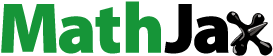
ABSTRACT
Feruloyl esterases (FAEs) are essential accessory enzymes in the hydrolysis of plant cell wall structure. A novel FAE was obtained from Penicillium sumatrense NCH-S2. Enzyme purification was conducted by ultrafiltration, ammonium sulfate precipitation, anion exchange, and gel filtration chromatography. This FAE has a molecular mass of 36 kDa. Its optimum temperature and pH were 50°C and pH 9.0–10.0, respectively. The FAE demonstrated high pH stability at the pH ranging from 6.0 to 10.0. After enzymatic hydrolysis with FAE, the DPPH free radical scavenging capacity, ferrous ion chelating ability, and total phenolic content (TPC) of defatted rice bran (DRB) hydrolysate significantly increased. Furthermore, the amount of released FA from DRB under a synergistic interaction of FAE and hemicellulose increased by 18–21% in comparison with that of either enzyme acting alone.
RESUMEN
Las feruloil esterasas (FAE) son enzimas accesorias esenciales en la hidrólisis de la estructura de la pared celular de las plantas. Para el presente estudio se obtuvo una nueva FAE a partir de Penicillium sumatrense NCH-S2. La purificación de dichas enzimas se llevó a cabo mediante ultrafiltración, precipitación de sulfato de amonio, intercambio de aniones y cromatografía de filtración en gel. La nueva FAE obtenida tiene una masa molecular de 36 kDa. Su temperatura y pH óptimos fueron 50°C y 9.0-10.0, respectivamente. La FAE exhibió alta estabilidad de pH en el rango de 6.0 a 10.0. Tras la hidrólisis enzimática con FAE, la capacidad de eliminación de radicales libres del DPPH, la capacidad de quelación de iones ferrosos y el contenido fenólico total (TPC) del hidrolizado de salvado de arroz desgrasado (DRB) aumentaron significativamente. Además, la cantidad de FA liberado de DRB bajo la interacción sinérgica de FAE y hemicelulasa aumentó entre 18 y 21% en comparación con la de cualquiera de las dos enzimas actuando solas.
1. Introduction
Lignocellulosic biomass is one of the most abundant renewable raw materials on earth. It is able to be converted to many value-added products such as biofuels, enzymes, and fine chemicals (Xue et al., Citation2017). However, expensive cellulase enzymes are required in the industrialization of lignocellulose conversion. It was reported that the utilization of cheaper lignocellulosic substrates for enzyme production could lower production cost and environmental pollutants (Iqtedar et al., Citation2015).
Ferulic acid (FA, 4-hydroxy-3-methoxycinnamic acid) has been widely applied in food, pharmaceuticals, and cosmetic industries. It is the most abundant hydroxycinnamic acid in plants. It is often found to link covalently to functional groups like ester or ether bonds on polysaccharides as well as lignins. Many studies have reported that FA and its derivatives possessed a wide variety of bioactivities, such as anti-oxidation (Itagaki et al., Citation2009), anti-microbial action (Borges et al., Citation2013; Khatkar et al., Citation2015), anti-inflammation (Chmielowski et al., Citation2017; Nile et al., Citation2016), anti-diabetes (Adisakwattana et al., Citation2009), and anti-hyperlipidemia (Bumrungpert et al., Citation2018). The antioxidative activity of FA is primarily attributed to its ability to form stable phenoxyl radicals, thus FA could scavenge free radicals, bind transition metals (e.g., iron and copper), and prevent lipid peroxidation (Zduńska et al., Citation2018). However, the binding of FA to polysaccharides would reduce its bioaccessibility and consequently its bioavailability (Amaya Villalva et al., Citation2018). Enzymatic and chemical (e.g., alkaline) hydrolysis are the two main methods to extract FA and increase its bioaccessibility (Ares-Peón et al., Citation2016; Mandal et al., Citation2015; Nieter et al., Citation2016). The enzymatic method involves the use of microbial carboxylic acid esterases such as feruloyl esterases (also known as ferulic acid esterases; FAEs). As compared to chemical methods, enzymatic hydrolysis is relatively environment friendly because of its milder operation condition.
FAEs (E.C. 3.1.1.73), a subclass of the carboxylic ester hydrolases (E.C. 3.1.1), have been found to hydrolyze the ester linkages between hydroxycinnamic acids and polysaccharides in plant cell walls. Based on the substrate specificities against methyl esters of hydroxycinnamic acids (i.e. methyl ferulate, methyl caffeate, methyl p-coumarate, and methyl sinapate), growth substrate requirements of the microorganisms and protein sequence identity, FAEs could be classified into four types (A-D) (Crepin et al., Citation2004). FAEs have been produced and characterized from a wide range of microorganisms, including bacteria (e.g., Actynomyces, Bacillus, Lactobacillus, Streptomyces) and fungi (e.g., Aspergillus, Fusarium, Penicillium, Talaromyces) (Oliveira et al., Citation2019; Topakas et al., Citation2007). It was reported that FAEs were typically found when these microbes were cultivated on complex substrates. Agricultural by-products, such as wheat bran, corn bran, sugar beet pulp, and rice bran, contain high amounts of esterified FA. These by-products were efficiently used as the substrates for microbial production of FAEs (Goufo & Trindade, Citation2014; Liyana-Pathirana & Shahidi, Citation2006; Saulnier & Thibault, Citation1999).
Previous studies have mentioned that FAE worked synergistically with cellulases or hemicellulases to enhance plant biomass degradation. This was attributed to its ability to break down the lignin-cellulose-hemicellulose crosslinked network, thus increasing the accessibility of cellulases and hemicellulases to their respective substrates (Oliveira et al., Citation2019). Xue et al. (Citation2017) revealed that the synergistic interaction between FAE and cellulase significantly increased the release of phenolic acids from rice straw, and further increasing its antioxidative activities. On the other hand, the release of FA and xylooligosaccharides from wheat bran under combined action of xylanase (AnXyn11A) and FAE (AnFaeA) were greatly enhanced, compared to using AnFaeA alone (Wu et al., Citation2017).
FAEs play an important role in the hydrolysis of lignocellulosic biomass and are applied in many industries. These wide applications involve various substrates, which require multiple FAEs with different specificities. The subtle differences in specificity are often neglected. This may be important in determining the optimum synergy between FAEs and hemicellulases. Therefore, the exploration of novel FAEs with different properties, such as substrate preferences and reaction conditions continues to be an active research area (Topakas et al., Citation2007). However, there are only few reports on FAEs production from Penicillium strains. In this study, we have isolated a new Penicillium sumatrense strain and evaluated its optimum medium for the production of FAE. The FAE produced from this strain was then purified and characterized. Subsequently, FAE and hemicellulases were used in combination with rice bran to evaluate its potentiality in improving the antioxidant activities and released FA of rice bran.
2. Materials and methods
2.1. Screening and cultivation of microorganisms
A screening assay was conducted according to the methods of Donaghy et al. (Citation1998) with some modifications. Strains were cultivated using potato dextrose agar (PDA, Difco, USA). Before pouring plates, each agar plate (15 mL) was supplemented with 0.15 mL ethyl ferulate (10% v/v in dimethylformamide) and was ensured a homogeneous distribution (cloudy overlay) throughout the plate. After incubating at 30°C for 6 d, the formation of clear zone around the colonies indicated feruloyl esterase production. The colonies with positive reaction were subsequently picked and transferred to potato dextrose broth (PDB, Difco, USA) supplemented with 2% (w/v) rice bran to determine the amount of released FA.
2.2. Identification of strain
The strain was identified by Bioresources Collection & Research Center (BCRC, Hsinchu, Taiwan). Morphological characteristics (i.e. colony color, diameter, and conidiophore morphology) of the strain were observed on three differential media: Czapek yeast extract agar (CYA), malt extract agar (MEA), and yeast extract sucrose agar (YES). DNA sequencing of the strain was conducted by rDNA ITS1-5.8S-ITS2 sequence analysis and the sequences were compared with NCBI GenBank database.
2.3. Determination of ferulic acid
The liberated free FA was analyzed by high performance liquid chromatography (HPLC). Sample was filtered through a 0.22 μm membrane filter into HPLC vial. The filtrate was injected into an HPLC system (Hitachi Ltd., Japan) equipped with an autosampler (L-2200; Hitachi Ltd., Japan), a photodiode-array detector (L-2455; Hitachi Ltd., Japan), and a reverse phase column (Mightysil RP-18 GP 250 × 4.6 mm, 5 μm, Kanto Corporation, USA) according to the methods of Yuan et al. (Citation2005). The mobile phase consisted of methanol/water/acetic acid (50:50:0.5, v/v/v), and eluted at a flow rate of 0.8 mL/min for 30 min. The absorbance of the eluate was monitored continuously at 320 nm. The concentration of FA was calculated using the standard curve of FA solutions.
2.4. Enzyme production
One mL of spore suspension with a concentration of 1 × 108 spores/mL was inoculated into a 500 mL Erlenmeyer flask containing 100 mL medium. The medium (containing 1.0 g K2HPO4, 0.5 g KCl, 0.5 g MgSO4·7 H2O, and 0.01 g FeSO4·7 H2O in 1 L of distilled water as basal medium) was supplemented with 120 g rice bran, 40 g sucrose, and 10 g sodium nitrate. The flasks were then placed in an orbital shaker at 30°C, 120 rpm. After 6 days of incubation, the fermentation residues were removed from the culture broth by centrifugating at 12,000 × g for 20 min. The supernatant was collected and concentrated to one fifth of the original volume by ultrafiltration with Minimate Tangential Flow Filtration System (Pall Corporation, USA) equipped with Minimate TFF Capsules (10 kDa and 100 kDa molecular weight cut off). The retentate from the ultrafiltration was then further purified to obtain the enzyme.
2.5. Enzyme purification
The purification flow chart of FAE is described in . After ultrafiltration, the crude enzyme was precipitated with 50–60% (w/v) ammonium sulfate. The precipitate was dialyzed against 20 mM Tris-HCl buffer (pH 7.0). After that, the enzyme sample was subjected to anion exchange chromatography, which was performed on a HiPrep DEAE FF 16/10 column (1.6 × 10 cm, GE Healthcare, USA) with ÄKTApurifier 10 system (GE Healthcare, USA). The mobile phase consisted of Buffer A (20 mM Tris-HCl buffer, pH 7.0) and Buffer B (Buffer A containing 1 M NaCl). The enzyme sample was loaded onto a pre-equilibrated column. After washing with Buffer A, a linear gradient of 0–1 M NaCl was applied with a flow rate of 0.5 mL/min. Eluted fractions (3 mL each) were collected and analyzed for their FAE activity. The active fractions were loaded onto a Hiprep 16/60 Sephacryl S-200 HR column (1.6 × 60 cm, GE Healthcare, USA) equilibrated and eluted with 20 mM Tris-HCl buffer containing 0.15 M NaCl (pH 7.0). The fractions (2 mL each) were collected at a flow rate of 0.8 mL/min and FAE activity was measured for each fraction.
2.6. FAE activity
FAE activity was assayed by measuring the amount of FA released from methyl ferulate (MFA). The assay was carried out in 20 mM Tris-HCl buffer (pH 7.0) containing 4.8 mM methyl ferulate as substrate at 50°C for 10 min and the liberated free FA was analyzed by HPLC as the methods described in 2.3. One unit of FAE activity is defined as the amount of enzyme required to release one μmol FA per min.
2.7. Determination of molecular mass
Enzyme purity and molecular mass were examined by sodium dodecyl sulfate-polyacrylamide gel electrophoresis (SDS-PAGE) according to the methods of Laemmli (Citation1970) with some modifications. SDS-PAGE was carried out using a 10% resolving gel and a 5% stacking gel. At the end of electrophoresis, the gel was stained with Coomassie brilliant blue. The electrophoretic mobility of the FAE was compared with prestained protein ladder (Thermo Scientific, USA). Gel filtration on a Sephacryl S-200 HR column, which was previously calibrated with molecular mass standards, was also employed to determine the molecular mass of the FAE from a calibration curve.
2.8. Determination of protein concentration
Total protein concentration was determined according to the Bradford method (Bradford, Citation1976), using Bio-Rad protein assay dye reagent. Bovine serum albumin was used as the standard. The protein samples were analyzed at 280 nm using a microplate reader (FLUOstar Omega; BMG LABTECH, Germany).
2.9. Characterization of FAE
The optimum temperature was studied by measuring the enzyme activity in 20 mM Tris-HCl buffer (pH 7.0) at different temperatures ranging from 30°C to 80°C. To determine thermostability, the enzyme was pre-incubated at different temperatures between 30°C and 80°C. The residual activity was determined at 50°C at one hour intervals.
The optimum pH was identified at 50°C in various buffers: 20 mM citrate buffer (pH 4.0–7.0), 20 mM Tris-HCl buffer (pH 7.0–10.0), and 20 mM Na2HPO4-NaOH (pH 10.0–11.0). In order to estimate pH stability, the enzyme was pre-incubated in various buffers (pH 4.0-pH 11.0) at 4°C for 24 h, and the residual activity was determined under the conditions (pH 7.0, 50°C).
Methyl caffeate (MCA), methyl p-coumarate (MpCA), and methyl sinapate (MSA) were used to evaluate the substrate specificity of the purified FAE. The enzyme activity was measured as described in 2.6.
2.10. Determination of antioxidant capacity
The fresh rice bran was sieved through a 20-mesh sieve. The sieved rice bran was subsequently defatted with n-hexane at a bran/solvent ratio of 1:10 (w/v) to obtain defatted rice bran (DRB). One mg of DRB was mixed with 10 mL Tris-HCl buffer (20 mM, pH 7). Then, 0.1 mL of purified FAE (1 mU) was added to the mixture and reacted at 50°C for 1 h. The residues were removed by centrifugating at 12,000 × g for 20 min. The antioxidant capacity of supernatant was determined by using the methods described as follows.
2.10.1. Determination of DPPH radical-scavenging capacity
The DPPH assay was conducted according to the methods of Shimada et al. (Citation1992) with some modifications. First, 0.1 mL of sample or ethanol (control) was mixed with 0.4 mL Tris-HCl buffer (100 mM, pH 7.4). Then, 0.5 mL of 250 μM DPPH (1,1-diphenyl-2-picrylhydrazyl) in ethanol was added to the mixture prior being mixed evenly. The mixtures were allowed to stand in the dark at room temperature for 20 min before reading the absorbance at 517 nm. The scavenging capacity of the sample was calculated using the following equation:
2.10.2. Determination of ferrous ion chelating ability
The ferrous ion (Fe2+) chelating ability was performed according to the methods of Decker and Welch (Citation1990) with some modifications. The sample (0.25 mL) was mixed with 0.8 mL distilled water and 0.025 mL FeCl2 (2 mM). After 30 s, 0.05 mL of 5 mM ferrozine was added. The solution was allowed to stand for 10 min at room temperature before taking the absorbance of samples at 562 nm. The chelating ability of the sample was calculated using the following equation:
2.10.3. Determination of total phenolic content
The total phenolic content (TPC) was determined using the Foline-Ciocalteu colorimetric method by Sato et al. (Citation1996) with modifications. The sample (0.4 mL) was mixed with 0.4 mL Foline-Ciocalteu reagent. After reacting for 3 min, 0.04 mL of 10% sodium carbonate was added to the solution and allowed to stand in the dark for 1 h at room temperature. The samples were measured at 735 nm versus a blank using a spectrophotometer. The results were expressed as mg of gallic acid equivalent (GAE)/mL of sample.
2.11. Synergistic interaction between FAE and hemicellulase
One mg of DRB was mixed with 10 mL Tris-HCl buffer (20 mM, pH 7). Then, 0.1 mL of Hemicellulase Amano 90 (Amano Enzyme Inc., Japan) or FAE were added to the mixture and reacted at 50°C. Different enzyme addition sequences were investigated as follows: H5- only hemicellulase was added and reacted for 5 h; F5- only FAE was added and reacted for 5 h; HF5- hemicellulase and FAE were added together and reacted for 5 h; H4F1- add hemicellulase to react for 4 h, then add FAE to react for 1 h; F1H4- add FAE to react for 1 h, then add hemicellulase to react for 4 h; Control- enzymes were replaced with water and reacted for 5 h. The amount of released FA was determined by HPLC as the methods described in 2.2. The amount of released saccharides was analyzed by using a refractive index detector (L-2490; Hitachi Ltd., Japan) and a CarboSep CHO-682 column (Transgenomic, USA). The mobile phase was distilled water at a flow rate of 0.4 mL/min and the column was kept at 80°C.
2.12. Statistical analysis
Statistical differences among groups were determined through one-way analysis of variance (ANOVA) followed by Duncan’s multiple range test using SPSS, version 20 (IBM Corporation, USA). Values of p < .05 were considered statistically significant.
3. Results and discussion
3.1. Screening and identification of strains with FAE activity
During the screening process, S-02 showed an increasing trend in the amount of FA released during fermentation and a higher content (56.13 ± 0.62 μg/mL) on the sixth day. Therefore, S-02 was selected as the strain for further study. It was identified according to the morphological characteristics of colony culture and rDNA ITS1-5.8S-ITS2 sequence analysis (Figure S1) that this particular colony was Penicillium sumatrense. This strain was then named as P. sumatrense NCH-S2.
3.2. Optimization of FAE production
Rice bran has been widely used as a substrate to produce value-added products (Meselhy et al., Citation2020; Wang et al., Citation2020). P. sumatrense NCH-S2 was grown in a liquid culture for eight days using rice bran as the substrate. By the use of optimum medium (containing 12% rice bran, 4% sucrose, and 1% sodium nitrate), FAE activity increased with the incubation period. The maximum FAE production was observed on the sixth day of incubation and the production remained steady until the eighth day (Figure S2). The FAE activity and protein content of the crude enzyme were 8.98 ± 0.19 mU/mL and 0.17 ± 0.01 mg/mL, respectively. The FAE activity was about 49.8 times higher than that of the initial cultivation conditions (0.18 ± 0.04 mU/mL).
3.3. Purification of FAE
In this study, FAE was purified with ultrafiltration, ammonium sulfate precipitation, anion exchange, and gel filtration chromatography. The chromatographic profile of the FAE on anion exchange chromatography is shown in ). Fractions 27 to 29 were found to exhibit the majority of the FAE activity at 0.3–0.5 M NaCl concentration by anion exchange chromatography. During gel filtration chromatography, it was found that fractions 31 to 35 demonstrated higher FAE activity ()). Fractions 33 and 34 with peaked activity were pooled and used for further characterization.
Figure 2. The elution profile of (a) anion exchange and (b) gel filtration chromatography of feruloyl esterase from P. sumatrense NCH-S2.
Figura 2. Perfil de elución de (a) intercambio de aniones y (b) cromatografía de filtración en gel de feruloil esterasa de P. sumatrense NCH-S2
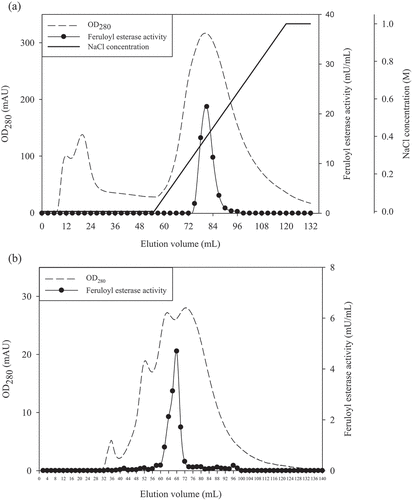
Changes in FAE activity throughout the purification process are shown in . After gel filtration chromatography, FAE activity was purified 13.92-fold. As demonstrated in , only a single band was detected in the purified FAE by SDS-PAGE and the molecular mass was about 37 kDa. Concurrently, the molecular mass of the FAE estimated by gel filtration chromatography was 36 kDa (), which means that the purified FAE is a monomer. Microbial FAEs in previous studies have molecular masses in a typical range of 11–210 kDa (Oliveira et al., Citation2019; Topakas et al., Citation2007).
Table 1. Purification of feruloyl esterase from P. sumatrense NCH-S2.
Tabla 1. Purificación de feruloil esterasa a partir de P. sumatrense NCH-S2
Figure 3. (a) SDS-PAGE and (b) determination of molecular mass of feruloyl esterase (FAE) from P. sumatrense NCH-S2. M: standard protein markers; lane 1: proteins of 50–60% ammonium sulfate saturation; lane 2: proteins of anion exchange chromatography; lane 3: protein of gel filtration chromatography.
Figura 3. (a) SDS-PAGE y (b) determinación de la masa molecular de la feruloil esterasa (FAE) a partir de P. sumatrense NCH-S2. M: marcadores de proteína estándar; carril 1: proteínas de saturación de 50–60% de sulfato de amonio; carril 2: proteínas de cromatografía de intercambio aniónico; carril 3: proteína de cromatografía de filtración en gel
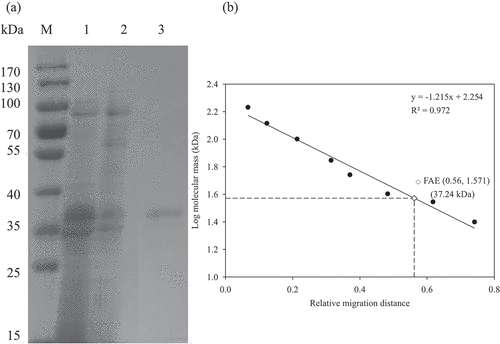
3.4. Characterization of the purified FAE
The optimum temperature of the purified FAE was observed at 50°C ()); however, FAE activity dropped sharply after 60°C. In the evaluation of thermostability, FAE retained 98% and 80% of relative activity after incubating for 5 h at 30°C and 40°C, respectively ()). At 50°C, the FAE activity decreased to 57% in 30 min, and 13% of residual activity was observed after incubation for 5 h. FAE activity was generally abolished in 30 min at temperatures greater than 60°C. The FAEs of Aspergillus niger (AnFaeB and AnFaeC), Aspergillus oryzae (AoFaeA), Aspergillus terreus (AtFaeA and AtFaeD), Penicillium chrysogenum (PcFAE1), and Penicillium brasilianum (FAE) had optimum esterase activity at 50°C (Oliveira et al., Citation2019; Phuengmaung et al., Citation2019). According to relative frequencies and medians, FAEs typically have a molecular mass of 36 kDa, optimum temperature at 50°C, and pH at 6.5 (Oliveira et al., Citation2019). The molecular mass and optimum temperature of the FAE from P. sumatrense NCH-S2 were similar to many past identified FAEs.
Figure 5. (a) Optimum temperature, (b) thermal stability, (c) optimum pH and (d) pH stability of the purified feruloyl esterase from P. sumatrense NCH-S2. Mean values and standard deviations from three replicate experiments are presented.
Figura 5. a) Temperatura óptima, b) estabilidad térmica, c) pH óptimo y d) estabilidad del pH de la feruloil esterasa purificada de P. sumatrense NCH-S2. Se presentan los valores medios y las desviaciones estándar de tres experimentos replicados
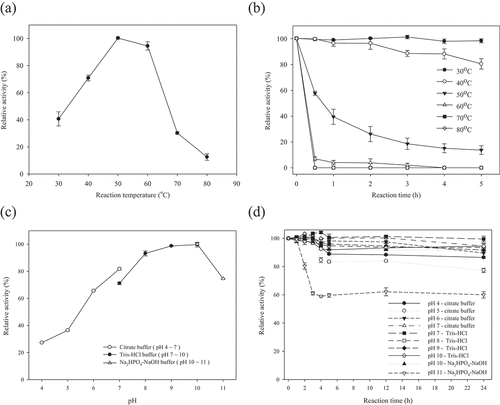
The optimum pH of the FAE produced in this study was at 9.0–10.0 ()). The FAE was more active in alkaline conditions. The relative activity decreased to 27% and 75% at pH 4.0 and pH 11.0, respectively. It was noteworthy to mention that the FAE displayed good pH stability between 6.0 and 10.0 ()). The FAE was also stable at pH 4.0–5.0, which retained over 77% of its initial activity after storage at 4°C for 24 h. Many studies revealed that the majority of fungal FAEs demonstrated either acidic or neutral pH optima (Oliveira et al., Citation2019). Alkaline feruloyl esterases are useful in industrial applications, especially in the treatment of alkaline pulp. Nevertheless, there are scarce reports on such kinds of feruloyl esterases (Li et al., Citation2011). The good activity in alkaline conditions as well as the high stability in both acidic and alkaline conditions made the FAE produced by P. sumatrense NCH-S2 a potential candidate to apply in various industries.
shows the substrate specificity of the purified FAE. The FAE is capable of hydrolyzing four methyl hydroxycinnamates. In particular, the highest FAE activity was observed with MFA (710.71 mU/mg), followed by those for MCA, MSA and MpCA. Phuengmaung et al. (Citation2019) reported that PcFAE1 produced by Penicillium chrysogenum 31B had activity towards MpCA, MFA, and MCA, but not on MSA. PpFAE produced by Penicillium piceum showed enzymatic activity against four methyl hydroxycinnamates, and the highest activity was MpCA (Gao et al., Citation2016). The FAE produced by P. sumatrense NCH-S2 has higher relative activity for MFA comparing to other Penicillium species.
Table 2. Substrate specificity of feruloyl esterase from P. sumatrense NCH-S2.
Tabla 2. Especificidad del sustrato de la feruloil esterasa de P. sumatrense NCH-S2
3.5. Improved antioxidant activities of DRB by the purified FAE
The DPPH radical-scavenging capacity, Fe2+ chelating ability, and total polyphenol content (TPC) were determined to evaluate the antioxidant activities of DRB after treating with purified FAE (). DRB enzymatic hydrolysate had good DPPH scavenging capacity, which increased from 57.44% to 78.18%. The ability to chelate transition metal ions could reflect the antioxidant ability. Fe2+ chelating ability of DRB was increased from 73.56% to 89.78% by enzymatic hydrolysis.
Figure 6. Antioxidant activity and total polyphenol content of defatted rice bran treated with purified feruloyl esterase. Control of each experiment was performed by replacing the purified feruloyl esterase with distilled water. Mean values and standard deviations from three replicate experiments are presented. Bars with different letters (a-b) are significantly different (p < 0.05).
Figura 6. Actividad antioxidante y contenido total de polifenoles del salvado de arroz desgrasado tratado con feruloil esterasa purificada. El control de cada experimento se realizó sustituyendo la feruloil esterasa purificada por agua destilada. Se presentan los valores medios y las desviaciones estándar de tres experimentos replicados. Las barras con letras distintas (a-b) son significativamente diferentes (p < 0.05)
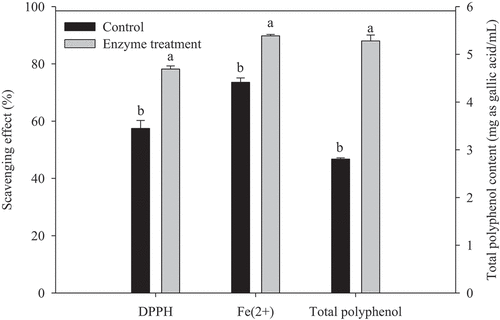
As shown in , TPC of rice bran was increased by 89% (from 2.8 mg as GAE/mL to 5.28 mg as GAE/mL) after the enzymatic treatment. Liu et al. (Citation2017) revealed that enzymatic treatment altered the ratios of free and soluble conjugate forms of phenolics in rice bran extract. The amount of released FA was present at the highest levels, followed by protocatechuic acid. The increases in the phenolic compounds are mainly due to the release of insoluble bound phenolics, such as FA, protocatechuic acid, and quercetin.
The elevations in DPPH radical-scavenging capacity, Fe2+ chelating ability and TPC of the DRB hydrolysate were attributed to the higher amounts of free FA and other phenolic acids from enzymatic hydrolysis (Xue et al., Citation2017). The antioxidant potential of FA can be ascribed to the formation of a phenoxy radical from the phenolic nucleus. The stable resonance structure of the phenoxy radical could cease propagation of radical chain reactions (Paiva et al., Citation2013).
3.6. Synergistic interaction of the purified FAE
demonstrates the variations in the amounts of released FA and different saccharides from DRB by enzymatic hydrolysis with different types of enzymes as well as additional sequences. The control, H5, and F5 treatments were able to release 106.93, 118.46, 115.93 μg/g of FA, respectively, from DRB. Both enzymes significantly (p < .05) enhanced the release of FA. It was interesting to note that the combination of hemicellulase and FAE showed a synergistic effect in which the FA yield increased by 18–21% compared to the F5 treatment. These results indicated that the synergistic interaction of FAE and hemicellulase was useful in extracting FA from DRB, which was consistent with the previous reports (Oliveira et al., Citation2019). More specifically, different addition sequences were found to affect the efficiency to hydrolyze DRB, with H4F1 treatment releasing the highest amount of FA (147.28 μg/g) among the other treatments. Oliveira et al. (Citation2019) mentioned in his review that the sequence and co-incubation treatment with FAE and xylanase on lignocellulosic substrates such as wheat bran, sugar-beet pulp, and sugarcane bagasse also affected the concentration of FA released.
Table 3. Variation in the amount of ferulic acid and saccharides released from defatted rice bran by enzymatic hydrolysis with different enzyme addition sequences.
Tabla 3. Variación de la cantidad de ácido ferúlico y sacáridos liberados del salvado de arroz desgrasado por hidrólisis enzimática con diferentes secuencias de adición de enzimas
According to the report by Beloshapka et al. (Citation2016), DRB contained 34.2% starch, 24.4% dietary fiber and 4.6% hemicellulose. The monosaccharide composition of hemicelluloses from DRB were xylose, arabinose, galactose and glucose (Shibuya & Iwasaki, Citation1985). To evaluate the activity of the FAE produced in this study, different saccharides, including glucose, arabinose, galactose, xylose, and xylobiose were quantified after hydrolyzing DRB. As shown in , apparently higher (p < .05) releases in glucose (3.718–3.872 mg/mL) and arabinose (0.151–0.159 mg/mL) were found with all combinations of hemicellulase and FAE as well as H5 treatments. It was observed that F5 treatment had lower efficiency to release glucose in DRB than that with hemicellulase and its combination treatment. On the other hand, there were no statistical differences in the amounts of galactose, xylose, and xylobiose released from the DRB. Parallel to the results of glucose, all combinations of enzymes (ranging from 3.947 mg/mL to 4.134 mg/mL) as well as H5 treatments (3.971 mg/mL) showed higher amount of total sugars than the other treatments (2.442–2.880 mg/mL).
4. Conclusion
The FAE produced by P. sumatrense NCH-S2 exhibited highest enzyme activity at 50°C. It has unique characteristics such as basophilicity (pH 9.0–10.0) and high stability in both acidic and alkaline conditions. The DPPH radical-scavenging capacity, Fe2+ chelating ability and TPC of DRB were enhanced by FAE hydrolysis. Moreover, the synergistic interaction between hemicellulase and FAE significantly increased the amount of released FA and antioxidant activity of DRB. The results of this study provide useful information for the characteristics of a new FAE produced by P. sumatrense NCH-S2. The enzyme could be a potential candidate to be applied in various industries due to its wide pH stability range and high resistance in alkaline.
Author contributions
Conceptualization, C.S.C., and J.S.L.; Methodology, J.S.L, Y.Q.L. and T.Y.S.; Formal Analysis, J.S.L., and Y.Q.L.; Investigation, C.S.C., J.S.L., and T.Y.S.; Resources, C.S.C.; Data Curation, J.S.L., and Y.Q.L.; Writing – Original Draft Preparation, J.S.L.; Writing – Review & Editing, C.S.C., J.S.L., and Y.Q.L.; Visualization, C.S.C. and J.S.L.; Supervision, C.S.C.; Project Administration, C.S.C. All authors have read and agreed to the published version of the manuscript.
Supplemental Material
Download MS Word (263.6 KB)Acknowledgments
We acknowledge financial support by Grant NSC 101-2221-E-005-079 and NSC 102-2221-E-005-043 from the Ministry of Science and Technology, Taiwan, ROC.
Disclosure statement
No potential conflict of interest was reported by the authors.
Supplementary material
Supplemental data for this article can be accessed on the publisher’s website.
Additional information
Funding
References
- Adisakwattana, S., Chantarasinlapin, P., Thammarat, H., & Yibchok-Anun, S. (2009). A series of cinnamic acid derivatives and their inhibitory activity on intestinal α-glucosidase. Journal of Enzyme Inhibition and Medicinal Chemistry, 24(5), 1194–1200. https://doi.org/https://doi.org/10.1080/14756360902779326
- Amaya Villalva, M. F., González-Aguilar, G., Sández, O. R., Astiazarán García, H., Ledesma Osuna, A. I., López-Ahumada, G. A., & Robles-Sánchez, R. M. (2018). Bioprocessing of wheat (Triticum aestivum cv. Kronstad) bran from Northwest Mexico: Effects on ferulic acid bioaccessibility in breads. CyTA - Journal of Food, 16(1), 570–579. https://doi.org/https://doi.org/10.1080/19476337.2018.1440007
- Ares-Peón, I. A., Garrote, G., Domínguez, H., & Parajó, J. C. (2016). Phenolics production from alkaline hydrolysis of autohydrolysis liquors. CyTA - Journal of Food, 14(2), 255–265. https://doi.org/https://doi.org/10.1080/19476337.2015.1094516
- Beloshapka, A., Buff, P., Fahey, G., & Swanson, K. (2016). Compositional analysis of whole grains, processed grains, grain co-products, and other carbohydrate sources with applicability to pet animal nutrition. Foods, 5(2), 23. https://doi.org/https://doi.org/10.3390/foods5020023
- Borges, A., Ferreira, C., Saavedra, M. J., & Simões, M. (2013). Antibacterial activity and mode of action of ferulic and gallic acids against pathogenic bacteria. Microbial Drug Resistance, 19(4), 256–265. https://doi.org/https://doi.org/10.1089/mdr.2012.0244
- Bradford, M. M. (1976). A rapid and sensitive method for the quantitation of microgram quantities of protein utilizing the principle of protein-dye binding. Analytical Biochemistry, 72(1–2), 248–254. https://doi.org/https://doi.org/10.1016/0003-2697(76)90527-3
- Bumrungpert, A., Lilitchan, S., Tuntipopipat, S., Tirawanchai, N., & Komindr, S. (2018). Ferulic acid supplementation improves lipid profiles, oxidative stress, and inflammatory status in hyperlipidemic subjects: A randomized, double‐blind, placebo‐controlled clinical trial. Nutrients, 10(6), 713. https://doi.org/https://doi.org/10.3390/nu10060713
- Chmielowski, R. A., Abdelhamid, D. S., Faig, J. J., Petersen, L. K., Gardner, C. R., Uhrich, K. E., Joseph, L. B., & Moghe, P. V. (2017). Athero-inflammatory nanotherapeutics: Ferulic acid-based poly(anhydride-ester) nanoparticles attenuate foam cell formation by regulating macrophage lipogenesis and reactive oxygen species generation. Acta Biomaterialia, 57, 85–94. https://doi.org/https://doi.org/10.1016/j.actbio.2017.05.029
- Crepin, V. F., Faulds, C. B., & Connerton, I. F. (2004). Functional classification of the microbial feruloyl esterases. Applied Microbiology and Biotechnology, 63(6), 647–652. https://doi.org/https://doi.org/10.1007/s00253-003-1476-3
- Decker, E. A., & Welch, B. (1990). Role of ferritin as a lipid oxidation catalyst in muscle food. Journal of Agricultural and Food Chemistry, 38(3), 674–677. https://doi.org/https://doi.org/10.1021/jf00093a019
- Donaghy, J., Kelly, P. F., & McKay, A. M. (1998). Detection of ferulic acid esterase production by Bacillus spp. and lactobacilli. Applied Microbiology and Biotechnology, 50(2), 257–260. https://doi.org/https://doi.org/10.1007/s002530051286
- Gao, L., Wang, M., Chen, S., & Zhang, D. (2016). Biochemical characterization of a novel feruloyl esterase from Penicillium piceum and its application in biomass bioconversion. Journal of Molecular Catalysis B: Enzymatic, 133, S388–S394. https://doi.org/https://doi.org/10.1016/j.molcatb.2017.02.012
- Goufo, P., & Trindade, H. (2014). Rice antioxidants: Phenolic acids, flavonoids, anthocyanins, proanthocyanidins, tocopherols, tocotrienols, γ-oryzanol, and phytic acid. Food Science & Nutrition, 2(2), 75–104. https://doi.org/https://doi.org/10.1002/fsn3.86
- Iqtedar, M., Nadeem, M., Naeem, H., Abdullah, R., Naz, S., Syed, Q. U. A., & Kaleem, A. (2015). Bioconversion potential of Trichoderma viride HN1 cellulase for a lignocellulosic biomass Saccharum spontaneum. Natural Product Research, 29(11), 1012–1019. https://doi.org/https://doi.org/10.1080/14786419.2014.971320
- Itagaki, S., Kurokawa, T., Nakata, C., Saito, Y., Oikawa, S., Kobayashi, M., Hirano, T., & Iseki, K. (2009). In vitro and in vivo antioxidant properties of ferulic acid: A comparative study with other natural oxidation inhibitors. Food Chemistry, 114(2), 466–471. https://doi.org/https://doi.org/10.1016/j.foodchem.2008.09.073
- Khatkar, A., Nanda, A., Kumar, P., & Narasimhan, B. (2015). Synthesis and antimicrobial evaluation of ferulic acid derivatives. Research on Chemical Intermediates, 41(1), 299–309. https://doi.org/https://doi.org/10.1007/s11164-013-1192-2
- Laemmli, U. K. (1970). Cleavage of structural proteins during the assembly of the head of bacteriophage T4. Nature, 227(5259), 680–685. https://doi.org/https://doi.org/10.1038/227680a0
- Li, J., Cai, S., Luo, Y., & Dong, X. (2011). Three feruloyl esterases in Cellulosilyticum ruminicola H1 act synergistically to hydrolyze esterified polysaccharides. Applied and Environmental Microbiology, 77(17), 6141–6147. https://doi.org/https://doi.org/10.1128/AEM.00657-11
- Liu, L., Wen, W., Zhang, R., Wei, Z., Deng, Y., Xiao, J., & Zhang, M. (2017). Complex enzyme hydrolysis releases antioxidative phenolics from rice bran. Food Chemistry, 214, 1–8. https://doi.org/https://doi.org/10.1016/j.foodchem.2016.07.038
- Liyana-Pathirana, C. M., & Shahidi, F. (2006). Importance of insoluble bound phenolics to antioxidant properties of wheat. Journal of Agricultural and Food Chemistry, 54(4), 1256–1264. https://doi.org/https://doi.org/10.1021/jf052556h
- Mandal, S., Rath, C., Gupta, C. K., Nath, V., & Singh, H. S. (2015). Probing occurrence of phenylpropanoids in Morinda citrifolia in relation to foliar diseases. Natural Product Research, 29(6), 535–542. https://doi.org/https://doi.org/10.1080/14786419.2014.954245
- Meselhy, K. M., Shams, M. M., Sherif, N. H., & El‐sonbaty, S. M. (2020). Phytochemical study, potential cytotoxic and antioxidant activities of selected food byproducts (Pomegranate peel, Rice bran, Rice straw & Mulberry bark). Natural Product Research, 34(4), 530–533. https://doi.org/https://doi.org/10.1080/14786419.2018.1488708
- Nieter, A., Kelle, S., Linke, D., & Berger, R. G. (2016). Feruloyl esterases from Schizophyllum commune to treat food industry side-streams. Bioresource Technology, 220, 38–46. https://doi.org/https://doi.org/10.1016/j.biortech.2016.08.045
- Nile, S. H., Ko, E. Y., Kim, D. H., & Keum, Y. S. (2016). Screening of ferulic acid related compounds as inhibitors of xanthine oxidase and cyclooxygenase-2 with anti-inflammatory activity. Revista Brasileira De Farmacognosia, 26(1), 50–55. https://doi.org/https://doi.org/10.1016/j.bjp.2015.08.013
- Oliveira, D. M., Mota, T. R., Oliva, B., Segato, F., Marchiosi, R., Ferrarese-Filho, O., Faulds, C. B., & Dos Santos, W. D. (2019). Feruloyl esterases: Biocatalysts to overcome biomass recalcitrance and for the production of bioactive compounds. Bioresource Technology, 278, 408–423. https://doi.org/https://doi.org/10.1016/j.biortech.2019.01.064
- Paiva, L. B., Goldbeck, R., Santos, W. D., & Squina, F. M. (2013). Ferulic acid and derivatives: Molecules with potential application in the pharmaceutical field. Brazilian Journal of Pharmaceutical Sciences, 49(3), 395–411. https://doi.org/https://doi.org/10.1590/S1984-82502013000300002
- Phuengmaung, P., Sunagawa, Y., Makino, Y., Kusumoto, T., Handa, S., Sukhumsirichart, W., & Sakamoto, T. (2019). Identification and characterization of ferulic acid esterase from Penicillium chrysogenum 31B: De-esterification of ferulic acid decorated with L-arabinofuranoses and D-galactopyranoses in sugar beet pectin. Enzyme and Microbial Technology, 131, 109380. https://doi.org/https://doi.org/10.1016/j.enzmictec.2019.109380
- Sato, M., Ramarathnam, N., Suzuki, Y., Ohkubo, T., Takeuchi, M., & Ochi, H. (1996). Varietal differences in the phenolic content and superoxide radical scavenging potential of wines from different sources. Journal of Agricultural and Food Chemistry, 44(1), 37–41. https://doi.org/https://doi.org/10.1021/jf950190a
- Saulnier, L., & Thibault, J. F. (1999). Ferulic acid and diferulic acids as components of sugar beet pectins and maize bran heteroxylans. Journal of the Science of Food and Agriculture, 79(3), 396–402. https://doi.org/https://doi.org/10.1002/(SICI)1097-0010(19990301)79:3<396::AID-JSFA262>3.0.CO;2-B
- Shibuya, N., & Iwasaki, T. (1985). Structural features of rice bran hemicellulose. Phytochemistry, 24(2), 285–289. https://doi.org/https://doi.org/10.1016/S0031-9422(00)83538-4
- Shimada, K., Fujikawa, K., Yahara, K., & Nakamura, T. (1992). Antioxidative properties of xanthan on the autoxidation of soybean oil in cyclodextrin emulsion. Journal of Agricultural and Food Chemistry, 40(6), 945–948. https://doi.org/https://doi.org/10.1021/jf00018a005
- Topakas, E., Vafiadi, C., & Christakopoulos, P. (2007). Microbial production, characterization and applications of feruloyl esterases. Process Biochemistry, 42(4), 497–509. https://doi.org/https://doi.org/10.1016/j.procbio.2007.01.007
- Wang, M., Lei, M., Samina, N., Chen, L. L., Liu, C. L., Yin, T. T., Yan, X. T., Wu, C., He, H., & Yi, C. P. (2020). Impact of Lactobacillus plantarum 423 fermentation on the antioxidant activity and flavor properties of rice bran and wheat bran. Food Chemistry, 330, 127156. https://doi.org/https://doi.org/10.1016/j.foodchem.2020.127156
- Wu, H., Li, H., Xue, Y., Luo, G., Gan, L., Liu, J., Mao, L., & Long, M. (2017). High efficiency co-production of ferulic acid and xylooligosaccharides from wheat bran by recombinant xylanase and feruloyl esterase. Biochemical Engineering Journal, 120, 41–48. https://doi.org/https://doi.org/10.1016/j.bej.2017.01.001
- Xue, Y., Wang, X., Chen, X., Hu, J., Gao, M. T., & Li, J. (2017). Effects of different cellulases on the release of phenolic acids from rice straw during saccharification. Bioresource Technology, 234, 208–216. https://doi.org/https://doi.org/10.1016/j.biortech.2017.02.127
- Yuan, X., Wang, J., Yao, H., & Chen, F. (2005). Free radical-scavenging capacity and inhibitory activity on rat erythrocyte hemolysis of feruloyl oligosaccharides from wheat bran insoluble dietary fiber. LWT-Food Science and Technology, 38(8), 877–883. https://doi.org/https://doi.org/10.1016/j.lwt.2004.09.012
- Zduńska, K., Dana, A., Kolodziejczak, A., & Rotsztejn, H. (2018). Antioxidant properties of ferulic acid and its possible application. Skin Pharmacology and Physiology, 31(6), 332–336. https://doi.org/https://doi.org/10.1159/000491755