Abstract
G-protein coupled receptors (GPCRs) evolved as specialized sensors of the extracellular environment. Comprising the largest family of cell surface receptors, GPCRs are common therapeutic targets. Over the last 25 years, several GPCRs have been observed at the cell nucleus, suggesting the presence of intracrine GPCR signaling beyond the plasma membrane. Yet specific physiological functions of nuclear GPCRs had not been reported, until lately. We recently uncovered distinct but complementary angiogenic roles of F2rl1 (formerly known as PAR2) depending on its subcellular localization at the plasma membrane or at the nucleus. Targeting subcellular compartments to improve drug selectivity may therefore inspire novel therapeutic strategies for transmembrane receptors.
1. Intracrine G-protein coupled receptor signaling
The phrase ‘intracrine signaling’ was initially coined to describe the action of peptide hormones on intracellular receptors Citation[1]. Increasing evidence suggests that G-protein coupled receptors (GPCRs) can also signal inside the cell Citation[2-4]. At the plasma membrane, GPCRs bind heterotimeric G-proteins and β-arrestins to form a signaling platform for second messenger cascades. However, alternative modes of transcriptional regulation have expanded GPCRs’ signaling repertoire. In response to delta-opioid receptor activation, β-arrestin1 translocates to the cell nucleus where it directly regulates histone acetylation and gene transcription, bypassing traditional second messengers Citation[5]. An example of targeting transcriptional events even more directly involves a fragment of Frizzled2 C-terminus, which is able to migrate to the nucleus upon activation Citation[6], with a unique signaling signature in neurons Citation[7]. Full-length GPCRs also internalize following activation and redistribute to early endosomal compartments where cyclic AMP is produced for extended period of time Citation[2,8]. Similarly mitochondrial cannabinoid receptor 1 (Cnr1) triggers cAMP production and protein kinase-A activation in murine hippocampal neurons Citation[3]. GPCRs and their signaling partners have also been detected at the trans-golgi network (e.g., Gpr107) Citation[9] and melanosomes (e.g., Gpr143) Citation[10] in recent years. Hence, the plasma membrane can no longer be considered the exclusive signaling locus of GPCRs. However, the trafficking and signaling pathways of intracellular non-nuclear GPCRs remain largely unknown.
Since 1987 Citation[11], more than 30 GPCRs have been detected at the nuclei of endogenous (non-transfected) cells or tissues Citation[4,12-16]. The presence of phospholipids in the nucleoplasm Citation[17] and nuclear bodies Citation[18], as well as electron microscopy evidence of nuclear membrane invaginations Citation[19] support the existence of intranuclear network of membranes and hydrophobic regions, that could harbor nuclear GPCRs. As GPCRs, tyrosine kinase receptors previously believed to be solely expressed at the plasma membrane have now been uncovered at the nucleus, having distinct signaling signatures Citation[20]. Although the presence of transmembrane receptors at the nucleus is accepted, the mechanisms governing their nuclear localization remain actively investigated.
2. Journey to the center of the cell
Subcellular localization sequence motifs and structural domains dictate the trafficking fate of GPCRs. Following synthesis, GPCRs undergo post-translational modifications in the ER-Golgi network. From the trans-golgi, receptors are delivered to their final destination using the vesicular transport pathway that is tightly regulated by a network of intracellular small GTPases Citation[21]. Interestingly, many GPCRs contain putative nuclear localization signal (NLS) motifs Citation[14] recognized by importins, which are carrier proteins of the karyopherin superfamily. Sorting-nexins (SNX) are also involved in the intracellular sorting of cargo proteins, albeit discovered more recently Citation[22]. In addition, cytoskeletal tracks (microtubules, micro- and intermediate filaments) and carrier motors (dynein and kinesin) drive the vesicular transport of many receptors. Ultimately, the spatio-temporal distribution of the endocytic cargos is determined by the timely interaction of these players, highlighting once more the complexity of intracellular trafficking and ensuing signals. In the case of F2rl1, we found two NLS motifs required for agonist-induced translocation of the receptor mediated by importin α/β heterodimer and Snx11, using retrograde motor dynein via microtubules to reach the nucleus Citation[4]. The oxytocin receptor also translocates to the nucleus upon agonist stimulation and the process is regulated by transportin Citation[12]. Conversely, bradykinin (B2R) and apelin receptors that contain putative NLS motifs are constitutively expressed at the nuclear membrane Citation[14]. To date, mechanisms that dictate constitutive or agonist-induced nuclear localization of GPCRs remain unknown. Choosing the right subcellular therapeutic targeting strategy will require knowledge of the cellular origin of the nuclear GPCR.
3. Location, location, location: why signaling from the nucleus matters to GPCRs?
The presence of GPCRs at the cell nucleus suggests unique subcellular functions. Accordingly, an elaborate GPCR signaling machinery is found at the nucleus, including heterotrimeric G-proteins Citation[23], β-arrestin1 Citation[24], G-protein–receptor kinases Citation[25], regulators of G protein signaling Citation[26] and nuclear membrane channels Citation[27] involved in the regulation of nuclear calcium currents, the latter affecting gene expression Citation[28]. Indeed, direct stimulation of pure isolated nuclei can also result in calcium transients and distinct gene expression profiles Citation[16]. In heart myocardium, nuclear β-adrenergic and endothelin receptors regulate nitric oxide production, thereby, indirectly modulating gene expression Citation[13]. Finally, we recently showed that proximity to the genome allows F2rl1 to form a transcriptional complex that directly binds DNA. Nuclear F2rl1 chromatin immuno-precipitation and sequencing of DNA fragments (ChiP-Seq) confirmed a unique transcriptional program at the nucleus Citation[4]. Hence, nuclear localization of GPCRs may favor in situ interaction with transcription factors and DNA creating a subcellular geographic signaling bias.
To confirm that distinct in vivo phenotypes are conferred by location-specific signaling events, we used F2rl1 deficient mice in which mutant F2rl1 receptors, either preventing nuclear translocation or remaining at the cell nucleus, were introduced in the developing retina. Plasma membrane F2rl1 caused early vascular maturation concordant with increased angiopoeitin-1 (Ang1) and reduced vascular endothelial growth factor-a (Vegfa) synthesis. Nuclear F2rl1 instead promoted a proliferative vascular phenotype, producing more Vegfa and less Ang1. Thus, the same GPCR (F2rl1) in different subcellular locations orchestrates distinct yet complementary angiogenic functions important to retinal vascular development () Citation[4]. Along similar lines, the nuclear oxytocin receptor was recently shown to promote osteoblastic gene expression, required in vivo for bone formation Citation[12]. Dissecting the relative physiological significance of distinct subcellular signals remains a practical challenge but it is the ultimate readout of our efforts to achieve greater therapeutic signaling selectivity.
Figure 1. Subcellular localization of F2rll governs angiogenesis. Schematic representation of F2rll translocation from the plasma membrane to the cell nucleus, requiring Snxll and importin-β on microtubules; ensuing Vegfa expression involves recruitment of transcription factor (Sp1), whereas signaling from F2rll at the plasma membrane results in Angl expression. Putative orientation of nuclear G-protein coupled receptor (GPCR) with N-termini buried within the nuclear GPCR with n-terminal buried within the nuclear envelope, exposing the C-terminus to the cytoplasm (outer NM) or nucleoplasm (inner NM).
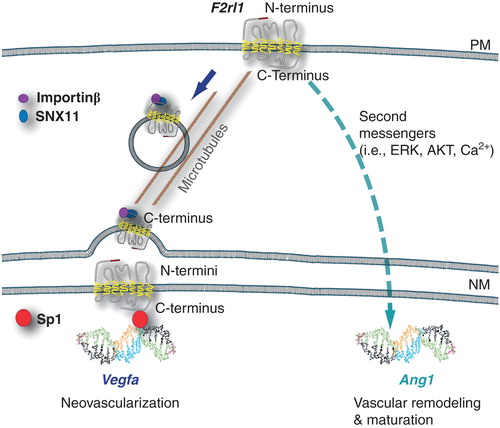
4. Expert opinion: targeting GPCR subcellular localization to achieve greater therapeutic specificity
Understanding GPCRs signaling bias at the plasma membrane may help to improve drug selectivity. To identify bias signaling, conformational changes in receptor’s quaternary structure induced by orthosteric or allosteric modulators are assessed against their functional impact. This iterative exploratory approach will likely be necessary to uncover the means that govern the subcellular distribution of nuclear GPCRs, such as F2rl1. Upon activation at the plasma membrane, F2rl1 simultaneously triggers traditional second messenger cascades and dispatches a pool of receptors to the cell nucleus (and late endosomes); the presence of F2rl1 at the nucleus is negligible prior to stimulation. Hence, pharmacologically tilting the balance between the plasma membrane and nuclear translocation signals will require greater understanding of F2rl1 quaternary structure and the conformational changes that expose the NLS motifs at the expanse of the β-arrestin binding site, for example. Alternatively, interfering with the assembly of carrier proteins (importins, Snx11, dynein) on the receptor may prevent its nuclear translocation, preserving only the plasma membrane signals. Therefore, targeting agonist-induced nuclear translocation mechanisms will involve strategies to modulate signaling at the plasma membrane and relies on pharmacological selectivity and allosteric modulation.
Specifically targeting GPCRs, which are constitutively distributed inside the cell, raises a unique set of delivery challenges. Ligands would first need to cross the plasma membrane and bypass their cell-surface counterparts, in order to reach intracellular targets. Many endogenous as well as synthetic GPCR ligands are hydrophilic and, thus, impermeable to the plasma membrane. ‘Caged ligands’ with hydrophobic protective groups that permeate the cell membrane have recently been used to study intracrine GPCR signaling in intact cells, instead of isolated organelles Citation[13,29]. The ligands are activated intracellularly by exposing the cells to UV light, which removes caging groups without any change to the parent compound. So far, caged ligands have been reported for endothelin, β-adrenergic and angiotensin-II receptors Citation[13,29]. Hence, these ligands offer a solution for targeting intracellular receptors, but are not specific for nuclear GPCRs. Moreover, the application of this approach in vivo is not readily amenable.
The double-membrane anatomy of the nuclear envelope poses a signaling dilemma. At the cell-surface, GPCRs’ N-termini face the extracellular matrix and their ligands bind to one or more of the extracellular domains, whereas inside the cell heterotrimeric G-proteins interact with the C-termini. However, the exact orientation of a GPCR at the nuclear envelope is currently unknown. It is hypothesized that the C-terminus would face either cytoplasm or nucleoplasm for a GPCR located at the outer or the inner nuclear membrane respectively, to allow the interaction with aforementioned signaling messengers (). Accordingly, the ligand-binding domain(s) of GPCRs would be buried inside the nuclear envelope, between outer and inner nuclear membranes. This is not a concern for lipid agonists that can be synthesized locally from nuclear membrane phospholipids, such as the platelet activating factor (PAF) and its nuclear receptor Citation[30]. Other hydrophilic ligands such as norepinephrine use selective membrane transporters (OCT3) at the nuclear envelope and activate nuclear α1-adrenergic receptors in cardiomyocytes Citation[15]. Presently, two promising intracellular drug-delivery systems include lipid-coated nanoparticles and cell-penetrating peptides (CPPs) Citation[31,32]. Some of them use organelle-specific localizing tags, such as NLS for nuclear localization Citation[31,32] and cleavable mitochondria targeting signal (MTS) for mitochondrial drug delivery Citation[33]. However, to date, no drug-delivery strategies have been designed to specifically target nuclear membrane proteins. In our quest to achieve greater therapeutic selectivity, we may expand the therapeutic repertoire of current drugs by developing new intracellular delivery strategies that will harness the functional significance of subcellular GPCR signaling.
Acknowledgments
J-S Joyal and VK Bhosle contributed comparably to this work.
Declaration of interest
J-S Joyal is supported by grants from the Burroughs Wellcome Fund Career Award for Medical Scientists, Foundation Fighting Blindness, Fonds de la Recherche du Québec – Santé (FRQS) and the Canadian Child Health Clinician Scientist Program (CIHR). VK Bhosle is a recipient of CIHR Systems Biology studentship award at McGill University, Montréal, Canada. S Chemtob is supported by the Canadian Institutes of Health Research (CIHR), March of Dimes Birth Defects Foundation, FRQS and Quebec Vision health Network. The authors have no other relevant affiliations or financial involvement with any organization or entity with a financial interest in or financial conflict with the subject matter or materials discussed in the manuscript apart from those disclosed.
Notes
Bibliography
- Re RN. The cellular biology of angiotensin: paracrine, autocrine and intracrine actions in cardiovascular tissues. J Mol Cell Cardiol 1989;21(Suppl 5):63-9
- Ferrandon S, Feinstein TN, Castro M, et al. Sustained cyclic AMP production by parathyroid hormone receptor endocytosis. Nat Chem Biol 2009;5(10):734-42
- Benard G, Massa F, Puente N, et al. Mitochondrial CB(1) receptors regulate neuronal energy metabolism. Nat Neurosci 2012;15(4):558-64
- Joyal JS, Nim S, Zhu T, et al. Subcellular localization of coagulation factor II receptor-like 1 in neurons governs angiogenesis. Nat Med 2014;20(10):1165-73
- Kang J, Shi Y, Xiang B, et al. A nuclear function of beta-arrestin1 in GPCR signaling: regulation of histone acetylation and gene transcription. Cell 2005;123(5):833-47
- Mathew D, Ataman B, Chen J, et al. Wingless signaling at synapses is through cleavage and nuclear import of receptor DFrizzled2. Science 2005;310(5752):1344-7
- Mosca TJ, Schwarz TL. The nuclear import of Frizzled2-C by Importins-beta11 and alpha2 promotes postsynaptic development. Nat Neurosci 2010;13(8):935-43
- Vilardaga JP, Jean-Alphonse FG, Gardella TJ. Endosomal generation of cAMP in GPCR signaling. Nat Chem Biol 2014;10(9):700-6
- Tafesse FG, Guimaraes CP, Maruyama T, et al. GPR107, a G-protein-coupled receptor essential for intoxication by Pseudomonas aeruginosa exotoxin A, localizes to the Golgi and is cleaved by furin. J Biol Chem 2014;289(35):24005-18
- Young A, Powelson EB, Whitney IE, et al. Involvement of OA1, an intracellular GPCR, and G alpha i3, its binding protein, in melanosomal biogenesis and optic pathway formation. Invest Ophthalmol Vis Sci 2008;49(7):3245-52
- Omary MB, Kagnoff MF. Identification of nuclear receptors for VIP on a human colonic adenocarcinoma cell line. Science 1987;238(4833):1578-81
- Di Benedetto A, Sun L, Zambonin CG, et al. Osteoblast regulation via ligand-activated nuclear trafficking of the oxytocin receptor. Proc Natl Acad Sci USA 2014;111(46):16502-7
- Vaniotis G, Glazkova I, Merlen C, et al. Regulation of cardiac nitric oxide signaling by nuclear beta-adrenergic and endothelin receptors. J Mol Cell Cardiol 2013;62:58-68
- Lee DK, Lanca AJ, Cheng R, et al. Agonist-independent nuclear localization of the Apelin, angiotensin AT1, and bradykinin B2 receptors. J Biol Chem 2004;279(9):7901-8
- Wright CD, Chen Q, Baye NL, et al. Nuclear alpha1-adrenergic receptors signal activated ERK localization to caveolae in adult cardiac myocytes. Circ Res 2008;103(9):992-1000
- Gobeil F, Fortier A, Zhu T, et al. G-protein-coupled receptors signalling at the cell nucleus: an emerging paradigm. Can J Physiol Pharmacol 2006;84(3-4):287-97
- Maraldi NM, Mazzotti G, Capitani S, et al. Morphological evidence of function-related localization of phospholipids in the cell nucleus. Adv Enzyme Regul 1992;32:73-90
- Zimber A, Nguyen QD, Gespach C. Nuclear bodies and compartments: functional roles and cellular signalling in health and disease. Cell Signal 2004;16(10):1085-104
- Fricker M, Hollinshead M, White N, et al. Interphase nuclei of many mammalian cell types contain deep, dynamic, tubular membrane-bound invaginations of the nuclear envelope. J Cell Biol 1997;136(3):531-44
- Song S, Rosen KM, Corfas G. Biological function of nuclear receptor tyrosine kinase action. Cold Spring Harb Perspect Biol 2013;5(7). pii: a009001
- Mizuno-Yamasaki E, Rivera-Molina F, Novick P. GTPase networks in membrane traffic. Annu Rev Biochem 2012;81:637-59
- Cullen PJ, Korswagen HC. Sorting nexins provide diversity for retromer-dependent trafficking events. Nat Cell Biol 2012;14(1):29-37
- Willard FS, Crouch MF. Nuclear and cytoskeletal translocation and localization of heterotrimeric G-proteins. Immunol Cell Biol 2000;78(4):387-94
- Scott MG, Le Rouzic E, Perianin A, et al. Differential nucleocytoplasmic shuttling of beta-arrestins. Characterization of a leucine-rich nuclear export signal in beta-arrestin2. J Biol Chem 2002;277(40):37693-701
- Zhang Y, Matkovich SJ, Duan X, et al. Nuclear effects of G-protein receptor kinase 5 on histone deacetylase 5-regulated gene transcription in heart failure. Circ Heart Fail 2011;4(5):659-68
- Hepler JR. R7BP: a surprising new link between G proteins, RGS proteins, and nuclear signaling in the brain. Sci STKE 2005;2005:294-pe38
- Li B, Jie W, Huang L, et al. Nuclear BK channels regulate gene expression via the control of nuclear calcium signaling. Nat Neurosci 2014;17(8):1055-63
- Bading H. Nuclear calcium signalling in the regulation of brain function. Nat Rev Neurosci 2013;14(9):593-608
- Tadevosyan A, Letourneau M, Folch B, et al. Photo-releasable ligands to study intracrine angiotensin II signalling. J Physiol 2015;593(3):521-39
- Marrache AM, Gobeil FJr, Bernier SG, et al. Proinflammatory gene induction by platelet-activating factor mediated via its cognate nuclear receptor. J Immunol 2002;169(11):6474-81
- Cohen O, Granek R. Nucleus-targeted drug delivery: theoretical optimization of nanoparticles decoration for enhanced intracellular active transport. Nano Lett 2014;14(5):2515-21
- Wang F, Wang Y, Zhang X, et al. Recent progress of cell-penetrating peptides as new carriers for intracellular cargo delivery. J Control Release 2014;174:126-36
- Rin Jean S, Tulumello DV, Wisnovsky SP, et al. Molecular vehicles for mitochondrial chemical biology and drug delivery. ACS Chem Biol 2014;9(2):323-33