Abstract
The current investigation was carried out to examine the role of arbuscular mycorrhizal fungi (AMF) in alleviating adverse effects of salt stress in Ephedra aphylla. The plants were exposed to 75 and 150 mM sodium chloride (NaCl) stress with and without application of AMF. Salt stress caused significant decrease in chlorophyll and carotenoid contents; however, the application of AMF restored the pigments content in salt-affected plants. Proline, phenols, and lipid peroxidation were increased with increasing concentration of NaCl, but lower accumulation has been reported in plants treated with AMF. NaCl stress also showed increase in different antioxidant enzymes activities (catalase, ascorbate peroxidase, peroxidase, glutathione reductase, and superoxide dismutase), and further increase was observed in plants treated with AMF. The nutrient uptake, Na+ and Na/K ratio increased and potassium and phosphorus were decreased with increasing concentration of NaCl in the present study. However, the colonization with AMF significantly increased K+ and P and reduced Na+ uptake. It is concluded that presown soil treatment with AMF reduced severity of salt stress in E. aphylla through alterations in physiological parameters, antioxidants and uptake of nutrients.
Introduction
Desertification or the disappearance of vegetation from arid land is an increasing problem worldwide including Saudi Arabia (SA). Abiotic stress leads to loss of natural resources such as plant biomass, biodiversity, and imparts a great role in global warming. Among the abiotic stresses, salinity is one of the main factor that contributes to desertification of arid lands. Soil salinization has become a great challenge for rehabilitation of range lands and plant productivity (Alqarawi et al. Citation2014). Salinity not only reduces yield of plants but also disrupts the ecological balance of the area. Stress tolerance of plants is a complex phenomenon involving changes at morphological, physiological, biochemical, and molecular levels and are undoubtedly the most important response of plants to the adverse environmental conditions (Rasool, Hameed, et al. Citation2013; Shaheen et al. Citation2013; Ahmad et al. Citation2014; Alqarawi et al. Citation2014). Salinity causes nutritional disorders in plants which lead to deficiencies of several nutrients and drastically increasing Na+ levels in the plant cells (Iqbal & Ashraf Citation2013).
The adverse effects of salt stress causes imbalance in the synthesis of endogenous plant growth regulators (PGR), hence a number of changes take place which disturb growth and physiological processes such as plant cell permeability, respiration, energy transport, and photosynthesis in plant cells (Ahmad et al. Citation2012; Iqbal & Ashraf Citation2013). Moreover, the salt stress also causes oxidative stress through the generation of reactive oxygen species (ROS), such as hydrogen peroxide, superoxide ions, singlet oxygen, and hydroxyl radical. These ROS cause deleterious effects on mitochondria, chloroplast, membranes, etc., by disturbing cellular structures in plant cells (Ahmad, Jaleel, Salem, et al. Citation2010, Ahmad et al. Citation2012). However, plants are equipped with antioxidant machinery which includes, superoxide dismutase, catalase, ascorbate peroxidase, and glutathione reductase (Ahmad, Jaleel, and Sharma Citation2010; Rasool, Ahmad, et al. Citation2013). These antioxidants scavenge the ROS and provide resistance to the plants against NaCl stress.
Range plants are considered the most important natural sources in SA. These plants face several biotic and abiotic challenges to growth and reproduction. Ephedra aphylla Forssk. (family Ephedraceae) is a genus of nonflowering seed plants which is an erect shrub up to 1.5 m high, stems usually rough and leaves small (3 mm long). The habitat of E. aphylla is shallow dry runnels in limestone. The shoots of E. aphylla contain the alkaloid ephedrine and are valuable in the treatment of asthma and many other complaints of the respiratory system (Alqarawi et al. Citation2014).
Plants grown in fields are surrounded by various microorganisms like bacteria and fungi that help and improve the plant growth and yield under various stress conditions (Creus et al. Citation1998). Scientists are looking forward for the alternatives to enhance the crop production under the salt-affected soils. The usage of AMF as a biologically based strategy to alleviate the adverse impact induced by salt is of such alternatives. AMF act as growth regulator and mitigate the harmful effects of plants exposed to salt stress. AMF play a key role in alleviating the toxicity induced by salt stress thus normalizing the uptake mechanism in plants by supplying the essential nutrients. In this way, the plant recovers the water balance machinery, enhancing their tolerance capacity, and thereby enduring the salt stress (Bhosale & Shinde Citation2011). AMF supply mineral nutrients to plants, especially phosphorous, which is precipitated by ions like Ca, Mg, Zn (Al-Karaki Citation2000). The objective of this study was to observe the effect of AMF on growth and some metabolic process of E. aphylla under saline and nonsaline conditions.
Materials and methods
The experimental plant and soil
The seeds of E. aphylla Forssk. (Synonym name: Ephedra alte C.A.Mey.) used in the present experiment were collected from Derab rangelands, Riyadh, SA, during seed production season of 2012. The seeds were surface sterilized with sodium hypochlorite (0.5%, v/v) for 3 min, washed thoroughly with distilled water before germination on blotter. The experiment was carried out in sandy soil collected from Derab Agricultural Research Station, Riyadh, SA. The physical and chemical properties of the soil were measured according to Brady and Weil (Citation2002). The soil used throughout this investigation was sandy loam with the following properties (%): sand (84.3); clay (8.2); silt (7.5); moisture content, 4.23; organic carbon, 0.17; total nitrogen, 0.007; (EC) = 7.12 dS/m; and pH 7.8 (Carter & Gregorich Citation2008). The soil was autoclaved for 3 h at 121°C, cooled down, and then divided among plastic pots (150 g capacity). Hoagland’s solution supplemented with different concentrations of NaCl to get concentration of 0, 75, and 150 mM was used for irrigation.
Mycorrhizal inoculum
The AMF used in the present study were isolated previously from salt march habitat soil [(EC) = 9.2 dS m–1] sown with maize (Zea mays) plants in Alserw, Dakahlia, Egypt. The extraction and quantification of AMF were carried by wet sieving, decanting, and sucrose density gradient centrifugation techniques as described by Daniels and Skipper (Citation1982) and modified by Utobo et al. (Citation2011). The extracted spores were collected on filter paper (Whatman No.1), and the morphologically similar spores were picked up for identification according to Bethemfalvay and Yoder (Citation1981) and Schenck and Perez (Citation1990) based on spore color, shape, surface ornamentation, spore contents, and wall structures. The selected AMF (Funneliformis mosseae [syn. Glomus mosseae]; Rhizophagus intraradices [syn. Glomus intraradices], and Claroideoglomus etunicatum [syn. Glomus etunicatum]) were inoculated singly with maize (Zea mays) plants using autoclaved soil (clay:sand, 1:1, w/w) as a host plant for three generations in pot cultures in a greenhouse for propagation of fungal spores. The growth conditions were 25°C day, 20°C night temperatures, 65% relative humidity, 16/8 h light/dark photoperiod cycle with a photosynthetic photon flux density of (750 µmol m–2 S−1). Hoagland’s solution without phosphorus source (free phosphorus) was used for irrigation to enhance mycorrhizal colonization. AM fungal spores were extracted from the trap cultures as described above to develop pure AM fungal cultures. Fungal inoculums potential was determined by the most probable numbers method (Alexander Citation1982) and each trap culture contained ~10.2 × 103 propagules per pot (1 kg capacity). Fungal inocolum consisted of AM fungal spores, hyphae, and colonized root fragments. The mycorrhizal inocolum was added to the experimental soil as 10 g of trap soil culture (approximately 100 spores/g trap soil, M = 80%)/pot (1 kg). Nonmycorrhizal soil was used as reference.
Pot experiment
The pot experiment was carried out in a growth chamber by split-plot in randomized complete block design with five replications. Hoagland’s solution (Hoagland & Arnon Citation1950) supplemented with different concentrations of sodium chloride to get concentration of 0, 75 and 150 mM was used for irrigation in normal pots as well as in pots with mycorrhizal inocolum. The rate of irrigation was 100 ml for each treatment every 3 day for eight weeks at alternative temperature rate of (27°C day and 18°C night) with a photosynthetic photon flux density of 1500 µmol m–2 s−1 (18 h light and 6 h dark period). At the end of experiment period (8 weeks), the plants were harvested for growth and biochemical estimation.
Determination of photosynthetic pigments
The photosynthetic pigments (chlorophyll a [Chl a], chlorophyll b [Chl b], and carotenoids,) were extracted from leaves of E. aphylla plants in dimethyl sulfoxide (DMSO) as described by Hiscox and Israelstam (Citation1979). Absorbance was determined spectrophotometrically at 480, 510, 645, and 663 nm (T80 UV/VIS Spectrometer, PG Instruments Ltd, USA). DMSO was used as blank.
Determination of proline
The proline extracted from leaf samples (0.5 g) using sulfosalicylic acid (MP, Biomedicals, Inc.) solution (3%; w/v) and estimated by acid ninhydrin solution according to Bates et al. (Citation1973). The OD of the filtrate was measured at 520 nm. The toluene was used as blank.
Determination of total phenolics
The total phenolics in the shoot system were extracted with 80% (v/v) acetone and estimated using (20%, w/v) sodium carbonate (Na2CO3) and Folin and Ciocalteau’s phenol reagent following Julkunen-Tiitto (Citation1985). The ODs of the mixtures were read at 750 nm. Standard curve of pyrogallol was used as reference.
Determination of lipid peroxidation
The method of Heath and Packer (Citation1968) was used to determine the lipid peroxidation in terms of change in malondialdehyde (MDA) content. In this method, fresh leaves (300 mg) were ground in 1% (w/v) trichloro acetic acid (TCA). The homogenate was centrifuged at 10,000 rpm for 5 min. The reaction mixture contains 1.0 ml of supernatant and 4.0 ml of 0.5% (w/v) thiobarbituric acid (TBA). The mixture was heated at 95°C for 30 min, cooled in an ice bath, and centrifuged at 5000 rpm for 5 min for clarification. Absorbance at 532 and 600 nm were used for calculation of MDA equivalents. Blank sample was used as reference.
Determination of antioxidant enzyme activities
Fresh leaves (10 g) were homogenized in 50 mM sodium phosphate buffer (pH 7.0) containing 1% (w/v) PVP-40 (Polyvinylpyrrolidone). The homogenate was centrifuged at 15,000 rpm for 20 min at 4°C, and the supernatant was completed to known volume with sodium phosphate buffer and was used for the assays of enzyme activity. Proteins were estimated in the enzyme extract using Folin reaction according to Lowry et al. (Citation1951).
The activity of ascorbate peroxidase APX (EC 1.11.1.11) was assayed according to the method of Nakano and Asada (Citation1981). The reaction mixture contained 1.0 ml of reaction buffer (25 mM potassium phosphate [pH 7.0] with 0.1 mM EDTA, 0.5 mM ascorbate, 0.1 mM H2O2, and 0.1 ml of enzyme extract). APX was assayed as a decrease in absorbance at 290 nm of ascorbate. APX activity was expressed as unit mg−1 protein. For the calculation of APX enzyme activity, the extinction coefficient of 2.8 mM−1 cm−1 was used. One unit of enzyme was considered as the amount necessary to decompose 1 mol of substrate per min at 25°C.
The method of Foyer and Halliwell (Citation1976) was used to estimate the activity of glutathione reductase (GR; EC 1.6.4.2). In this method, the assay mixture contained 50 mM Na-phosphate buffer (pH 7.8), 0.12 mM nicotinamide adenine dinucleotide phosphate (NADPH), 0.5 mM oxidized glutathione (GSSG), and 0.1 ml of the enzyme extract in a total volume of 1.0 mL. NADPH oxidation was followed at 340 nm for 2 min. GR activity was expressed as l mol NADPH oxidized min−1 (units mg−1 protein). GR activity was calculated using the extinction coefficient for NADPH of 6.2 mM−1 cm−1. The specific enzyme activities were expressed as EU mg−1 protein.
Peroxidase (POD; EC 1.11.1.7) activity was assayed spectrophotometrically according to the method of Chance and Maehly (Citation1955). The reaction mixture contained 750 μL phosphate buffer (50 mM; pH 5.0), 100 μL guaiacol (20 mM); 100 μL H2O2 (40 mM); and 100 μL enzyme extract. OD at 470 nm of the reaction solution was read for 3 min after every 20 sec. The enzyme activity was expressed as EU mg−1 protein.
The catalase (CAT, EC 1.11.1.6) was estimated according to the method of Chance and Maehly (Citation1955). The reaction mixture (2 mL) contained (1.9 mL phosphate buffer [50 mM; pH 7.0] and 0.1 mL H2O2 [5.9 mM]), and the reaction was initiated by dissolving an aliquot (100 μL) of the enzyme extract. OD of the mixture was read spectrophotometrically at 240 nm for 2 min after every 20 seconds. Per unit CAT enzyme activity was based on change in OD per min. Enzyme activity was expressed as EU mg−1 protein.
Superoxide dismutase (SOD; EC 1.15.1.1) activity was assayed by measuring its ability to inhibit the photochemical reduction of nitroblue tetrazolium adopting the method of Giannopolitis and Ries (Citation1977). The reaction mixture consisted of 50 mM phosphate buffer (pH 7.8), 13 mM methionine, 60 μM nitroblue tetrazolium (NBT), 0.1 mM EDTA, and 100 μl enzyme extract. The reaction mixture was placed 30 cm below light source consisting of two 20 W fluorescent lamps for 20 min. A tube with protein kept in the dark served as a blank, while the control tube was with boiled enzyme and kept in the light. The absorbance was measured at 540 nm. The activity of SOD is the measure of NBT reduction in light without protein minus NBT reduction in light with protein. One unit of activity is the amount of enzyme required to inhibit 50% initial reduction of NBT under light. The results were expressed as EU mg−1 protein.
Determination of ions
The oven-dry shoot samples (at 110°C for 24 h) were digested, and the ions Na+ and K+ were estimated according to the method of Wolf (Citation1982) using a flame photometer Jenway Flame Photometer, Bibby Scientific Ltd-Stone-Staffs-St15 0SA, UK. The Phosphorus (P) was extracted by nitric-perchloric acid digestion and measured using the Vanado-molybdophosphoric colorimetric method (Jackson Citation1962). Standard curve of each mineral (10–100 µg/ml) was used as reference.
Statistical analysis
Two-way analysis (ANOVA) was used for statistical analysis followed by Duncan’s Multiple Range Test (DMRT). The values obtained were the mean ± SE for five replicates in each group. P-value at 0.05 was considered as significant.
Results
The salt stress caused significant decrease in all the growth parameters such as shoot length, shoot dry weight, root length, and root dry weight in E. aphylla (). The rate of decrease was directly proportional to the salt concentrations. Maximum decrease of 50.17% and 64.26% in shoot and root length, respectively, was observed at 150 mM NaCl. Dry weight of shoot decreased to 60.86% and root to 85.71% at the same concentration of NaCl. The use of AMF alleviated the negative effect of salinity on the growth and biomass yield significantly. Minimum decrease of 39.54% in shoot length, 53.57% in root length, 60.86% in shoot dry weight, and 47.05% in root dry weight was observed at 150 mM NaCl in combination with AMF.
Table 1. Effect of NaCl in the presence and absence of AMF on growth and biomass yield of E. aphylla seedlings.
Salt stress effectively decreased Chl a, Chl b and total Chl of E. aphylla as compared to control (). The salt concentrations 75 mM and 150 mM caused significant decrease in Chl a to 53.06% and 67.34%, respectively, in absence of AMF and 27.72% at 75 mM and 53.46% at 150 mM in presence of AMF. Maximum decrease in Chl b, carotenoids, and total pigments was 79.41%, 75.00%, and 72.52% at 150 mM NaCl. NaCl (150 mM) in combination with AMF showed decrease of 68.25% in Chl b, 61.90% in carotenoids, and 59.45% in total pigments as compared to the NaCl treatment alone. The Chl a/b ratio showed increase of 36.84% and 31.91% at 150 mM NaCl treated without and with AMF, respectively, as compared to control.
Table 2. Effect of NaCl in the presence and absence of AMF on photosynthetic pigments of E. aphylla seedlings.
The results related to the effect of different concentrations of NaCl on proline, total phenols, and lipid peroxidation (MDA) in E. aphylla are presented in . Salinity at concentration 75 mM significantly increased the proline, total phenol, and MDA contents in E. aphylla to 46.91%, 68.63%, and 68.67%, respectively, as compared to control. At 150 mM, further increase in proline, total phenols, and lipid peroxidation (MDA) was observed in directly proportional with salt stress. Application of AMF have shown further increase in proline content and total phenols and decrease in MDA content means that AMF decreased the adverse effect of salinity in E. aphylla.
Table 3. Effect of NaCl in the presence and absence of AMF on proline content (µmol g−1 fr. wt.); Total phenols (mg g−1 fr. wt.), and malondialdehyde (MDA) contents (μmol g−1 fr.wt.) of E. aphylla seedlings.
Data in indicated that salt stress caused significant increase in activity of all antioxidant enzymes in E. aphylla. A significant increase in activity of antioxidant enzymes such as CAT, APX, POD, GR, and SOD by 33.55%, 20.15%, 20.90%, 25.00%, and 26.53%, respectively, was observed at 75 mM NaCl stress as compared to control. Further increase in the antioxidant enzymes has been reported at 150 mM as compared to 75 mM NaCl and control. Salt-treated plants in combination with AMF further increases the antioxidant activity in present study. Application of AMF effectively caused more enhancement in antioxidant enzyme activities at both concentrations of salt stress. In comparison to control, an increase of 52.48% in CAT, 47.21% in APX, 42.90% in POD, 51.62% in GR, and 55.37% in SOD as compared to control was observed with combined effect of NaCl (150 mM) and AMF.
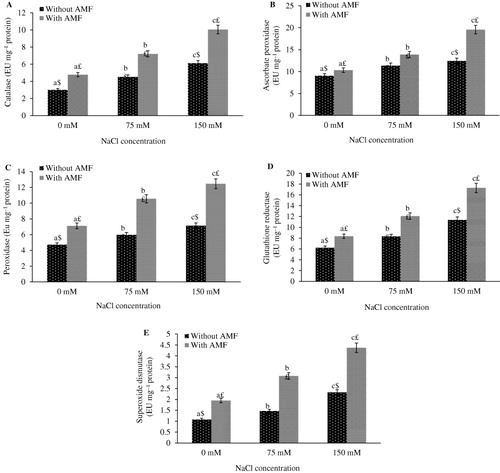
The shoot sodium content of E. aphylla was increased to 30.57% and 47.75% with 75 mM and 150 mM NaCl, respectively, in the absence of AMF. However, such salt concentrations hamper potassium (K+) uptake significantly; hence, Na/K ratio is directly proportional to increased salt concentration (). Salt stress (150 mM) caused significant decrease of 54.69% and 58.98% in potassium and phosphorus content, respectively, as compared to control. The treatment of soil with AMF was very effective in alleviating the deleterious effects of salinity stress by increasing K/Na ratio in plants. AMF-treated plants in combination with 150 mM NaCl showed less decrease of 32.18% in K+ content and 41.19% in P content as compared to salt-treated plants alone and control.
Table 4. Effect of NaCl in the presence and absence of AMF on ion accumulation of E. aphylla seedlings.
Discussion
In the present study, E. aphylla subjected to different concentrations of salt stress showed decreased growth and biomass yield at all NaCl concentrations. The decrease in growth is in accordance with the studies on Salsola villosa (Assaeed Citation2001) and Panicum antidotale (Ahmad, Ashraf, et al. Citation2010). Delay in plant growth under saline conditions was attributed to decrease in osmotic potential of the soil solution consequently and water uptake by plant root (Rasool, Hameed, et al. Citation2013). Also salinity affected many metabolic processes, which lead to retarded growth and development of plants (Iqbal & Ashraf Citation2013; Rasool, Ahmad, et al. Citation2013). The application of AMF alleviate the adverse effect of salt stress in present study and has also been reported by many researchers (Bhosale & Shinde Citation2011; Evelin & Kapoor Citation2013). AMF help in uptake of minerals and water from the soil and increases the osmotic potential of the soil solution. Moreover, AMF have been reported to produce plant growth hormones that have beneficial effects on plant growth under salt stress (Iqbal & Ashraf Citation2013).
The harmful effect of salinity on photosynthetic pigments has been reported in many plants such as Ocimum basilicum (Heidari Citation2012) and Cicer arietinum (Rasool, Ahmad, et al. Citation2013). The reduction in the pigment content is attributed to the destructive effect of salt stress on chloroplast (Zörb et al. Citation2009) and increased activity of chlorophyll-degrading enzymes such as chlorophyllase (Sultana et al. Citation1999). However, AMF-associated plants showed improvement in photosynthetic pigments under salt stress. Bhosale and Shinde (Citation2011) and Arya and Buch (Citation2013) also reported that AMF increased chlorophyll contents of Zingiber officinale and Gossypium herbaceum, respectively, under salt stress suggesting the less interference of salt with chlorophyll biosynthesis. Chlorophyll activity is restored in mycorrhizal plants grown under salt stress due to increased activity of specific enzymes required for its biosynthesis (Kadian et al. Citation2013).
Salt stress considerably triggered accumulation of free proline in E. aphylla. Increase in proline accumulation is reported as an adaptive response to salinity in different plants such as sugar beet (Farkhondeh et al. Citation2012) and tobacco (Cęlik & Atak Citation2012). This increment in proline could be due to the induction of proline biosynthesis enzymes and/or to the reduction of oxidation to glutamate (Stewart Citation1981). It is well evident that increased accumulation of proline plays a significant role in the protection of various vital enzymes, membranes, and proteins in the plant cells. Proline has a key role in scavenging ROS (Miller et al. Citation2010), osmotic adjustment (Sheng et al. Citation2008), and facilitating water uptake (Hassine & Lutts Citation2010). In the present study, proline content in mycorrhizal E. aphylla was significantly higher than that in control (nonmycorrhizal plants) grown in saline soil. Other researchers have also reported the increase of proline content in different plants grown under salt stress (Bhosale & Shinde Citation2011; Ahmad et al. Citation2014). Production of proline by the application of AMF in wheat demonstrates the high tolerance capacity by stabilizing the osmotic balance and scavenging the toxic radicals (García-Garrido & Ocampo Citation2002).
Phenolic compounds enhance protection against biotic and abiotic stresses by acting as a potential antioxidant particularly to scavenge singlet oxygen (Rice-Evans et al. Citation1997; Tsao Citation2010; Pohjala & Tammela Citation2012). An increase in phenolic compounds in the present study under salt stress are in agreement with the reports of Lim et al. (Citation2012); Mehr et al. (Citation2012) and Petridis et al. (Citation2012) in Fagopyrum esculentum, Anethum graveolens, and Olea europaea, respectively. Treatment with AMF further increased the total phenolic content in the present study. AMF have the potential to stimulate the host plants to produce their own defence compounds. Phenolic compounds have antioxidant properties that scavenge the free radicals produced during oxidative stress induced by NaCl.
Lipid peroxidation expressed as MDA content significantly increased in E. aphylla grown under salt stress. Our results are supported by earlier studies on Triticum aestivum (Ashraf et al. Citation2010), Rosmarinus officinalis (Hejazi et al. Citation2012), and C. arietinum (Rasool, Ahmad, et al. Citation2013). Recently, Ghogdi et al. (Citation2012) also reported similar effects of salinity on MDA content on wheat. The application of AMF caused significant decrease in MDA content compared with control in the present study. Reduced contents of MDA is an important indicator of stress tolerance as reported by Ahmad, Jaleel, and Sharma (Citation2010) in mulberry, Rasool, Ahmad, et al. (2Citation013) in chickpea, and Ahmad et al. (Citation2012) in mustard. The ROS generated through oxidative stress reacts with membrane lipids and causes lipid peroxidation (Ahmad, Jaleel & Sharma Citation2010; Ahmad et al. Citation2012; Rasool, Ahmad, et al. Citation2013). Treatment with AMF enhanced the activity of antioxidants, which quenches the ROS and protects the membranes from further damage. AMF have been found to up-regulate the stress-related proteins like glutathione S-transferase (GST), glutathione dependent formaldehyde dehydrogenase (FALDH), and peroxidase. These proteins are also able to scavenge the ROS and protect the cell membranes and other organelles from damage (Rasool, Hameed, et al. Citation2013).
A marked increase in activity of antioxidant defence enzymes such as CAT, APX, POD, GR, and SOD of E. aphylla under saline conditions was observed in present study. The contributions of these antioxidants against salt stress is also reported by Nounjan et al. (Citation2012) in rice, Rasool, Ahmad, et al. (Citation2013) in chickpea, Ahmad, Ashraf, et al. (Citation2010), Ahmad, Jaleel, Salem, et al. (Citation2010) and Ahmad, Jaleel, and Sharma (Citation2010) in mulberry, and Ahmad et al. (Citation2012) in mustard. Generally, accumulation of antioxidants plays an important role in regulating the oxidative stress and ROS production during the growth under salt-stress conditions (Rasool, Hameed, et al. Citation2013). Our results related to the effect of AMF on antioxidants under salt stress in E. aphylla corroborated with the findings of Wu et al. (Citation2010) in Poncirus trifoliate. He et al. (Citation2007) in Solanum lycopersicum and Huang et al. (Citation2008) in Avena nuda also showed increase in antioxidant activity in plants colonized by AMF under NaCl. Arbuscular mycorrhizas themselves possessed various SOD genes to up-regulate stress tolerance and decrease the accumulation of H2O2 as compared to nonmycorrhizal plants, indicating lower oxidative damage in the colonized plants (Wu et al. Citation2010; Hajiboland et al. Citation2012).
High accumulation of Na+ associated with a marked suppression of both K+ and phosphorus contents due to NaCl was observed in present study. Ionic imbalance is considered as one of the main effect of salinity stress in many plants (Zheng et al. Citation2008; Bhosale & Shinde Citation2011; Iqbal & Ashraf Citation2013). The ionic balance has a key role in photosynthesis and other metabolic activities of the cell (Zheng et al. Citation2008). The treatment of soil with AMF were very effective in alleviating the deleterious effects of salinity stress on ionic balance of E. aphylla and mitigates the deficiency of K+ and increases K/Na ratio at all levels of salt stress. Similar results have been reported by other researchers (Mohammad et al. Citation2003; Audet & Charest Citation2006). Al-Karaki (Citation2000) also reported that AM fungal symbiosis plays a key role in higher K+ accumulation and hence higher K/Na ratio in mycorrhizal plants. AMF spread the mycelium deep into the soil and absorb water and minerals for the host plant so increase the efficiency of the plants to withstand the NaCl stress.
In conclusion, NaCl stress negatively affects growth and biomass yield and other physiological parameters; however, association with AMF mitigates the negative effect and seem to enhance plant growth through the accumulation of different solutes and enhanced enzyme activity. Moreover, mycorrhizal inoculation can improve nutrient status of plants by increasing absorption area of root systems and enhancing uptake of water and nutrients from soil through the extraradical hyphae. Antioxidant enzyme activity proved to be the main defense for E. aphylla against salt stress in present study. This will be an alternative way of increasing tolerance against the adverse effects of salt stress.
Acknowledgment
The authors would like to extend their sincere appreciation to the Deanship of Scientific Research at King Saud University for its funding of this research through the Research Group Project no RGP-VPP-271.
References
- Ahmad MS, Ashraf M, Ali Q. 2010. Soil salinity as a selection pressure is a key determinant for the evolution of salt tolerance in Blue Panic grass (Panicum antidotale Retz.). Flora. 205:37–45. 10.1016/j.flora.2008.12.002
- Ahmad P, Ashraf M, Hakeem KR, Azooz MM, Rasool S, Chandna R, Akram NA. 2014. Potassium starvation-induced oxidative stress and antioxidant defense responses in Brassica juncea. J Plant Interact. 9:1–9. 10.1080/17429145.2012.747629
- Ahmad P, Hakeem KR, Kumar A, Ashraf M, Akram NA. 2012. Salt-induced changes in photosynthetic activity and oxidative defense system of three cultivars of mustard (Brassica juncea L.). Afr J Biotechnol. 11:2694–2703.
- Ahmad P, Jaleel CA, Salem MA, Nabi G, Sharma S. 2010. Roles of enzymatic and nonenzymatic antioxidants in plants during abiotic stress. Crit Rev Biotechnol. 30:161–175. 10.3109/07388550903524243
- Ahmad P, Jaleel CA, Sharma S. 2010. Antioxidant defense system, lipid peroxidation, proline metabolizing enzymes, and biochemical activities in two Morus alba genotypes subjected to NaCl stress. Russian J Plant Physiol. 57:509–517.
- Alexander M. 1982. Most probable number method for microbial populations. In: Black CA, editor. Methods of soil analysis. Madison (WI): American Society of Agronomy; p. 815–820.
- Al-Karaki GN. 2000. Growth of mycorrhizal tomato and mineral acquisition under salt stress. Mycorrhiza. 10:51–54. 10.1007/s005720000055
- Alqarawi AA, Hashem A, Abd-Allah EF, Alshahrani TS, Huqail AA. 2014. Effect of salinity on moisture content, pigment system, and lipid composition in Ephedra alata Decne. Acta Biol Hung. 65:61–71. 10.1556/ABiol.65.2014.1.6
- Arya A, Buch H. 2013. Response of arbuscular mycorrhizal fungi on growth and chlorophyll content of three varieties of Gossypium herbaceum L. Plant Pathol Quar. 3:54–57.
- Ashraf MA, Ashraf M, Ali Q. 2010. Response of two genetically diverse wheat cultivars to salt stress at different growth stages: leaf lipid peroxidation and phenolic contents. Pak J Bot. 42:559–565.
- Assaeed AM 2001. Effect of temperature and water potential on germination of Salsola villosa DEL. EX ROEM Et Schult. Assiut J Agric Sci. 32:173–183.
- Audet P, Charest C. 2006. Effects of AM colonization on “wild tobacco” plants grown in zinc-contaminated soil. Mycorrhiza. 16:277–283. 10.1007/s00572-006-0045-x
- Bates LS, Waldren RP, Teare ID. 1973. Rapid determination of free proline for water stress studies. Plant Sci. 39:205–207.
- Bethemfalvay GJ, Yoder JF. 1981. The Glycine-Glomus-Rhizobium symbiosis. I. Phosphorus effect on nitrogen fixation and mycorrhizal infection. Physiol Plant. 52:141–145. 10.1111/j.1399-3054.1981.tb06047.x
- Bhosale KS, Shinde BP. 2011. Influence of arbuscular mycorrhizal fungi on proline and chlorophyll content in Zingiber officinale Rosc grown under water stress. Indian J Fundam Appl Life Sci. 1:172–176.
- Brady N, Weil R. 2002. The nature and properties of soils. 13th ed. Upper Saddle River (NJ): Prentice Hall; p. 960.
- Carter MR, Gregorich EG, editors. 2008. Soil sampling and methods of analysis. 2nd ed. Canadian Society of Soil Science. Boca Raton (FL): Taylor & Francis Group; p. 198. Available from: http://www.planta.cn/forum/files_planta/methods_of_analysis_212.pdf
- Cęlik Ö, Atak C. 2012. The effect of salt stress on antioxidative enzymes and proline content of two Turkish tobacco varieties. Turk J Biol. 36:339–356.
- Chance B, Maehly C. 1955. Assay of catalase and peroxidases. Methods Enzymol. 11:764–775. 10.1016/S0076-6879(55)02300-8
- Creus CM, Sueldo RJ, Barassi CA. 1998. Water relations in Azospirillum inoculated wheat seedlings under osmotic stress. Can J Bot. 76:238–244.
- Daniels BA, Skipper HD. 1982. Methods for the recovery and quantitative estimation of propagules from soil. In: Schenck NC, editor. Methods and principles of Mycorrhizal research. St. Paul (MN): The American Phytopathological Society; p. 29–36.
- Evelin H, Kapoor R. 2013. Arbuscular mycorrhizal symbiosis modulates antioxidant response in salt-stressed Trigonella foenum-graecum plants. Mycorrhiza. 24:197–208. 10.1007/s00572-013-0529-4
- Farkhondeh R, Nabizadeh E, Jalilnezhad N. 2012. Effect of salinity stress on proline content, membrane stability and water relations in two sugar beet cultivars. Int J Agri Sci. 2:385–392.
- Foyer CH, Halliwell B. 1976. The presence of glutathione and glutathione reductase in chloroplast: a proposed role in ascorbic acid metabolism. Planta. 133:21–25. 10.1007/BF00386001
- García-Garrido JM, Ocampo JA. 2002. Regulation of the plant defence response in arbuscular mycorrhizal symbiosis. J Exp Bot. 53:1377–1386. 10.1093/jexbot/53.373.1377
- Ghogdi EA, Izadi-Darbandi A, Borzouei A. 2012. Effects of salinity on some physiological traits in wheat (Triticum aestivum L.) cultivars. Indian J Sci Technol. 5:1901–906.
- Giannopolitis CN, Ries SK. 1977. Superoxide dismutase. I. Occurrence in higher plants. Plant Physiol. 59:309–314. 10.1104/pp.59.2.309
- Hajiboland R, Aliasgharzadeh N, Laiegh SF, Poschenrieder C. 2012. Colonization with arbuscular mycorrhizal fungi improves salinity tolerance of tomato (Solanum lycopersicon L.) plants. Plant Soil. 331:313–327. 10.1007/s11104-009-0255-z
- Hassine AB, Lutts S. 2010. Differential responses of saltbush Atriplex halimus L. exposed to salinity and water stress in relation to senescing hormones abscisic acid and ethylene. J Plant Physiol. 167:1448–1456. 10.1016/j.jplph.2010.05.017
- He ZQ, He CX, Zhang ZB. 2007. Changes of antioxidative enzymes and cell membrane osmosis in tomato colonized by arbuscular mycorrhizae under NaCl stress. Colloid Surf B. 59:128–133. 10.1016/j.colsurfb.2007.04.023
- Heath RL, Packer L. 1968. Photoperoxidation in isolated chloroplasts. I. Kinetics and stoichiometry of fatty acid peroxidation. Arch Biochem Biophys. 125:189–198. 10.1016/0003-9861(68)90654-1
- Heidari M. 2012. Effects of salinity stress on growth, chlorophyll content and osmotic components of two basil (Ocimum basilicum L.) genotypes. Afr J Biotechnol. 11:379–384.
- Hejazi MM, Shariatmadari H, Khoshgoftarmanesh AH, Dehghani F. 2012. Copper effects on growth, lipid Peroxidation, and total phenolic content of rosemary leaves under salinity stress. J Agr Sci Tech. 14:205–212.
- Hiscox JD, Israelstam GF. 1979. A method for the extraction of chlorophyll from leaf tissue without maceration. Can J Bot. 57:1332–1334. 10.1139/b79-163
- Hoagland DR, Arnon DI. 1950. The water-culture method for growing plants without soil. California Agric Exp Station Circ. 347:1–32.
- Huang LL, Yang C, Zhao Y, Xu X, Xu Q, Li GZ, Cao J, Herbert SJ, Hao L. 2008. Antioxidant defenses of mycorrhizal fungus infection against SO2-induced oxidative stress in Avena nuda seedlings. Bull Environ Contam Toxicol. 81:440–444. 10.1007/s00128-008-9521-7
- Iqbal M, Ashraf M. 2013. Alleviation of salinity-induced perturbations in ionic and hormonal concentrations in spring wheat through seed preconditioning in synthetic auxins. Acta Physiol Plant. 35:1093–1112.
- Jackson ML. 1962. Soil chemical analysis. New York (NY): Prentice Hall; p. 263–268.
- Julkunen-Tiitto R. 1985. Phenolic constituents in the leaves of northern willows: methods for the analysis of certain phenolics. J Agric Food Chem. 33:213–217. 10.1021/jf00062a013
- Kadian N, Yadav K, Badda N, Aggarwal A. 2013. Application of arbuscular Mycorrhizal fungi in improving growth and nutrient of Cyamopsis tetragonoloba (L.) Taub. under saline soil. Int J Agron Plant Prod. 4:2796–2805.
- Lim JH, Park KJ, Kim BK, Jeong JW, Kim HJ. 2012. Effect of salinity stress on phenolic compounds and carotenoids in buckwheat (Fagopyrum esculentum M.) sprout. Food Chem. 135:1065–1070. 10.1016/j.foodchem.2012.05.068
- Lowry OH, Rosenbough NJ, Farr AL, Randall RJ. 1951. Protein measurement with folin reagent. J Biol Chem. 193:269–275.
- Mehr ZS, Khajeh H, Bahabadi SE, Sabbagh SK. 2012. Changes on proline, phenolic compounds and activity of antioxidant enzymes in Anethum graveolens L. under salt stress. Int J Agron Plant Prod. 3(S): 710–715.
- Miller G, Suzuki N, Ciftci-Yilmaz S, Mittler R. 2010. Reactive oxygen species homeostasis and signalling during drought and salinity stresses. Plant Cell Environ. 33:453–467. 10.1111/j.1365-3040.2009.02041.x
- Mohammad MJ, Hamad SR, Malkawi HI. 2003. Population of arbuscular mycorrhizal fungi in semi-arid environment of Jordan as influenced by biotic and abiotic factors. J Arid Environ. 53:409–417. 10.1006/jare.2002.1046
- Nakano Y, Asada K. 1981. Hydrogen peroxide is scavenged by ascorbate-specific peroxidase in spinach chloroplasts. Plant Cell Physiol. 22:867–880.
- Nounjan N, Nghia PT, Theerakulpisut P. 2012. Exogenous proline and trehalose promote recovery of rice seedlings from salt-stress and differentially modulate antioxidant enzymes and expression of related genes. J Plant Physiol. 169:596–604. 10.1016/j.jplph.2012.01.004
- Petridis A, Therios I, Samouris G, Koundouras S, Giannakoula A. 2012. Effect of water deficit on leaf phenolic composition, gas exchange, oxidative damage and antioxidant activity of four Greek olive (Olea europaea L.) cultivars. Plant Physiol Biochem. 60:1–11.
- Pohjala L, Tammela P. 2012. Aggregating Behavior of Phenolic Compounds – A Source of False Bioassay Results? Molecules. 17:10774–10790. 10.3390/molecules170910774
- Rasool R, Hameed A, Azooz MM, Rehman M, Siddiqi TO, Ahmad P. 2013. Salt stress: causes, types and responses of plants. Ecophysiology and responses of plants under salt stress. New York (NY): Springer
- Rasool S, Ahmad A, Siddiqi TO, Ahmad P. 2013. Changes in growth, lipid peroxidation and some key antioxidant enzymes in chickpea genotypes under salt stress. Acta Physiol Plant. 35:1039–1050.
- Rice-Evans C, Miller N, Paganga G. 1997. Antioxidant properties of phenolic compounds. Trends in Plant Science. 2:152–159. 10.1016/S1360-1385(97)01018-2
- Schenck NC, Perez Y. 1990. Manual for the identification of VA mycorrhizal fungi. 3rd edition. Gainesville (FL): Synergistic.
- Shaheen S, Naseer S, Ashraf M, Akram NA. 2013. Salt stress affects water relations, photosynthesis, and oxidative defense mechanisms in Solanum melongena L. J Plant Interact. 8:85–96. 10.1080/17429145.2012.718376
- Sheng M, Tang M, Chen H, Yang B, Zhang F, Huang Y. 2008. Influence of arbuscular mycorrhizae on photosynthesis and water status of maize plants under salt stress. Mycorrhiza. 18:287–296. 10.1007/s00572-008-0180-7
- Stewart CR. 1981. Proline accumulation: biochemical aspects. In: Paleg LG, Aspinall D, editors. Physiology and biochemistry of drought resistance in plants. p. 243–251.
- Sultana N, Ikeda T, Itoh R. 1999. Effect of NaCl salinity on photosynthesis and dry matter accumulation in developing rice grains. Environ Exp Bot. 42:211–220. 10.1016/S0098-8472(99)00035-0
- Tsao R. 2010. Chemistry and biochemistry of dietary polyphenols. Nutrients. 2:1231–1246. 10.3390/nu2121231
- Utobo EB, Ogbodo EN, Nwogbaga AC. 2011. Techniques for extraction and quantification of arbuscular mycorrhizal fungi. Libyan Agric Res Center Int. 2:68–78.
- Wolf B. 1982. A comprehensive system of leaf analyses and its use for diagnosing crop nutrient status. Comm Soil Sci Plant Anal. 13:1035–1059. 10.1080/00103628209367332
- Wu QS, Zou YN, Liu W, Ye XF, Zai HF, Zhao LJ. 2010. Alleviation of salt stress in citrus seedlings inoculated with mycorrhiza: changes in leaf antioxidant defense systems. Plant Soil Environ. 56:470–475.
- Zheng Y, Wang Z, Sun X, Jia A, Jiang G, Li Z. 2008. Higher salinity tolerance cultivars of winter wheat relieved senescence at reproductive stage. Environ Exp Bot. 62:129–138. 10.1016/j.envexpbot.2007.07.011
- Zörb C, Herbst R, Forreiter C, Schubert S. 2009. Short-term effects of salt exposure on the maize chloroplast protein pattern. Proteomics. 9:4209–4220. 10.1002/pmic.200800791