- Rasouli, H.; Farzaei, M. H.; Mansouri, K.; Mohammadzadeh, S.; Khodarahmi, R. Plant Cell Cancer: May Natural Phenolic Compounds Prevent Onset and Development of Plant Cell Malignancy? A Literature Review. Molecules. 2016, 21(9), 1104. 10.3390/molecules21091104.
- Villaño, D.; Vilaplana, C.; Medina, S.; Algaba-Chueca, F.; Cejuela-Anta, R.; Martínez-Sanz, J.; Ferreres, F.; Gil-Izquierdo, A. Relationship between the Ingestion of a Polyphenol-Rich Drink, Hepcidin Hormone, and Long-Term Training. Molecules. 2016, 21(10), 1333. 10.3390/molecules21101333.
- Zhou, Z.-Q.; Xiao, J.; Fan, H.-X.; Yu, Y.; He, -R.-R.; Feng, X.-L.; Kurihara, H.; So, K.-F.; Yao, X.-S.; Gao, H. Polyphenols from Wolfberry and Their Bioactivities. Food Chem. 2017, 214, 644–654. 10.1016/j.foodchem.2016.07.105.
- Farzaei, H.; Rahimi, M. R.; Abdollahi, M. The Role of Dietary Polyphenols in the Management of Inflammatory Bowel Disease. Curr. Pharm. Biotechnol. 2015, 16(3), 196–210. PMID: 25601607.
- Khurana, S.; Venkataraman, K.; Hollingsworth, A.; Piche, M.; Tai, T. Polyphenols: Benefits to the Cardiovascular System in Health and in Aging. Nutrients. 2013, 5(10), 3779–3827. 10.3390/nu5103779.
- Pinent, M.; Blay, M.; Serrano, J.; Ardévol, A. Effects of Flavanols on the Enteroendocrine System: Repercussions on Food Intake. Crit. Rev. Food Sci. Nutr. 2015, (just-accepted), 00–00. 10.1080/10408398.2013.871221.
- Visioli, F.; Lastra, C. A. D. L.; Andres-Lacueva, C.; Aviram, M.; Calhau, C.; Cassano, A.; D’Archivio, M.; Faria, A.; Favé, G.; Fogliano, V. Polyphenols and Human Health: A Prospectus. Crit. Rev. Food Sci. Nutr. 2011, 51(6), 524–546. 10.1080/10408391003698677.
- McSweeney, M.; Seetharaman, K. State of Polyphenols in the Drying Process of Fruits and Vegetables. Crit. Rev. Food Sci. Nutr. 2015, 55(5), 660–669.
- Yang, C. S.; Chung, J. Y.; Yang, G.-Y.; Chhabra, S. K.; Lee, M.-J. Tea and Tea Polyphenols in Cancer Prevention. J. Nutr. 2000, 130(2), 472S–478S.
- Bose, M.; Lambert, J. D.; Ju, J.; Reuhl, K. R.; Shapses, S. A.; Yang, C. S. The Major Green Tea Polyphenol,(-)-Epigallocatechin-3-Gallate, Inhibits Obesity, Metabolic Syndrome, and Fatty Liver Disease in High-Fat–Fed Mice. J. Nutr. 2008, 138(9), 1677–1683. PMCID: PMC2586893.
- Lu, C.; Zhu, W.; Shen, C.-L.; Gao, W. Green Tea Polyphenols Reduce Body Weight in Rats by Modulating Obesity-Related Genes. PLoS ONE. 2012, 7(6), e38332.
- Aviram, M.; Dornfeld, L.; Rosenblat, M.; Volkova, N.; Kaplan, M.; Coleman, R.; Hayek, T.; Presser, D.; Fuhrman, B. Pomegranate Juice Consumption Reduces Oxidative Stress, Atherogenic Modifications to LDL, and Platelet Aggregation: Studies in Humans and in Atherosclerotic Apolipoprotein E–Deficient Mice. Am. J. Clin. Nutr. 2000, 71(5), 1062–1076.
- Scalbert, A.; Johnson, I. T.; Saltmarsh, M. Polyphenols: Antioxidants and Beyond. Am. J. Clin. Nutr. 2005, 81(1), 215S–217S.
- Bravo, L. Polyphenols: Chemistry, Dietary Sources, Metabolism, and Nutritional Significance. Nutr. Rev. 1998, 56(11), 317–333. PMID: 9838798.
- Brglez Mojzer, E.; Knez Hrnčič, M.; Škerget, M.; Knez, Ž.; Bren, U. Polyphenols: Extraction Methods, Antioxidative Action, Bioavailability and Anticarcinogenic Effects. Molecules. 2016, 21(7), 901–939. 10.3390/molecules21070901.
- Shao, Y.; Bao, J. Polyphenols in Whole Rice Grain: Genetic Diversity and Health Benefits. Food Chem. 2015, 180, 86–97. 10.1016/j.foodchem.2015.02.027.
- Pereira, D. M.; Valentão, P.; Pereira, J. A.; Andrade, P. B. Phenolics: From Chemistry to Biology. Molecules. 2009, 14(6), 2202–2211.
- Zhang, H.; Tsao, R. Dietary Polyphenols. Oxidative Stress Antioxidant Anti-Inflammatory Effects. Cur. Opinion Food Sci. 2016, 8, 33–42.
- Moga, M.; Dimienescu, O.; Arvatescu, C.; Mironescu, A.; Dracea, L.; Ples, L. The Role of Natural Polyphenols in the Prevention and Treatment of Cervical Cancer—An Overview. Molecules. 2016, 21(8), 1055.
- Miglio, C.; Chiavaro, E.; Visconti, A.; Fogliano, V.; Pellegrini, N. Effects of Different Cooking Methods on Nutritional and Physicochemical Characteristics of Selected Vegetables. J. Agric. Food Chem. 2007, 56(1), 139–147.
- Napolitano, A.; Cascone, A.; Graziani, G.; Ferracane, R.; Scalfi, L.; Di Vaio, C.; Ritieni, A.; Fogliano, V. Influence of Variety and Storage on the Polyphenol Composition of Apple Flesh. J. Agric. Food Chem. 2004, 52(21), 6526–6531.
- Xiao, J. Phytochemicals in Food and Nutrition. Crit. Rev. Food Sci. Nutr. 2016, 56(1), S1–S3.
- Pangeni, R.; Sahni, J. K.; Ali, J.; Sharma, S.; Baboota, S. Resveratrol: Review on Therapeutic Potential and Recent Advances in Drug Delivery. Expert Opin. Drug Deliv. 2014, 11(8), 1285–1298.
- Scopus. Scopus Database, USA (20.10.2016); 2016.
- Rendeiro, C.; Vauzour, D.; Rattray, M.; Waffo-Téguo, P.; Mérillon, J. M.; Butler, L. T.; Williams, C. M.; Spencer, J. P. Dietary Levels of Pure Flavonoids Improve Spatial Memory Performance and Increase Hippocampal Brain-Derived Neurotrophic Factor. PLoS ONE. 2013, 8(5), e63535.
- Nie, N. H.; Bent, D. H.; Hull, C. H. SPSS: Statistical Package for the Social Sciences; McGraw-Hill: New York, 1970.
- Kaufman, L.; Rousseeuw, P. J. Finding Groups in Data: An Introduction to Cluster Analysis. John Wiley & Sons, New Jercy, USA: 2009; Vol. 344.
- Tian, Y.; Liimatainen, J.; Alanne, A.-L.; Lindstedt, A.; Liu, P.; Sinkkonen, J.; Kallio, H.; Yang, B. Phenolic Compounds Extracted by Acidic Aqueous Ethanol from Berries and Leaves of Different Berry Plants. Food Chem. 2017, 220, 266–281. 10.1016/j.foodchem.2016.09.145.
- Botelho, G.; Canas, S.; Lameiras, J. 14 - Development of Phenolic Compounds Encapsulation Techniques as a Major Challenge for Food Industry and for Health and Nutrition Fields A2 - Grumezescu, Alexandru Mihai, in Nutrient Delivery; Academic Press, USA : 2017; 535–586.
- Archivio, D.; Filesi, M. C.; Di Benedetto, R.; Gargiulo, R.; Giovannini, C.; Masella, R. Polyphenols, Dietary Sources and Bioavailability. Annali dell’Istituto Superiore Di Sanità. 2007, 43(4), 348.
- Lattanzio, V.; Lattanzio, V. M.; Cardinali, A. Role of Polyphenols in the Resistance Mechanisms of Plants against Fungal Pathogens and Insects, in Phytochemistry, Kerala, India ; 2006; 23–67.
- Gómez-Caravaca, A.; Verardo, V.; Segura-Carretero, A.; Fernández-Gutiérrez, A.; Caboni, M.; Watson, R. Phenolic Compounds and Saponins in Plants Grown under Different Irrigation Regimes; Academic Press: New York, USA: 2013; 37–48.
- Ajila, C.; Brar, S.; Verma, M.; Tyagi, R.; Godbout, S.; Valero, J. Extraction and Analysis of Polyphenols: Recent Trends. Crit. Rev. Biotechnol. 2011, 31(3), 227–249. 10.3109/07388551.2010.513677.
- Ignat, I.; Volf, I.; Popa, V. I. A Critical Review of Methods for Characterisation of Polyphenolic Compounds in Fruits and Vegetables. Food Chem. 2011, 126(4), 1821–1835. 10.1016/j.foodchem.2010.12.026.
- Schöttner, M.; Ganßer, D.; Spiteller, G. Lignans from the Roots of Urtica Dioica and Their Metabolites Bind to Human Sex Hormone Binding Globulin (SHBG). Planta Med. 1997, 63(06), 529–532.
- Neveu, V.; Perez-Jimenez, J.; Vos, F.; Crespy, V.; Du Chaffaut, L.; Mennen, L.; Knox, C.; Eisner, R.; Cruz, J.; Wishart, D. Phenol-Explorer: An Online Comprehensive Database on Polyphenol Contents in Foods. Database. 2010, 2010, 1–9. 10.1093/database/bap024.
- Bhagwat, S.; Haytowitz, D. B.; Holden, J. M. USDA Database for the Flavonoid Content of Selected Foods, Release 3.1; US Department of Agriculture: Beltsville, MD, USA, 2014.
- Coskun, O.; Kanter, M.; Korkmaz, A.; Oter, S. Quercetin, a Flavonoid Antioxidant, Prevents and Protects Streptozotocin-Induced Oxidative Stress and Β-Cell Damage in Rat Pancreas. Pharm. Res. 2005, 51(2), 117–123.
- Nijveldt, R. J.; Van Nood, E.; Van Hoorn, D. E.; Boelens, P. G.; Van Norren, K.; Van Leeuwen, P. A. Flavonoids: A Review of Probable Mechanisms of Action and Potential Applications. Am. J. Clin. Nutr. 2001, 74(4), 418–425.
- Kohn, M. J. You are What You Eat. Science. 1999, 283(5400), 335–336.
- Herzog, E. D.; Muglia, L. J. You are When You Eat. Nat. Neurosci. 2006, 9(3), 300–302.
- Liang, D.; Silverman, J. “You are What You Eat”: Diet Modifies Cuticular Hydrocarbons and Nestmate Recognition in the Argentine Ant, Linepithema Humile. Naturwissenschaften. 2000, 87(9), 412–416.
- Taylor, L. You are What You Eat. Vet. Nurs. J. 2005, 20(2), 34–34.
- Vita, J. A. Polyphenols and Cardiovascular Disease: Effects on Endothelial and Platelet Function. Am. J. Clin. Nutr. 2005, 81(1), 292S–297S. PMID: 15640493.
- Kanadaswami, C.; Lee, L.-T.; Lee, -P.-P. H.; Hwang, -J.-J.; Ke, F.-C.; Huang, Y.-T.; Lee, M.-T. The Antitumor Activities of Flavonoids. In Vivo. 2005, 19(5), 895–909.
- Chen, A. Y.; Chen, Y. C. A Review of the Dietary Flavonoid, Kaempferol on Human Health and Cancer Chemoprevention. Food Chem. 2013, 138(4), 2099–2107. 10.1016/j.foodchem.2012.11.139.
- Guiné, R.; Ferreira, M.; Correia, P.; Duarte, J. Perception of Health Benefits of Dietary Fibre among the Portuguese Population. Atención Primaria. 2016, 48, 65–65.
- Hollman, P.; De Vries, J.; Van Leeuwen, S. D.; Mengelers, M.; Katan, M. B. Absorption of Dietary Quercetin Glycosides and Quercetin in Healthy Ileostomy Volunteers. Am. J. Clin. Nutr. 1995, 62(6), 1276–1282.
- Hosseinzadeh, H.; Nassiri-Asl, M. Review of the Protective Effects of Rutin on the Metabolic Function as an Important Dietary Flavonoid. J. Endocrinol. Invest. 2014, 37(9), 783–788. Epub. 10.1007/s40618-014-0096-3.
- Little, C.; Combet, E.; McMillan, D.; Horgan, P.; Roxburgh, C. The Role of Dietary Polyphenols in the Moderation of the Inflammatory Response in Early Stage Colorectal Cancer. Crit. Rev. Food Sci. Nutr. 2015, 57(11), 2310–2320. 10.1080/10408398.2014.997866.
- Liu, R. H. Health Benefits of Dietary Flavonoids: Flavonols and Flavones. Fruit Q. 2002, 10, 21–23.
- Calderon-Montano, M.; Burgos-Morón, J. E.; Pérez-Guerrero, C.; López-Lázaro, M. A Review on the Dietary Flavonoid Kaempferol. Mini-Rev. Med. Chem. 2011, 11(4), 298–344.
- Williamson, G.; Day, A.; Plumb, G.; Couteau, D. Human Metabolic Pathways of Dietary Flavonoids and Cinnamates. Biochem. Soc. Trans. 2000, 28(2), 16–22.
- Pandey, K. B.; Rizvi, S. I. Plant Polyphenols as Dietary Antioxidants in Human Health and Disease. Oxid. Med. Cell. Longev. 2009, 2(5), 270–278. 10.4161/oxim.2.5.9498.
- Taylor, P. W.; Hamilton-Miller, J. M.; Stapleton, P. D. Antimicrobial Properties of Green Tea Catechins. Food Sci. Technol. Bull. Functional Foods. 2005, 2, 71–81. PMCID: PMC2763290.
- Diniz, T. C.; Silva, J. C.; Lima-Saraiva, S. R. G. D.; Ribeiro, F. P. R. D. A.; Pacheco, A. G. M.; De Freitas, R. M.; Quintans-Júnior, L. J.; Quintans, J. D. S. S.; Mendes, R. L.; Almeida, J. R. G. D. S. The Role of Flavonoids on Oxidative Stress in Epilepsy. Oxid. Med. Cell. Longev. 2015, 2015.
- Ramalingam, R.; Nath, A. R.; Madhavi, B. B.; Nagulu, M.; Balasubramaniam, A. Free Radical Scavenging and Antiepileptic Activity of Leucas Lanata. J. Pharm. Res. 2013, 6(3), 368–372. 10.1016/j.jopr.2013.03.011.
- Choudhary, N.; Bijjem, K. R. V.; Kalia, A. N. Antiepileptic Potential of Flavonoids Fraction from the Leaves of Anisomeles Malabarica. J. Ethnopharmacol. 2011, 135(2), 238–242. 10.1016/j.jep.2011.02.019.
- Costa Marques, T. H.; Santos De Melo, C. H.; De Carvalho, F.; Rusbene, B.; Costa, L. M.; De Souza, A. A.; David, J. M.; De Lima David, J. P.; De Freitas, R. M. Phytochemical Profile and Qualification of Biological Activity of an Isolated Fraction of Bellis Perennis. Biol. Res. 2013, 46(3), 231–238. 10.4067/S0716-97602013000300002.
- Santangelo, C.; Varì, R.; Scazzocchio, B.; Di Benedetto, R.; Filesi, C.; Masella, R. Polyphenols, Intracellular Signalling and Inflammation. Annali Istituto Superiore Di Sanita. 2007, 43(4), 394–405. PMID: 18209273.
- Luceri, C.; Caderni, G.; Sanna, A.; Dolara, P. Red Wine and Black Tea Polyphenols Modulate the Expression of Cycloxygenase-2, Inducible Nitric Oxide Synthase and Glutathione-Related Enzymes in Azoxymethane-Induced F344 Rat Colon Tumors. J. Nutr. 2002, 132(6), 1376–1379. PMID: 12042461.
- Ferrandiz, M.; Alcaraz, M. Anti-Inflammatory Activity and Inhibition of Arachidonic Acid Metabolism by Flavonoids. Inflamm. Res. 1991, 32(3), 283–288. 10.1007/BF01980887.
- Kim, H.; Mani, I.; Iversen, L.; Ziboh, V. Effects of Naturally-Occurring Flavonoids and Biflavonoids on Epidermal Cyclooxygenase and Lipoxygenase from Guinea-Pigs. Prostaglandins Leukot. Essent. Fatty Acids. 1998, 58(1), 17–24. PMID: 9482162.
- Hou, D.-X.; Luo, D.; Tanigawa, S.; Hashimoto, F.; Uto, T.; Masuzaki, S.; Fujii, M.; Sakata, Y. Prodelphinidin B-4 3′-O-Gallate, a Tea Polyphenol, Is Involved in the Inhibition of COX-2 and iNOS via the Downregulation of Tak1-Nf-Κb Pathway. Biochem. Pharmacol. 2007, 74(5), 742–751. 10.1016/j.bcp.2007.06.006.
- Hou, D.-X.; Masuzaki, S.; Hashimoto, F.; Uto, T.; Tanigawa, S.; Fujii, M.; Sakata, Y. Green Tea Proanthocyanidins Inhibit Cyclooxygenase-2 Expression in LPS-activated Mouse Macrophages: Molecular Mechanisms and Structure–Activity Relationship. Arch. Biochem. Biophys. 2007, 460(1), 67–74. 10.1016/j.abb.2007.01.009.
- Ozcan, T.; Akpinar-Bayizit, A.; Yilmaz-Ersan, L.; Delikanli, B. Phenolics in Human Health. Int. J. Chem. Eng. Appl. 2014, 5(5), 393–396.
- Petti, S.; Scully, C. Polyphenols, Oral Health and Disease: A Review. J. Dent. 2009, 37(6), 413–423.
- Bensalem, J.; Dal-Pan, A.; Gillard, E.; Calon, F.; Pallet, V. Protective Effects of Berry Polyphenols against Age-Related Cognitive Impairment. Nutr. Aging. 2016, 3(2–4), 89–106. 10.3233/NUA-150051.
- Schneider, M.; Norman, R.; Steyn, N.; Bradshaw, D.; Collaboration, S. A. C. R. A. Estimating the Burden of Disease Attributable to Low Fruit and Vegetable Intake in South Africa in 2000. South. Afr. Med. J. 2007, 97(8), 717–723.
- Whitley, A. C.; Sweet, D. H.; Walle, T. Site‐Specific Accumulation of the Cancer Preventive Dietary Polyphenol Ellagic Acid in Epithelial Cells of the Aerodigestive Tract. J. Pharm. Pharmacol. 2006, 58(9), 1201–1209.
- Kumar, S.; Pandey, A. K. Chemistry and Biological Activities of Flavonoids: An Overview. Sci. World J. 2013, 2013, 1–17.
- Gülçin, İ. Antioxidant Properties of Resveratrol: A Structure–Activity Insight. Innovative Food Sci. Emer. Technol. 2010, 11(1), 210–218. 10.1016/j.ifset.2009.07.002.
- Torel, J.; Cillard, J.; Cillard, P. Antioxidant Activity of Flavonoids and Reactivity with Peroxy Radical. Phytochemistry. 1986, 25(2), 383–385. 10.1016/S0031-9422(00)85485-0.
- Cos, P.; Ying, L.; Calomme, M.; Hu, J. P.; Cimanga, K.; Van Poel, B.; Pieters, L.; Vlietinck, A. J.; Berghe, D. V. Structure-Activity Relationship and Classification of Flavonoids as Inhibitors of Xanthine Oxidase and Superoxide Scavengers. J. Nat. Prod. 1998, 61(1), 71–76. 10.1021/np970237h.
- Dehmlow, C.; Erhard, J.; De Groot, H. Inhibition of Kupffer Cell Functions as an Explanation for the Hepatoprotective Properties of Silibinin. Hepatology. 1996, 23(4), 749–754. 10.1053/jhep.1996.v23.pm0008666328.
- Friesenecker, B.; Tsai, A.; Allegra, C.; Intaglietta, M. Oral Administration of Purified Micronized Flavonoid Fraction Suppresses Leukocyte Adhesion in Ischemia-Reperfusion Injury: In Vivo Observations in the Hamster Skin Fold. J. Vasc. Res. 1994, 14(1–2), 50–55.
- Lima, G. P. P.; Vianello, F.; Corrêa, C. R.; Da Silva Campos, R. A.; Borguini, M. G. Polyphenols in Fruits and Vegetables and Its Effect on Human Health. Food Nutr. Sci. 2014, 5(11), 1065–1082. 10.4236/fns.2014.511117.
- Harcourt, B. E.; Sourris, K. C.; Coughlan, M. T.; Walker, K. Z.; Dougherty, S. L.; Andrikopoulos, S.; Morley, A. L.; Thallas-Bonke, V.; Chand, V.; Penfold, S. A. Targeted Reduction of Advanced Glycation Improves Renal Function in Obesity. Kidney Int. 2011, 80(2), 190–198. Epub. 10.1038/ki.2011.57.
- Yamagishi, A.; Kunisawa, T.; Nagashima, M.; Takahata, O.; Iwasaki, H. [Clinical Usefulness of Continuous Cardiac Output Measurement: PulseCO]. Masui. 2009, 58(4), 422–425.
- Rodiño-Janeiro, B. K.; Salgado-Somoza, A.; Teijeira-Fernández, E.; González-Juanatey, J. R.; Álvarez, E.; Eiras, S. Receptor for Advanced Glycation End-Products Expression in Subcutaneous Adipose Tissue Is Related to Coronary Artery Disease. Eur. J. Endocrinol. 2011, 164(4), 529–537.
- Alappat, L.; Awad, A. B. Curcumin and Obesity: Evidence and Mechanisms. Nutr. Rev. 2010, 68(12), 729–738. 10.1111/j.1753-4887.2010.00341.x.
- Shen, C.-L.; Cao, J. J.; Dagda, R. Y.; Tenner, T. E., Jr; Chyu, M.-C.; Yeh, J. K. Supplementation with Green Tea Polyphenols Improves Bone Microstructure and Quality in Aged, Orchidectomized Rats. Calcif. Tissue Int. 2011, 88(6), 455–463.
- Araújo, J. R.; Gonçalves, P.; Martel, F. Chemopreventive Effect of Dietary Polyphenols in Colorectal Cancer Cell Lines. Nutr. Rev. 2011, 31(2), 77–87. 10.1016/j.nutres.2011.01.006.
- Aguirre, L.; Arias, N.; Macarulla, M. T.; Gracia, A.; Portillo, M. P. Beneficial Effects of Quercetin on Obesity and Diabetes. Open Nutraceuticals J. 2011, 4, 189–198.
- Kelly, G. S. Quercetin. Altern. Med. Rev. 2011, 16(2), 172–194.
- Jung, J. Y.; Lim, Y.; Moon, M. S.; Kim, J. Y.; Kwon, O. Onion Peel Extracts Ameliorate Hyperglycemia and Insulin Resistance in High Fat Diet/Streptozotocin-Induced Diabetic Rats. J. Nutr. Metab. 2011, 8(1), 8–18. 10.1186/1743-7075-8-18.
- Rauter, A. P.; Martins, A.; Borges, C.; Mota‐Filipe, H.; Pinto, R.; Sepodes, B.; Justino, J. Antihyperglycaemic and Protective Effects of Flavonoids on Streptozotocin–Induced Diabetic Rats. Phytother. Res. 2010, 24(S2), S133–S138.
- Choi, M.; Jung, U.; Yeo, J.; Kim, M.; Lee, M. Genistein and Daidzein Prevent Diabetes Onset by Elevating Insulin Level and Altering Hepatic Gluconeogenic and Lipogenic Enzyme Activities in Non‐Obese Diabetic (NOD) Mice. Diabetes Metab. Res. Rev. 2008, 24(1), 74–81. 10.1002/dmrr.780.
- González-Castejón, M.; Rodriguez-Casado, A. Dietary Phytochemicals and Their Potential Effects on Obesity: A Review. Pharm. Res. 2011, 64(5), 438–455. 10.1016/j.phrs.2011.07.004.
- Zhou, H.; Beevers, C. S.; Huang, S. The Targets of Curcumin. Curr Drug Targets. 2011, 12(3), 332–347.
- Aggarwal, B. B.; Shishodia, S. Molecular Targets of Dietary Agents for Prevention and Therapy of Cancer. Biochem. Pharmacol. 2006, 71(10), 1397–1421. 10.1016/j.bcp.2006.02.009.
- Boots, A. W.; Haenen, G. R.; Bast, A. Health Effects of Quercetin: From Antioxidant to Nutraceutical. Eur. J. Pharmacol. 2008, 585(2), 325–337. Epub. 10.1016/j.ejphar.2008.03.008.
- Buchweitz, M.; Kroon, P. A.; Rich, G. T.; Wilde, P. J. Quercetin Solubilisation in Bile Salts: A Comparison with Sodium Dodecyl Sulphate. Food Chem. 2016, 211, 356–364. 10.1016/j.foodchem.2016.05.034.
- Amić, A.; Lučić, B.; Stepanić, V.; Marković, Z.; Marković, S.; Dimitrić Marković, J. M.; Amić, D. Free Radical Scavenging Potency of Quercetin Catecholic Colonic Metabolites: Thermodynamics of 2h+/2e− Processes. Food Chem. 2017, 218, 144–151. 10.1016/j.foodchem.2016.09.018.
- Popova, A. V.; Hincha, D. K. Effects of Flavonol Glycosides on Liposome Stability during Freezing and Drying. Biochim. Biophys. Acta. 2016, 1858(12), 3050–3060.
- Harris, S.; Brunton, N.; Tiwari, U.; Cummins, E. Human Exposure Modelling of Quercetin in Onions (Allium Cepa L.) following Thermal Processing. Food Chem. 2015, 187, 135–139. 10.1016/j.foodchem.2015.04.035.
- Lee, J.; Mitchell, A. E. Quercetin and Isorhamnetin Glycosides in Onion (Allium Cepa L.): Varietal Comparison, Physical Distribution, Coproduct Evaluation, and Long-Term Storage Stability. J. Agric. Food Chem. 2011, 59(3), 857–863. 10.1021/jf1033587.
- Murakami, A.; Ashida, H.; Terao, J. Multitargeted Cancer Prevention by Quercetin. Cancer Lett. 2008, 269(2), 315–325.
- Shinoki, A.; Lang, W.; Thawornkuno, C.; Kang, H.-K.; Kumagai, Y.; Okuyama, M.; Mori, H.; Kimura, A.; Ishizuka, S.; Hara, H. A Novel Mechanism for the Promotion of Quercetin Glycoside Absorption by Megalo Α-1,6-Glucosaccharide in the Rat Small Intestine. Food Chem. 2013, 136(2), 293–296. 10.1016/j.foodchem.2012.08.028.
- Chen, X.; Zou, L.; Liu, W.; McClements, D. J. Potential of Excipient Emulsions for Improving Quercetin Bioaccessibility and Antioxidant Activity: An in Vitro Study. J. Agric. Food Chem. 2016, 64(18), 3653–3660. Epub. 10.1021/acs.jafc.6b01056.
- Hisanaga, A.; Mukai, R.; Sakao, K.; Terao, J.; Hou, D. X. Anti‐Inflammatory Effects and Molecular Mechanisms of 8‐Prenyl Quercetin. Mol. Nutr. Food Res. 2016, 60(5), 1020–1032. Epub. 10.1002/mnfr.201500871.
- Guillermo Gormaz, J.; Quintremil, S.; Rodrigo, R. Cardiovascular Disease: A Target for the Pharmacological Effects of Quercetin. Curr. Top. Med. Chem. 2015, 15(17), 1735–1742. PMID: 25915608.
- Vaidya, B.; Cho, S.-Y.; Oh, K.-S.; Kim, S. H.; Kim, Y. O.; Jeong, E.-H.; Nguyen, T. T.; Kim, S. H.; Kim, I. S.; Kwon, J. Effectiveness of Periodic Treatment of Quercetin against Influenza A Virus H1N1 through Modulation of Protein Expression. J. Agric. Food Chem. 2016. 10.1021/acs.jafc.6b00148.
- Al-Jabban, S.; Zhang, X.; Chen, G.; Mekuria, E. A.; Rakotondraibe, L. H.; Chen, Q.-H. Synthesis and Anti-Proliferative Effects of Quercetin Derivatives. Nat. Prod. Commun. 2015, 10(12), 2113–2118. PMID: 26882678.
- Snyder, S. M.; Zhao, B.; Luo, T.; Kaiser, C.; Cavender, G.; Hamilton-Reeves, J.; Sullivan, D. K.; Shay, N. F. Consumption of Quercetin and Quercetin-Containing Apple and Cherry Extracts Affects Blood Glucose Concentration, Hepatic Metabolism, and Gene Expression Patterns in Obese C57BL/6J High Fat–Fed Mice. J. Nutr. 2016, 146(5), 1001–1007.
- Murota, K.; Terao, J. Antioxidative Flavonoid Quercetin: Implication of Its Intestinal Absorption and Metabolism. Arch. Biochem. Biophys. 2003, 417(1), 12–17.
- Andarwulan, N.; Batari, R.; Sandrasari, D. A.; Bolling, B.; Wijaya, H. Flavonoid Content and Antioxidant Activity of Vegetables from Indonesia. Food Chem. 2010, 121(4), 1231–1235. 10.1016/j.foodchem.2010.01.033.
- Iacopini, P.; Baldi, M.; Storchi, P.; Sebastiani, L. Catechin, Epicatechin, Quercetin, Rutin and Resveratrol in Red Grape: Content, in Vitro Antioxidant Activity and Interactions. J. Food Composition Anal. 2008, 21(8), 589–598. 10.1016/j.jfca.2008.03.011.
- Xiao, J.; Kai, G. A Review of Dietary Polyphenol-Plasma Protein Interactions: Characterization, Influence on the Bioactivity, and Structure-Affinity Relationship. Crit. Rev. Food Sci. Nutr. 2012, 52(1), 85–101.
- Petersen, B.; Egert, S.; Bosy-Westphal, A.; Müller, M. J.; Wolffram, S.; Hubbermann, E. M.; Rimbach, G.; Schwarz, K. Bioavailability of Quercetin in Humans and the Influence of Food Matrix Comparing Quercetin Capsules and Different Apple Sources. Food Res. Int. 2016, 88(Part A), 159–165.
- Russo, M.; Spagnuolo, C.; Tedesco, I.; Bilotto, S.; Russo, G. L. The Flavonoid Quercetin in Disease Prevention and Therapy: Facts and Fancies. Biochem. Pharmacol. 2012, 83(1), 6–15.
- Wang, L.; Morris, M. E. Liquid Chromatography–Tandem Mass Spectroscopy Assay for Quercetin and Conjugated Quercetin Metabolites in Human Plasma and Urine. J. Chromatogr. B. 2005, 821(2), 194–201.
- Day, A. J.; Gee, J. M.; DuPont, M. S.; Johnson, I. T.; Williamson, G. Absorption of Quercetin-3-Glucoside and Quercetin-4′-Glucoside in the Rat Small Intestine: The Role of Lactase Phlorizin Hydrolase and the Sodium-Dependent Glucose Transporter. Biochem. Pharmacol. 2003, 65(7), 1199–1206.
- Piskula, M. K. Factors Affecting Flavonoids Absorption. Biofactors. 2000, 12(1–4), 175–180.
- Arts, I. C.; Sesink, A. L.; Faassen-Peters, M.; Hollman, P. C. The Type of Sugar Moiety Is a Major Determinant of the Small Intestinal Uptake and Subsequent Biliary Excretion of Dietary Quercetin Glycosides. Br. J. Nutr. 2004, 91(06), 841–847. 10.1079/BJN20041123.
- Crespy, V.; Morand, C.; Manach, C.; Besson, C.; Demigne, C.; Remesy, C. Part of Quercetin Absorbed in the Small Intestine Is Conjugated and Further Secreted in the Intestinal Lumen. Am. J. Physiol. Gastrointest. Liver Physiol. 1999, 277(1), G120–G126.
- Meng, X.; Maliakal, P.; Lu, H.; Lee, M.-J.; Yang, C. S. Urinary and Plasma Levels of Resveratrol and Quercetin in Humans, Mice, and Rats after Ingestion of Pure Compounds and Grape Juice. J. Agric. Food Chem. 2004, 52(4), 935–942.
- Bischoff, S. C. Quercetin: Potentials in the Prevention and Therapy of Disease. Curr. Opin. Clin. Nutr. Metab. Care. 2008, 11(6), 733–740. 10.1097/MCO.0b013e32831394b8.
- Perez-Vizcaino, F.; Duarte, J.; Andriantsitohaina, R. Endothelial Function and Cardiovascular Disease: Effects of Quercetin and Wine Polyphenols. Free Radic. Res. 2006, 40(10), 1054–1065.
- Laslett, L. J.; Alagona, P.; Clark, B. A.; Drozda, J. P.; Saldivar, F.; Wilson, S. R.; Poe, C.; Hart, M. The Worldwide Environment of Cardiovascular Disease: Prevalence, Diagnosis, Therapy, and Policy Issues: A Report from the American College of Cardiology. J. Am. Coll. Cardiol. 2012, 60(25_S), S1–S49. 10.1016/j.jacc.2012.11.002.
- Xu, S.-W.; Law, B. Y. K.; Mok, S. W. F.; Leung, E. L. H.; Fan, X. X.; Coghi, P. S.; Zeng, W.; Leung, C.-H.; Ma, D.-L.; Liu, L. Autophagic Degradation of Epidermal Growth Factor Receptor in Gefitinib-Resistant Lung Cancer by Celastrol. Int. J. Oncol. 2016, 49(4), 1576–1588.
- Chan, C.-Y.; Lien, C.-H.; Lee, M.-F.; Huang, C.-Y. Quercetin Suppresses Cellular Migration and Invasion in Human Head and Neck Squamous Cell Carcinoma (HNSCC). Biomedicine. 2016, 6(3), 10–15. 10.7603/s40681-016-0015-3.
- Pan, H.-C.; Jiang, Q.; Yu, Y.; Mei, J.-P.; Cui, Y.-K.; Zhao, W.-J. Quercetin Promotes Cell Apoptosis and Inhibits the Expression of MMP-9 and Fibronectin via the AKT and ERK Signalling Pathways in Human Glioma Cells. Neurochem. Int. 2015, 80, 60–71. 10.1016/j.neuint.2014.12.001.
- Zhang, S.; Qin, C.; Safe, S. H. Flavonoids as Aryl Hydrocarbon Receptor Agonists/Antagonists: Effects of Structure and Cell Context. Environ. Health Perspect. 2003, 111(16), 1877.
- Chen, W.; Wang, X.; Zhuang, J.; Zhang, L.; Lin, Y. Induction of Death Receptor 5 and Suppression of Survivin Contribute to Sensitization of TRAIL-induced Cytotoxicity by Quercetin in Non-Small Cell Lung Cancer Cells. Carcinogenesis. 2007, 28(10), 2114–2121. 10.1093/carcin/bgm133.
- Xu, L.; Qu, X.; Zhang, Y.; Hu, X.; Yang, X.; Hou, K.; Teng, Y.; Zhang, J.; Sada, K.; Liu, Y. Oxaliplatin Enhances TRAIL‐induced Apoptosis in Gastric Cancer Cells by CBL‐regulated Death Receptor Redistribution in Lipid Rafts. FEBS Lett. 2009, 583(5), 943–948.
- Tarahovsky, Y. S.; Kim, Y. A.; Yagolnik, E. A.; Muzafarov, E. N. Flavonoid–Membrane Interactions: Involvement of Flavonoid–Metal Complexes in Raft Signaling. Biochim. Biophys. Acta. 2014, 1838(5), 1235–1246. 10.1016/j.bbamem.2014.01.021.
- Simons, K.; Toomre, D. Lipid Rafts and Signal Transduction. Nat. Rev. Mol. . Biol. 2000, 1(1), 31–39.
- Lamson, D. W.; Brignall, M. S. Antioxidants and Cancer, Part 3: Quercetin. Altern. Med. Rev. 2000, 5(3), 196–208. PMID: 10869101.
- Lee, K. W.; Bode, A. M.; Dong, Z. Molecular Targets of Phytochemicals for Cancer Prevention. Nat. Rev. Cancer. 2011, 11(3), 211–218. 10.1038/nrc3017.
- Annapurna, A.; Reddy, C. S.; Akondi, R. B.; Rao, S. R. Cardioprotective Actions of Two Bioflavonoids, Quercetin and Rutin, in Experimental Myocardial Infarction in Both Normal and Streptozotocin‐Induced Type I Diabetic Rats. J. Pharm. Pharmacol. 2009, 61(10), 1365–1374. 10.1211/jpp/61.10.0014.
- Sugamura, K.; Keaney, J. F. Reactive Oxygen Species in Cardiovascular Disease. Free Radic. Biol. Med. 2011, 51(5), 978–992.
- Liu, H.; Zhang, L.; Lu, S. Evaluation of Antioxidant and Immunity Activities of Quercetin in Isoproterenol-Treated Rats. Molecules. 2012, 17(4), 4281–4291. 10.3390/molecules17044281.
- Glässer, G.; Graefe, E.; Struck, F.; Veit, M.; Gebhardt, R. Comparison of Antioxidative Capacities and Inhibitory Effects on Cholesterol Biosynthesis of Quercetin and Potential Metabolites. Phytomedicine. 2002, 9(1), 33–40. 10.1078/0944-7113-00080.
- Chuang, -C.-C.; Martinez, K.; Xie, G.; Kennedy, A.; Bumrungpert, A.; Overman, A.; Jia, W.; McIntosh, M. K. Quercetin Is Equally or More Effective than Resveratrol in Attenuating Tumor Necrosis Factor-Α–Mediated Inflammation and Insulin Resistance in Primary Human Adipocytes. Am. J. Clin. Nutr. 2010, 92(6), 1511–1521. Epub. 10.3945/ajcn.2010.29807.
- Setorki, M.; Asgary, S.; Eidi, A.; Esmaeil, N. Effects of Apple Juice on Risk Factors of Lipid Profile, Inflammation and Coagulation, Endothelial Markers and Atherosclerotic Lesions in High Cholesterolemic Rabbits. Lipids Health Dis. 2009, 8(1), 1–9.
- Lee, K.-H.; Park, E.; Lee, H.-J.; Kim, M.-O.; Cha, Y.-J.; Kim, J.-M.; Lee, H.; Shin, M.-J. Effects of Daily Quercetin-Rich Supplementation on Cardiometabolic Risks in Male Smokers. Nutr. Res. Pract. 2011, 5(1), 28–33. 10.4162/nrp.2011.5.1.28.
- Oberley, L. W. Free Radicals and Diabetes. Free Radic. Biol. Med. 1988, 5(2), 113–124.
- Delcourt, C.; Cristol, J.-P.; Tessier, F.; Léger, C. L.; Michel, F.; Papoz, L.; Group, P. S. Risk Factors for Cortical, Nuclear, and Posterior Subcapsular Cataracts: The POLA Study. Am. J. Epidemiol. 2000, 151(5), 497–504.
- Ottonello, S.; Foroni, C.; Carta, A.; Petrucco, S.; Maraini, G. Oxidative Stress and Age-Related Cataract. Ophthalmologica. 2000, 214(1), 78–85.
- Jung, H. A.; Yoon, N. Y.; Kang, S. S.; Kim, Y. S.; Choi, J. S. Inhibitory Activities of Prenylated Flavonoids from Sophora Flavescens against Aldose Reductase and Generation of Advanced Glycation Endproducts. J. Pharm. Pharmacol. 2008, 60(9), 1227–1236. 10.1211/jpp.60.9.0016.
- Vrensen, G. F. Early Cortical Lens Opacities: A Short Overview. Acta Ophthalmol. 2009, 87(6), 602–610. 10.1111/j.1755-3768.2009.01674.x.
- Stefek, M.; Karasu, C. Eye Lens in Aging and Diabetes: Effect of Quercetin. Rejuvenation Res. 2011, 14(5), 525–534.
- Dayan, N.; Kromidas, L. Formulating, Packaging, and Marketing of Natural Cosmetic Products; John Wiley & Sons, USA: 2011.
- Mulholland, P.; Ferry, D.; Anderson, D.; Hussain, S.; Young, A.; Cook, J.; Hodgkin, E.; Seymour, L.; Kerr, D. Pre-Clinical and Clinical Study of QC12, a Water-Soluble, Pro-Drug of Quercetin. Ann. Oncol. 2001, 12(2), 245–248.
- Chen, C.; Zhou, J.; Ji, C. Quercetin: A Potential Drug to Reverse Multidrug Resistance. Life Sci. 2010, 87(11), 333–338. Epub. 10.1016/j.lfs.2010.07.004.
- Sharma, S.; Ali, A.; Ali, J.; Sahni, J. K.; Baboota, S. Rutin: Therapeutic Potential and Recent Advances in Drug Delivery. Expert Opin. Investig. Drugs. 2013, 22(8), 1063–1079.
- Erlund, I.; Kosonen, T.; Alfthan, G.; Mäenpää, J.; Perttunen, K.; Kenraali, J.; Parantainen, J.; Aro, A. Pharmacokinetics of Quercetin from Quercetin Aglycone and Rutin in Healthy Volunteers. Eur. J. Clin. Pharmacol. 2000, 56(8), 545–553.
- Sattanathan, K.; Dhanapal, C.; Manavalan, R. LDL Lowering Properties of Rutin in Diabetic Patients. Int. J. Pharma Bio Sci. 2010, 1(4), P–473.
- Kamalakkannan, N.; Prince, P. S. M. Antihyperglycaemic and Antioxidant Effect of Rutin, a Polyphenolic Flavonoid, in Streptozotocin‐Induced Diabetic Wistar Rats. Basic Clin. Pharmacol. Toxicol. 2006, 98(1), 97–103.
- Fernandes, A. A. H.; Novelli, E. L. B.; Okoshi, K.; Okoshi, M. P.; Di Muzio, B. P.; Guimarães, J. F. C.; Junior, A. F. Influence of Rutin Treatment on Biochemical Alterations in Experimental Diabetes. Biomed. Pharmacol. 2010, 64(3), 214–219. Epub. 10.1016/j.biopha.2009.08.007.
- Boyle, S.; Dobson, V.; Duthie, S.; Hinselwood, D.; Kyle, J.; Collins, A. Bioavailability and Efficiency of Rutin as an Antioxidant: A Human Supplementation Study. Eur. J. Clin. Nutr. 2000, 54(10), 774–782. PMID: 11083486.
- La Casa, C.; Villegas, I.; De La Lastra, C. A.; Motilva, V.; Calero, M. M. Evidence for Protective and Antioxidant Properties of Rutin, a Natural Flavone, against Ethanol Induced Gastric Lesions. J. Ethnopharmacol. 2000, 71(1), 45–53. PMID: 10904145.
- Manach, C.; Williamson, G.; Morand, C.; Scalbert, A.; Rémésy, C. Bioavailability and Bioefficacy of Polyphenols in Humans. I. Review of 97 Bioavailability Studies. Am. J. Clin. Nutr. 2005, 81(1), 230S–242S.
- Yu, C.-P.; Wu, -P.-P.; Hou, Y.-C.; Lin, S.-P.; Tsai, S.-Y.; Chen, C.-T.; Chao, P.-D. L. Quercetin and Rutin Reduced the Bioavailability of Cyclosporine from Neoral, an Immunosuppressant, through Activating P-Glycoprotein and CYP 3A4. J. Agric. Food Chem. 2011, 59(9), 4644–4648.
- Cai, X.; Fang, Z.; Dou, J.; Yu, A.; Zhai, G. Bioavailability of Quercetin: Problems and Promises. Curr. Med. Chem. 2013, 20(20), 2572–2582. PMID: 23514412.
- Dian, L.; Yu, E.; Chen, X.; Wen, X.; Zhang, Z.; Qin, L.; Wang, Q.; Li, G.; Wu, C. Enhancing Oral Bioavailability of Quercetin Using Novel Soluplus Polymeric Micelles. Nanoscale Res. Lett. 2014, 9(1), 684. 10.1186/1556-276x-9-684.
- Kessler, M.; Ubeaud, G.; Jung, L. Anti‐And Pro‐Oxidant Activity of Rutin and Quercetin Derivatives. J. Pharm. Pharmacol. 2003, 55(1), 131–142. 10.1211/002235702559.
- Kappel, V. D.; Zanatta, L.; Postal, B. G.; Silva, F. R. M. B. Rutin Potentiates Calcium Uptake via Voltage-Dependent Calcium Channel Associated with Stimulation of Glucose Uptake in Skeletal Muscle. Arch. Biochem. Biophys. 2013, 532(2), 55–60.
- Krishna, K. M.; Annapurna, A.; Gopal, G. S.; Chalam, C. R.; Madan, K.; Kumar, V. K.; Prakash, G. J. Partial Reversal by Rutin and Quercetin of Impaired Cardiac Function in Streptozotocin-Induced Diabetic Rats. Can. J. Physiol. Pharmacol. 2005, 83(4), 343–355. 10.1139/y05-009.
- Je, H. D.; Shin, C. Y.; Park, S. Y.; Yim, S. H.; Kum, C.; Huh, I. H.; Kim, J. H.; Sohn, U. D. Combination of Vitamin C and Rutin on Neuropathy and Lung Damage of Diabetes Mellitus Rats. Arch. Pharm. Res. 2002, 25(2), 184–190.
- Cai, E. P.; Lin, J.-K. Epigallocatechin Gallate (EGCG) and Rutin Suppress the Glucotoxicity through Activating IRS2 and AMPK Signaling in Rat Pancreatic Β Cells. J. Agric. Food Chem. 2009, 57(20), 9817–9827. 10.1021/jf902618v.
- Korkmaz, A.; Kolankaya, D. Protective Effect of Rutin on the Ischemia/Reperfusion Induced Damage in Rat Kidney. J. Surg. Res. 2010, 164(2), 309–315. 10.1016/j.jss.2009.03.022.
- Kappel, V. D.; Cazarolli, L. H.; Pereira, D. F.; Postal, B. G.; Zamoner, A.; Reginatto, F. H.; Silva, F. R. M. B. Involvement of GLUT‐4 in the Stimulatory Effect of Rutin on Glucose Uptake in Rat Soleus Muscle. J. Pharm. Pharmacol. 2013, 65(8), 1179–1186. 10.1111/jphp.12066.
- Clouth, A.; Schöfer, H. Treatment of Recalcitrant Facial Verrucae Vulgares with Sinecatechins (Greentea Catechins) Ointment. J. Eur. Acad. Dermatol. Venereol. 2015, 29(1), 178–179. Epub. 10.1111/jdv.12341.
- Higdon, J. V.; Frei, B. Tea Catechins and Polyphenols: Health Effects, Metabolism, and Antioxidant Functions. Crit. Rev. Food Sci. Nutr. 2003, 89–143. 10.1080/10408690390826464.
- Türközü, D.; Acar Tek, N. A Minireview of Effects of Green Tea on Energy Expenditure. Crit. Rev. Food Sci. Nutr. 2015, (just-accepted), 00–00. 10.1080/10408398.2014.986672.
- Kondo, S.; Tsuda, K.; Muto, N.; Ueda, J.-E. Antioxidative Activity of Apple Skin or Flesh Extracts Associated with Fruit Development on Selected Apple Cultivars. Sci. Hortic. 2002, 96(1–4), 177–185. 10.1016/S0304-4238(02)00127-9.
- Gadkari, P. V.; Balaraman, M. Catechins: Sources, Extraction and Encapsulation: A Review. Food Bioprod. Proc. 2015, 93, 122–138. 10.1016/j.fbp.2013.12.004.
- Arts, I. C.; Hollman, P. C.; Feskens, E. J.; De Mesquita, H. B. B.; Kromhout, D. Catechin Intake Might Explain the Inverse Relation between Tea Consumption and Ischemic Heart Disease: The Zutphen Elderly Study. Am. J. Clin. Nutr. 2001, 74(2), 227–232. PMID: 11470725.
- Friedman, M. Overview of Antibacterial, Antitoxin, Antiviral, and Antifungal Activities of Tea Flavonoids and Teas. Mol. Nutr. Food Res. 2007, 51(1), 116–134. 10.1002/mnfr.200600173.
- Park, K. D.; Park, Y. S.; Cho, S. J.; Sun, W. S.; Kim, S. H.; Jung, D. H.; Kim, J. H. Antimicrobial Activity of 3-O-Acyl-(-)-Epicatechin and 3-O-Acyl-(+)-Catechin Derivatives. Planta Med. 2004, 70(03), 272–276.
- Zhao, L.; La, V. D.; Grenier, D. Antibacterial, Antiadherence, Antiprotease, and Anti-Inflammatory Activities of Various Tea Extracts: Potential Benefits for Periodontal Diseases. J. Med. Food. 2013, 16(5), 428–436. 10.1089/jmf.2012.0207.
- Kivits, G.; Weststrate, J.; Tijburg, L. Bioavailability of Catechins from Tea: The Effect of Milk. Eur. J. Clin. Nutr. 1998, 52(5), 356–359.
- Zhu, M.; Chen, Y.; Li, R. C. Oral Absorption and Bioavailability of Tea Catechins. Planta Med. 2000, 66(05), 444–447.
- Chung, J. Y.; Huang, C.; Meng, X.; Dong, Z.; Yang, C. S. Inhibition of Activator Protein 1 Activity and Cell Growth by Purified Green Tea and Black Tea Polyphenols in H-Ras-Transformed Cells: Structure- Activity Relationship and Mechanisms Involved. Cancer Res. 1999, 59(18), 4610–4617.
- Yang, C. S.; Wang, H.; Chen, J. X.; Zhang, J. Effects of Tea Catechins on Cancer Signaling Pathways. Enzymes. 2014, 36, 195–221.
- Navarro-Perán, E.; Cabezas-Herrera, J.; García-Cánovas, F.; Durrant, M. C.; Thorneley, R. N.; Rodríguez-López, J. N. The Antifolate Activity of Tea Catechins. Cancer Res. 2005, 65(6), 2059–2064.
- Shin, E. S.; Park, J.; Shin, J.-M.; Cho, D.; Cho, S. Y.; Shin, D. W.; Ham, M.; Kim, J. B.; Lee, T. R. Catechin Gallates are NADP+-competitive Inhibitors of Glucose-6-Phosphate Dehydrogenase and Other Enzymes that Employ NADP+ as a Coenzyme. Bioorg. Med. Chem. 2008, 16(7), 3580–3586. 10.1016/j.bmc.2008.02.030.
- Ishii, T.; Mori, T.; Tanaka, T.; Mizuno, D.; Yamaji, R.; Kumazawa, S.; Nakayama, T.; Akagawa, M. Covalent Modification of Proteins by Green Tea Polyphenol (–)-Epigallocatechin-3-Gallate through Autoxidation. Free Radic. Biol. Med. 2008, 45(10), 1384–1394. 10.1016/j.freeradbiomed.2008.07.023.
- Huang, W.; Ding, L.; Huang, Q.; Hu, H.; Liu, S.; Yang, X.; Hu, X.; Dang, Y.; Shen, S.; Li, J. Carbonyl Reductase 1 as a Novel Target of (−)‐Epigallocatechin Gallate against Hepatocellular Carcinoma. Hepatology. 2010, 52(2), 703–714. 10.1002/hep.23723.
- Adachi, S.; Nagao, T.; Ingolfsson, H. I.; Maxfield, F. R.; Andersen, O. S.; Kopelovich, L.; Weinstein, I. B. The Inhibitory Effect of (−)-Epigallocatechin Gallate on Activation of the Epidermal Growth Factor Receptor Is Associated with Altered Lipid Order in HT29 Colon Cancer Cells. Cancer Res. 2007, 67(13), 6493–6501. 10.1158/0008-5472.CAN-07-0411.
- Duhon, D.; Bigelow, R. L. H.; Coleman, D. T.; Steffan, J. J.; Yu, C.; Langston, W.; Kevil, C. G.; Cardelli, J. A. The Polyphenol Epigallocatechin-3-Gallate Affects Lipid Rafts to Block Activation of the c-Met Receptor in Prostate Cancer Cells. Mol. Carcinog. 2010, 49(8), 739–749. 10.1002/mc.20649.
- Fujimura, Y.; Yamada, K.; Tachibana, H. A Lipid Raft-Associated 67 kDa Laminin Receptor Mediates Suppressive Effect of epigallocatechin-3-O-gallate on FcεRI Expression. Biochem. Biophys. Res. Commun. 2005, 336(2), 674–681. 10.1016/j.bbrc.2005.08.146.
- Chen, Y.-K.; Cheung, C.; Reuhl, K. R.; Liu, A. B.; Lee, M.-J.; Lu, Y.-P.; Yang, C. S. Effects of Green Tea Polyphenol (−)-Epigallocatechin-3-Gallate on Newly Developed high-fat/Western-style Diet-Induced Obesity and Metabolic Syndrome in Mice. J. Agric. Food Chem. 2011, 59(21), 11862–11871. 10.1021/jf2029016.
- Korkmaz, A.; Reiter, R. J.; Topal, T.; Manchester, L. C.; Oter, S.; Tan, D.-X. Melatonin: An Established Antioxidant Worthy of Use in Clinical Trials. Mol. Med. 2009, 15(1–2), 43–50. 10.2119/molmed.2008.00117.
- Tsatsanis, C.; Androulidaki, A.; Venihaki, M.; Margioris, A. N. Signalling Networks Regulating Cyclooxygenase-2. Int. J. Biochem. Cell Biol. 2006, 38(10), 1654–1661. 10.1016/j.biocel.2006.03.021.
- Hu, K.-Q. Cyclooxygenase 2 (Cox2)-Prostanoid Pathway and Liver Diseases. Prostaglandins Leukot. Essent. Fatty Acids. 2003, 69(5), 329–337.
- Leung, T.-M.; Fung, M.-L.; Liong, E. C.; Lau, T. Y.; Nanji, A. A.; Tipoe, G. L. Role of Nitric Oxide in the Regulation of Fibrogenic Factors in Experimental Liver Fibrosis in Mice. Histol. Histopathol. 2011, 26(2), 201–211. 10.14670/HH-26.201.
- Tipoe, G. L.; Leung, T. M.; Liong, E. C.; Lau, T. Y. H.; Fung, M. L.; Nanji, A. A. Epigallocatechin-3-Gallate (EGCG) Reduces Liver Inflammation, Oxidative Stress and Fibrosis in Carbon Tetrachloride (Ccl 4)-Induced Liver Injury in Mice. Toxicology. 2010, 273(1), 45–52. 10.1016/j.tox.2010.04.014.
- Shankar, E.; Kanwal, R.; Candamo, M.; Gupta, S. Dietary Phytochemicals as Epigenetic Modifiers in Cancer: Promise and Challenges. Semin. Cancer Biol. 2016, 40–41, 82–99. 10.1016/j.semcancer.2016.04.002.
- Russo, G. L.; Vastolo, V.; Ciccarelli, M.; Albano, L.; Macchia, P. E.; Ungaro, P. Dietary Polyphenols and Chromatin Remodelling. Crit. Rev. Food Sci. Nutr. 2017, 57(12), 2589–2599.
- Fang, M. Z.; Wang, Y.; Ai, N.; Hou, Z.; Sun, Y.; Lu, H.; Welsh, W.; Yang, C. S. Tea Polyphenol (−)-Epigallocatechin-3-Gallate Inhibits DNA Methyltransferase and Reactivates Methylation-Silenced Genes in Cancer Cell Lines. Cancer Res. 2003, 63(22), 7563–7570.
- Lee, W. J.; Shim, J.-Y.; Zhu, B. T. Mechanisms for the Inhibition of DNA Methyltransferases by Tea Catechins and Bioflavonoids. Mol. Pharmacol. 2005, 68(4), 1018–1030. 10.1124/mol.104.008367.
- Xu, Y.; Ho, C.-T.; Amin, S. G.; Han, C.; Chung, F.-L. Inhibition of Tobacco-Specific Nitrosamine-Induced Lung Tumorigenesis in A/J Mice by Green Tea and Its Major Polyphenol as Antioxidants. Cancer Res. 1992, 52(14), 3875–3879. PMID: 1617663.
- Srividhya, R.; Jyothilakshmi, V.; Arulmathi, K.; Senthilkumaran, V.; Kalaiselvi, P. Attenuation of Senescence-Induced Oxidative Exacerbations in Aged Rat Brain by (−)-Epigallocatechin-3-Gallate. Int. J. Dev. Neurosci. 2008, 26(2), 217–223.
- Almajano, M. P.; Delgado, M. E.; Gordon, M. H. Albumin Causes a Synergistic Increase in the Antioxidant Activity of Green Tea Catechins in Oil-In-Water Emulsions. Food Chem. 2007, 102(4), 1375–1382. 10.1016/j.foodchem.2006.06.067.
- Trnková, L.; Boušová, I.; Staňková, V.; Dršata, J. Study on the Interaction of Catechins with Human Serum Albumin Using Spectroscopic and Electrophoretic Techniques. J. Mol. Struct. 2011, 985(2), 243–250. 10.1016/j.molstruc.2010.11.001.
- Ishii, T.; Ichikawa, T.; Minoda, K.; Kusaka, K.; Ito, S.; Suzuki, Y.; Akagawa, M.; Mochizuki, K.; Goda, T.; Nakayama, T. Human Serum Albumin as an Antioxidant in the Oxidation of (−)-Epigallocatechin Gallate: Participation of Reversible Covalent Binding for Interaction and Stabilization. Biosci. Biotechnol. Biochem. 2011, 75(1), 100–106. 10.1271/bbb.100600.
- Watanabe, J.; Kawabata, J.; Niki, R. Isolation and Identification of acetyl-CoA Carboxylase Inhibitors from Green Tea (Camellia Sinensis). Biosci. Biotechnol. Biochem. 1998, 62(3), 532–534.
- Lin, J. K.; Lin‐Shiau, S. Y. Mechanisms of Hypolipidemic and Anti‐Obesity Effects of Tea and Tea Polyphenols. Mol. Nutr. Food Res. 2006, 50(2), 211–217. 10.1002/mnfr.200500138.
- Wolfram, S.; Wang, Y.; Thielecke, F. Anti‐Obesity Effects of Green Tea: From Bedside to Bench. Mol. Nutr. Food Res. 2006, 50(2), 176–187.
- Xu, Y.; Zhang, M.; Wu, T.; Dai, S.; Xu, J.; Zhou, Z. The Anti-Obesity Effect of Green Tea Polysaccharides, Polyphenols and Caffeine in Rats Fed with a High-Fat Diet. Food Funct. 2015, 6(1), 296–303. 10.1039/c4fo00970c.
- Fujiki, H.; Sueoka, E.; Watanabe, T.; Suganuma, M. Synergistic Enhancement of Anticancer Effects on Numerous Human Cancer Cell Lines Treated with the Combination of EGCG, Other Green Tea Catechins, and Anticancer Compounds. J. Cancer Res. Clin. Oncol. 2015, 141(9), 1511–1522. Epub. 10.1007/s00432-014-1899-5.
- Downey, M. O.; Dokoozlian, N. K.; Krstic, M. P. Cultural Practice and Environmental Impacts on the Flavonoid Composition of Grapes and Wine: A Review of Recent Research. Am. J. Enol. Vitic. 2006, 57(3), 257–268.
- Huang, W. W.; Chiu, Y. J.; Fan, M. J.; Lu, H. F.; Yeh, H. F.; Li, K. H.; Chen, P. Y.; Chung, J. G.; Yang, J. S. Kaempferol Induced Apoptosis via Endoplasmic Reticulum Stress and Mitochondria‐Dependent Pathway in Human Osteosarcoma U‐2 OS Cells. Mol. Nutr. Food Res. 2010, 54(11), 1585–1595. 10.1002/mnfr.201000005.
- Sharma, V.; Joseph, C.; Ghosh, S.; Agarwal, A.; Mishra, M. K.; Sen, E. Kaempferol Induces Apoptosis in Glioblastoma Cells through Oxidative Stress. Mol. Cancer Ther. 2007, 6(9), 2544–2553.
- Barve, A.; Chen, C.; Hebbar, V.; Desiderio, J.; Saw, C. L. L.; Kong, A. N. Metabolism, Oral Bioavailability and Pharmacokinetics of Chemopreventive Kaempferol in Rats. Biopharm. Drug Dispos. 2009, 30(7), 356–365. 10.1002/bdd.677.
- DuPont, M.; Day, A.; Bennett, R.; Mellon, F.; Kroon, P. Absorption of Kaempferol from Endive, a Source of Kaempferol-3-Glucuronide, in Humans. Eur. J. Clin. Nutr. 2004, 58(6), 947–954. 10.1038/sj.ejcn.1601916.
- Taraphdar, A. K.; Roy, M.; Bhattacharya, R. Natural Products as Inducers of Apoptosis: Implication for Cancer Therapy and Prevention. Curr. Sci. 2001, 80(11), 1387–1396.
- Zhang, Y.; Chen, A. Y.; Li, M.; Chen, C.; Yao, Q. Ginkgo Biloba Extract Kaempferol Inhibits Cell Proliferation and Induces Apoptosis in Pancreatic Cancer Cells. J. Surg. Res. 2008, 148(1), 17–23.
- Song, W.; Dang, Q.; Xu, D.; Chen, Y.; Zhu, G.; Wu, K.; Zeng, J.; Long, Q.; Wang, X.; He, D. Kaempferol Induces Cell Cycle Arrest and Apoptosis in Renal Cell Carcinoma through EGFR/p38 Signaling. Oncol. Rep. 2014, 31(3), 1350–1356.
- Lee, J.; Kim, J. H. Kaempferol Inhibits Pancreatic Cancer Cell Growth and Migration through the Blockade of EGFR-Related Pathway in Vitro. PLoS ONE. 2016, 11(5), e0155264.
- Xu, K.; Shu, H.-K. G. EGFR Activation Results in Enhanced Cyclooxygenase-2 Expression through P38 Mitogen-Activated Protein Kinase–Dependent Activation of the SP1/SP3 Transcription Factors in Human Gliomas. Cancer Res. 2007, 67(13), 6121–6129.
- Cheng, J.-C.; Klausen, C.; Leung, P. C. Hydrogen Peroxide Mediates EGF-induced Down-Regulation of E-Cadherin Expression via P38 MAPK and Snail in Human Ovarian Cancer Cells. Mol. Endocrinol. 2010, 24(8), 1569–1580. Epub. 10.1210/me.2010-0034.
- Tang, X.-L.; Liu, J.-X.; Dong, W.; Li, P.; Li, L.; Hou, J.-C.; Zheng, Y.-Q.; Lin, C.-R.; Ren, J.-G. Protective Effect of Kaempferol on LPS Plus ATP-induced Inflammatory Response in Cardiac Fibroblasts. Inflammation. 2015, 38(1), 94–101. 10.1007/s10753-014-0011-2.
- Zang, Y.; Zhang, L.; Igarashi, K.; Yu, C. The Anti-Obesity and Anti-Diabetic Effects of Kaempferol Glycosides from Unripe Soybean Leaves in High-Fat-Diet Mice. Food Funct. 2015, 6(3), 834–841. 10.1039/c4fo00844h.
- Montero, M.; De La Fuente, S.; Fonteriz, R. I.; Moreno, A.; Alvarez, J. Effects of Long-Term Feeding of the Polyphenols Resveratrol and Kaempferol in Obese Mice. PLoS ONE. 2014, 9(11), e112825.
- García-Mediavilla, V.; Crespo, I.; Collado, P. S.; Esteller, A.; Sánchez-Campos, S.; Tuñón, M. J.; González-Gallego, J. The Anti-Inflammatory Flavones Quercetin and Kaempferol Cause Inhibition of Inducible Nitric Oxide Synthase, Cyclooxygenase-2 and Reactive C-Protein, and Down-Regulation of the Nuclear Factor kappaB Pathway in Chang Liver Cells. Eur. J. Pharmacol. 2007, 557(2), 221–229. 10.1016/j.ejphar.2006.11.014.
- Hossain, P.; Kawar, B.; El Nahas, M. Obesity and Diabetes in the Developing World—A Growing Challenge. N. Engl. J. Med. 2007, 356(3), 213–215. 10.1056/NEJMp068177.
- Song, Y.; Lee, S.-J.; Jang, S.-H.; Ha, J. H.; Song, Y. M.; Ko, Y.-G.; Kim, H.-D.; Min, W.; Kang, S. N.; Cho, J.-H. Sasa Borealis Stem Extract Attenuates Hepatic Steatosis in High-Fat Diet-Induced Obese Rats. Nutrients. 2014, 6(6), 2179–2195.
- Berger, A., Venturelli, S., Kallnischkies, M., Böcker, A., Busch, C., Weiland, T., Noor, S., Leischner, C., Weiss, T.S., Lauer, U.M., Bischoff, S.C., Bitzer, M. Kaempferol, a new nutrition-derived pan-inhibitor of human histone deacetylases. J NutrBiochem. 2013, 24(6), 977–985.
- Marfe, G.; Tafani, M.; Indelicato, M.; Sinibaldi‐Salimei, P.; Reali, V.; Pucci, B.; Fini, M.; Russo, M. A. Kaempferol Induces Apoptosis in Two Different Cell Lines via Akt Inactivation, Bax and SIRT3 Activation, and Mitochondrial Dysfunction. J. Cell. Biochem. 2009, 106(4), 643–650.
- Nguyen, T.; Tran, E.; Ong, C.; Lee, S.; Do, P.; Huynh, T.; Nguyen, T.; Lee, J.; Tan, Y.; Ong, C. Kaempferol‐Induced Growth Inhibition and Apoptosis in A549 Lung Cancer Cells Is Mediated by Activation of MEK‐MAPK. J. Cell. Physiol. 2003, 197(1), 110–121. 10.1002/jcp.10340.
- Tu, L.-Y.; Pi, J.; Jin, H.; Cai, J.-Y.; Deng, S.-P. Synthesis, Characterization and Anticancer Activity of Kaempferol-Zinc (II) Complex. Bioorg. Med. Chem. Lett. 2016, 26(11), 2730–2734. 10.1016/j.bmcl.2016.03.091.
- Escandón, R. A.; Del Campo, M.; López-Solis, R.; Obreque-Slier, E.; Toledo, H. Antibacterial Effect of Kaempferol and (−)-Epicatechin on Helicobacter Pylori. Eur. Food Res. Technol. 2016, 1–8. 10.1007/s00217-016-2650-z.
- Del Valle, P.; García-Armesto, M. R.; De Arriaga, D.; González-Donquiles, C.; Rodríquez-Fernández, P.; Rúa, J. Antimicrobial Activity of Kaempferol and Resveratrol in Binary Combinations with Parabens or Propyl Gallate against Enterococcus Faecalis. Food Control. 2016, 61, 213–220. 10.1016/j.foodcont.2015.10.001.
- Kai, H.; Obuchi, M.; Yoshida, H.; Watanabe, W.; Tsutsumi, S.; Park, Y. K.; Matsuno, K.; Yasukawa, K.; Kurokawa, M. In Vitro and in Vivo Anti-Influenza Virus Activities of Flavonoids and Related Compounds as Components of Brazilian Propolis (AF-08). J. Funct. Foods. 2014, 8, 214–223.
- Hassan, S. T.; Masarčíková, R.; Berchová, K. Bioactive Natural Products with Anti‐Herpes Simplex Virus Properties. J. Pharm. Pharmacol. 2015, 67(10), 1325–1336. Epub. 10.1111/jphp.12436.
- Wagner, H.; Bauer, R.; Melchart, D.; Staudinger, A. Herba Violae–Zihuadiding, in Chromatographic Fingerprint Analysis of Herbal Medicines Volume IV; Springer, Switzerland: 2016; 115–123.
- Behbahani, M.; Sayedipour, S.; Pourazar, A.; Shanehsazzadeh, M. In Vitro anti-HIV-1 Activities of Kaempferol and Kaempferol-7-O-glucoside Isolated from Securigera Securidaca. Res. Pharm. Sci. 2014, 9(6), 463–469. PMCID: PMC4326984.
- Park, U.-H.; Jeong, J.-C.; Jang, J.-S.; Sung, M.-R.; Youn, H.; Lee, S.-J.; Kim, E.-J.; Um, S.-J. Negative Regulation of Adipogenesis by Kaempferol, a Component of Rhizoma Polygonati Falcatum in 3T3-L1 Cells. Biol. Pharm. Bull. 2012, 35(9), 1525–1533.
- Mahat, M. Y. A.; Kulkarni, N. M.; Vishwakarma, S. L.; Khan, F. R.; Thippeswamy, B.; Hebballi, V.; Adhyapak, A. A.; Benade, V. S.; Ashfaque, S. M.; Tubachi, S. Modulation of the Cyclooxygenase Pathway via Inhibition of Nitric Oxide Production Contributes to the Anti-Inflammatory Activity of Kaempferol. Eur. J. Pharmacol. 2010, 642(1), 169–176.
- Kim, T. H.; Ku, S.-K.; Lee, I.-C.; Bae, J.-S. Anti-Inflammatory Effects of kaempferol-3-O-sophoroside in Human Endothelial Cells. Inflamm. Res. 2012, 61(3), 217–224. 10.1007/s00011-011-0403-9.
- Labbé, D.; Provençal, M.; Lamy, S.; Boivin, D.; Gingras, D.; Béliveau, R. The Flavonols Quercetin, Kaempferol, and Myricetin Inhibit Hepatocyte Growth Factor-Induced Medulloblastoma Cell Migration. J. Nutr. 2009, 139(4), 646–652. 10.3945/jn.108.102616.
- Bhat, K. P.; Kosmeder, J. W.; Pezzuto, J. M. Biological Effects of Resveratrol. Antioxid. Redox Signal. 2001, 3(6), 1041–1064. 10.1089/152308601317203567.
- Burns, J.; Yokota, T.; Ashihara, H.; Lean, M. E.; Crozier, A. Plant Foods and Herbal Sources of Resveratrol. J. Agric. Food Chem. 2002, 50(11), 3337–3340. PMID: 12010007.
- Harikumar, K. B.; Aggarwal, B. B. Resveratrol: A Multitargeted Agent for Age-Associated Chronic Diseases. Cell Cycle. 2008, 7(8), 1020–1035. 10.4161/cc.7.8.5740.
- Baur, J. A.; Sinclair, D. A. Therapeutic Potential of Resveratrol: The in Vivo Evidence. Nat. Rev. Drug Discov. 2006, 5(6), 493–506. 10.1038/nrd2060.
- Aguirre, L.; Fernández-Quintela, A.; Arias, N.; Portillo, M. P. Resveratrol: Anti-Obesity Mechanisms of Action. Molecules. 2014, 19(11), 18632–18655. 10.3390/molecules191118632.
- Olas, B.; Wachowicz, B.; Saluk-Juszczak, J.; Zieliński, T.; Kaca, W.; Buczyński, A. Antioxidant Activity of Resveratrol in Endotoxin-Stimulated Blood Platelets. Cell Biol. Toxicol. 2001, 17(2), 117–125.
- Dolinsky, V. W.; Jones, K. E.; Sidhu, R. S.; Haykowsky, M.; Czubryt, M. P.; Gordon, T.; Dyck, J. R. Improvements in Skeletal Muscle Strength and Cardiac Function Induced by Resveratrol during Exercise Training Contribute to Enhanced Exercise Performance in Rats. J. Physiol. 2012, 590(11), 2783–2799. Epub. 10.1113/jphysiol.2012.230490.
- Elmali, N.; Baysal, O.; Harma, A.; Esenkaya, I.; Mizrak, B. Effects of Resveratrol in Inflammatory Arthritis. Inflammation. 2007, 30(1–2), 1–6. 10.1007/s10753-006-9012-0.
- Palsamy, P.; Subramanian, S. Modulatory Effects of Resveratrol on Attenuating the Key Enzymes Activities of Carbohydrate Metabolism in Streptozotocin–Nicotinamide-Induced Diabetic Rats. Chem. Biol. Interact. 2009, 179(2), 356–362.
- Sun, A. Y.; Wang, Q.; Simonyi, A.; Sun, G. Y. Resveratrol as a Therapeutic Agent for Neurodegenerative Diseases. Mol. Neurobiol. 2010, 41(2–3), 375–383.
- Shin, J. A.; Lee, H.; Lim, Y.-K.; Koh, Y.; Choi, J. H.; Park, E.-M. Therapeutic Effects of Resveratrol during Acute Periods following Experimental Ischemic Stroke. J. Neuroimmunol. 2010, 227(1), 93–100.
- Bradamante, S.; Barenghi, L.; Villa, A. Cardiovascular Protective Effects of Resveratrol. Cardiovasc. Drug Rev. 2004, 22(3), 169–188. PMID: 15492766.
- Sun, W.; Wang, W.; Kim, J.; Keng, P.; Yang, S.; Zhang, H.; Liu, C.; Okunieff, P.; Zhang, L. Anti-Cancer Effect of Resveratrol Is Associated with Induction of Apoptosis via a Mitochondrial Pathway Alignment,AdvExp Med Biol. 2008, 614, 179–186.
- Sakagami, H.; Sheng, H.; Okudaira, N.; Yasui, T.; Wakabayashi, H.; Jia, J.; Natori, T.; Suguro-Kitajima, M.; Oizumi, H.; Oizumi, T. Prominent Anti-UV Activity and Possible Cosmetic Potential of Lignin-Carbohydrate Complex. In Vivo. 2016, 30(4), 331–339.
- Scalbert, A.; Manach, C.; Morand, C.; Rémésy, C.; Jiménez, L. Dietary Polyphenols and the Prevention of Diseases. Crit. Rev. Food Sci. Nutr. 2005, 45(4), 287–306.
- Trela, B. C.; Waterhouse, A. L. Resveratrol: Isomeric Molar Absorptivities and Stability. J. Agric. Food Chem. 1996, 44(5), 1253–1257. 10.1021/jf9504576.
- Delmas, D.; Aires, V.; Limagne, E.; Dutartre, P.; Mazué, F.; Ghiringhelli, F.; Latruffe, N. Transport, Stability, and Biological Activity of Resveratrol. Ann. N. Y. Acad. Sci. 2011, 1215(1), 48–59. 10.1111/j.1749-6632.2010.05871.x.
- Walle, T.; Hsieh, F.; DeLegge, M. H.; Oatis, J. E.; Walle, U. K. High Absorption but Very Low Bioavailability of Oral Resveratrol in Humans. Drug Metab. Dispos. 2004, 32(12), 1377–1382. 10.1124/dmd.104.000885.
- Walle, T. Bioavailability of Resveratrol. Ann. N. Y. Acad. Sci. 2011, 1215(1), 9–15.
- Vanamala, J.; Reddivari, L.; Radhakrishnan, S.; Tarver, C. Resveratrol Suppresses IGF-1 Induced Human Colon Cancer Cell Proliferation and Elevates Apoptosis via Suppression of IGF-1R/Wnt and Activation of P53 Signaling Pathways. BMC Cancer. 2010, 10(1), 238. 10.1186/1471-2407-10-238.
- Wang, -X.-X.; Li, Y.-B.; Yao, H.-J.; Ju, R.-J.; Zhang, Y.; Li, R.-J.; Yu, Y.; Zhang, L.; Lu, W.-L. The Use of Mitochondrial Targeting Resveratrol Liposomes Modified with a Dequalinium Polyethylene Glycol-Distearoylphosphatidyl Ethanolamine Conjugate to Induce Apoptosis in Resistant Lung Cancer Cells. Biomaterials. 2011, 32(24), 5673–5687.
- Filippi-Chiela, E. C.; Villodre, E. S.; Zamin, L. L.; Lenz, G. Autophagy Interplay with Apoptosis and Cell Cycle Regulation in the Growth Inhibiting Effect of Resveratrol in Glioma Cells. PLoS ONE. 2011, 6(6), e20849. 10.1371/journal.pone.0020849.
- Athar, M.; Back, J. H.; Tang, X.; Kim, K. H.; Kopelovich, L.; Bickers, D. R.; Kim, A. L. Resveratrol: A Review of Preclinical Studies for Human Cancer Prevention. Toxicol. Appl. Pharmacol. 2007, 224(3), 274–283. 10.1016/j.taap.2006.12.025.
- Jiang, H.; Shang, X.; Wu, H.; Gautam, S. C.; Al-Holou, S.; Li, C.; Kuo, J.; Zhang, L.; Chopp, M. Resveratrol Downregulates PI3K/Akt/mTOR Signaling Pathways in Human U251 Glioma Cells. J. Exp. Ther. Oncol. 2009, 8(1), 25–33.
- Kim, S.; Jin, Y.; Choi, Y.; Park, T. Resveratrol Exerts Anti-Obesity Effects via Mechanisms Involving Down-Regulation of Adipogenic and Inflammatory Processes in Mice. Biochem. Pharmacol. 2011, 81(11), 1343–1351.
- Timmers, S.; Konings, E.; Bilet, L.; Houtkooper, R. H.; Van De Weijer, T.; Goossens, G. H.; Hoeks, J.; Van Der Krieken, S.; Ryu, D.; Kersten, S. Calorie Restriction-Like Effects of 30 Days of Resveratrol Supplementation on Energy Metabolism and Metabolic Profile in Obese Humans. Cell Metab. 2011, 14(5), 612–622. 10.1016/j.cmet.2011.10.002.
- Smoliga, J. M.; Vang, O.; Baur, J. A. Challenges of Translating Basic Research into Therapeutics: Resveratrol as an Example. J. Gerontol. A Biol. Sci. Med. Sci. 2012, 67(2), 158–167.
- Ma, Z.-H.; Ma, Q.-Y.; Wang, L.-C.; Sha, H.-C.; Wu, S.-L.; Zhang, M. Effect of Resveratrol on Nf-Κb Activity in Rat Peritoneal Macrophages. Am. J. Chin. Med. (Gard City N Y). 2006, 34(04), 623–630. 10.1142/S0192415X06004156.
- Sharma, S.; Chopra, K.; Kulkarni, S.; Agrewala, J. Resveratrol and Curcumin Suppress Immune Response through CD28/CTLA‐4 and CD80 Co‐Stimulatory Pathway. Clin. Exp. Immunol. 2007, 147(1), 155–163. 10.1111/j.1365-2249.2006.03257.x.
- Renaud, J.; Martinoli, M. G. Prevention of Neuroinflammation by Resveratrol, in Neuroprotective Effects of Phytochemicals in Neurological Disorders, John Wiley & Sons, Inc, USA; 2017; 377–394.
- Bonnefont-Rousselot, D. Resveratrol and Cardiovascular Diseases. Nutrients. 2016, 8(5), 1–24. 10.3390/nu8050250.
- López-Nicolás, J. M.; García-Carmona, F. Aggregation State and P K a Values of (E)-Resveratrol as Determined by Fluorescence Spectroscopy and UV− Visible Absorption. J. Agric. Food Chem. 2008, 56(17), 7600–7605. 10.1021/jf800843e.
- Pezzuto, J. M. Resveratrol as an Inhibitor of Carcinogenesis. Pharm. Biol. 2008, 46(7–8), 443–573.
- Vang, O. Resveratrol: Challenges in Analyzing Its Biological Effects. Ann. N. Y. Acad. Sci. 2015, 1348(1), 161–170. 10.1111/nyas.12879.
- Barreiro-Hurlé, J.; Colombo, S.; Cantos-Villar, E. Is There a Market for Functional Wines? Consumer Preferences and Willingness to Pay for Resveratrol-Enriched Red Wine. Food Qual. Prefer. 2008, 19(4), 360–371. 10.1016/j.foodqual.2007.11.004.
- Taleb, H.; Morris, R. K.; Withycombe, C. E.; Maddocks, S. E.; Kanekanian, A. D. Date Syrup–Derived Polyphenols Attenuate Angiogenic Responses and Exhibits Anti-Inflammatory Activity Mediated by Vascular Endothelial Growth Factor and Cyclooxygenase-2 Expression in Endothelial Cells. Nutr. Res. 2016, 36(7), 636–647. 10.1016/j.nutres.2016.02.010.
- Leikert, J. F.; Räthel, T. R.; Wohlfart, P.; Cheynier, V.; Vollmar, A. M.; Dirsch, V. M. Red Wine Polyphenols Enhance Endothelial Nitric Oxide Synthase Expression and Subsequent Nitric Oxide Release from Endothelial Cells. Circulation. 2002, 106(13), 1614–1617. PMID: 12270851.
- Tapiero, H.; Tew, K.; Ba, G. N.; Mathe, G. Polyphenols: Do They Play a Role in the Prevention of Human Pathologies? Biomed. Pharmacother. 2002, 56(4), 200–207. PMID: 12109813.
- Rajan, S.; Ravi, J.; Suresh, A.; Guru, S. Hidden Secrets of ‘Punica Granatum’use and Its Effects on Oral Health: A Short Review. J. Dental Orofacial Res. 2013, 3(1), 38–41. 10.5005/jp-journals-10026-1061.
- Norusis, M. SPSS 16.0 Statistical Procedures Companion; Prentice Hall Press, Upper Saddle River, NJ, USA: 2008.
- Clatworthy, J.; Buick, D.; Hankins, M.; Weinman, J.; Horne, R. The Use and Reporting of Cluster Analysis in Health Psychology: A Review. Br. J. Health Psychol. 2005, 10(3), 329–358. 10.1348/135910705X25697.
- Sharplin, A. D.; Mabry, R. H. The Relative Importance of Journals Used in Management Research: An Alternative Ranking. Hum. Relations. 1985, 38(2), 139–149.
- Momen, H. The Role of Journals in Enhancing Health Research in Developing Countries. Bull. World Health Organ. 2004, 82, 163–163. PMCID: PMC2585931.
- Yao, L. H.; Jiang, Y.; Shi, J.; Tomas-Barberan, F.; Datta, N.; Singanusong, R.; Chen, S. Flavonoids in Food and Their Health Benefits. Plant Foods Hum. Nutr. 2004, 59(3), 113–122. 10.1007/s11130-004-0049-7.
- Hertog, M. G.; Hollman, P. C.; Katan, M. B. Content of Potentially Anticarcinogenic Flavonoids of 28 Vegetables and 9 Fruits Commonly Consumed in the Netherlands. J. Agric. Food Chem. 1992, 40(12), 2379–2383. 10.1021/jf00024a011.
- Balentine, D. A.; Dwyer, J. T.; Erdman, J. W.; Ferruzzi, M. G.; Gaine, P. C.; Harnly, J. M.; Kwik-Uribe, C. L. Recommendations on Reporting Requirements for Flavonoids in Research. Am. J. Clin. Nutr. 2015, 101(6), 1113–1125. 10.3945/ajcn.113.071274.
- Jones, E.; Hughes, R. Quercetin, Flavonoids and the Life-Span of Mice. Exp. Gerontol. 1982, 17(3), 213–217. PMID: 7140862.
- Gleichenhagen, M.; Schieber, A. Current Challenges in Polyphenol Analytical Chemistry. Curr. Opin. Food Sci. 2016, 7, 43–49. 10.1016/j.cofs.2015.10.004.
- Vauzour, D. Dietary Polyphenols as Modulators of Brain Functions: Biological Actions and Molecular Mechanisms Underpinning Their Beneficial Effects. Oxid. Med. Cell. Longev. 2012, 2012, 1–16. 10.1155/2012/914273.
- Rossi, L.; Mazzitelli, S.; Arciello, M.; Capo, C.; Rotilio, G. Benefits from Dietary Polyphenols for Brain Aging and Alzheimer’s Disease. Neurochem. Res. 2008, 33(12), 2390–2400.
- Obied, H. K. Biography of Biophenols: Past, Present and Future. Funct. Foods Health Disease. 2013, 3(6), 230–241.
- Halliwell, B. Are Polyphenols Antioxidants or Pro-Oxidants? What Do We Learn from Cell Culture and in Vivo Studies? Arch. Biochem. Biophys. 2008, 476(2), 107–112. 10.1016/j.abb.2008.01.028.
- Halliwell, B. Free Radicals and Antioxidants–Quo Vadis? Trends Pharmacol. Sci. 2011, 32(3), 125–130. 10.1016/j.tips.2010.12.002.
- Duarte, T. L.; Lunec, J. Review: When Is an Antioxidant Not an Antioxidant? A Review of Novel Actions and Reactions of Vitamin C. Free Radic. Res. 2005, 39(7), 671–686. 10.1080/10715760500104025.
- Yordi, E. G.; Pérez, E. M.; Matos, M. J.; Villares, E. U. Antioxidant and Pro-Oxidant Effects of Polyphenolic Compounds and Structure-Activity Relationship Evidence; Nutrition, Well-being and health, London : 2012; 23–48.
- Carocho, M.; Ferreira, I. C. A Review on Antioxidants, Prooxidants and Related Controversy: Natural and Synthetic Compounds, Screening and Analysis Methodologies and Future Perspectives. Food Chem. Toxicol. 2013, 51, 15–25. 10.1016/j.fct.2012.09.021.
- Galati, G.; O’brien, P. J. Potential Toxicity of Flavonoids and Other Dietary Phenolics: Significance for Their Chemopreventive and Anticancer Properties. Free Radic. Biol. Med. 2004, 37(3), 287–303. 10.1016/j.freeradbiomed.2004.04.034.
- Lacroix, S.; Lauria, M.; Scott-Boyer, M.-P.; Marchetti, L.; Priami, C.; Caberlotto, L. Systems Biology Approaches to Study the Molecular Effects of Caloric Restriction and Polyphenols on Aging Processes. Genes Nutr. 2015, 10(6), 1–10. 10.1007/s12263-015-0508-9.