Abstract
Information regarding the use of functional foods and nutraceuticals (FFN) in combating disease is rarely communicated to health care practitioners as medicinal strategies for patients. Metabolic syndrome (MetS) is an ideal paradigm for demonstrating the therapeutic properties of FFN. Encompassing multiple etiologies, including atherogenic dyslipidemia, insulin resistance, and hypertension, MetS affects over a third of American adults. However, as disease-related risk factors accumulate over time, guidelines for treating disorders of MetS progressively de-emphasize the use of FFN. Using marine omega-3 fatty acids, plant sterols, fiber, and tomato extract as examples, the purpose of this review is to endorse FFN as long-term adjunctive therapies to pharmaceutical treatment for disorders and risk factors for MetS. An additional goal is to compare physiological and molecular targets of FFN against corresponding prescription medications. Results reveal that FFN are viable treatment strategies for disorders of MetS, complementing pharmacological interventions by targeting and improving the biological processes that foster the development of disease. Thus, efficacious FFN therapies should be emphasized throughout all stages of treatment as adjuncts to pharmacotherapy for disorders of MetS. Accordingly, new developments in FFN research must be implemented into clinical guidelines with the prospect of improving disease prognoses as accessories to prescription medications.
Abbreviations | ||
ABCG | = | ATP-binding cassette G transporters |
AMP | = | Adenosine monophosphate |
AMPK | = | AMP-activated protein kinase |
ATP | = | Adenosine triphosphate |
BP | = | blood pressure |
CVD | = | cardiovascular disease |
DBP | = | diastolic blood pressure |
DHA | = | docohexaenoic acid |
EPA | = | eicosapentaenoic acid |
FDA | = | U.S. Food and Drug Administration |
FFN | = | functional foods and nutraceuticals |
FXR | = | farnesol X receptor |
GLUT-1 | = | glucose transporter 1 |
GPR | = | G-coupled proteins |
HCH | = | hypercholesterolemia |
HDL | = | high-density lipoprotein |
HMG-CoA | = | 3-hydroxy-3-methylgutaryl- coenzyme A |
HNF-4α | = | hepatocyte nuclear factor 4α |
HTG | = | hypertriglyceridemia |
IR | = | insulin resistance |
JNCBP | = | The Joint National Committee on Prevention, Detection, Evaluation, and Treatment of High Blood Pressure |
LDL | = | low-density lipoprotein |
LDL-C | = | low-density lipoprotein cholesterol |
LXR | = | liver X receptor |
MetS | = | metabolic syndrome |
MOM-3 | = | marine-derived omega-3 fatty acids |
NADPH | = | nicotinamide adenine dinucleotide phosphate |
NCEP | = | The National Cholesterol Education Program |
NEFA | = | non-esterified fatty acids |
NFκB | = | nuclear factor kappa-light-chain-enhancer of activated B cells |
NO | = | nitric oxide |
PPAR | = | peroxisome proliferator-activated receptor |
RS | = | resistant starch |
SBP | = | systolic blood pressure |
SCFA | = | short-chain fatty acids |
sdLDL | = | small dense low-density lipoprotein |
SREBP 1c | = | sterol regulatory element-binding protein 1c |
TG | = | triglyceride |
VLDL | = | very-low-density lipoprotein |
VLDL-C | = | very-low-density lipoprotein cholesterol |
Key messages
Similar to pharmaceutical agents, functional foods and nutraceuticals (FFN) possess physiological and molecular targets that modulate clinical end-points associated with chronic disease. Despite current research demonstrating that FFN combined with pharmaceuticals can benefit patients better than pharmaco-monotherapy, FFN-based therapies are de-emphasized as risk factors for chronic disease accumulate over time.
In the context of metabolic syndrome, a multifaceted disease state, FFN including marine-derived omega-3 fatty acids, plant sterols, fiber, and tomato extract have been shown to target metabolic processes associated with atherogenic dyslipidemia, hypercholesterolemia, insulin resistance, vascular dysfunction, and hypertension.
FFN should be emphasized throughout all stages of treatment as adjuncts to pharmacotherapy. For this to happen, however, new developments in FFN research must be communicated to health care practitioners and implemented into clinical guidelines so that they may be utilized by modern medicine as tools for combating disease.
Introduction
Nutraceuticals are bioactive compounds that confer protection from chronic disease via mechanisms beyond simply providing nutrition. A food becomes ‘functional’ when levels of one or more nutraceuticals are present at concentrations such that regular consumption elicits a positive biological effect. Nonetheless, nutraceuticals can also be isolated from functional foods and added to other food matrices or concentrated for distribution in capsules or tablets. Similar to pharmaceutical agents, research clearly demonstrates that functional foods and nutraceuticals (FFN) possess physiological and molecular targets that modulate clinical end-points associated with chronic disease. Despite current research demonstrating that FFN combined with pharmaceuticals can benefit patients better than pharmaco-monotherapy (Citation1–3), information regarding the efficacy of FFN-based therapies is rarely communicated to health care practitioners and implemented into disease treatment regimens. Metabolic syndrome (MetS) is an ideal and timely example for asserting FFN efficacy in treating chronic disease given that MetS is a multifaceted ailment affecting a third of American adults (Citation4).
MetS is characterized as a combination of medical disorders including central obesity, insulin resistance (IR), hypertension, as well as atherogenic dyslipidemia, itself characterized as hypertriglyceridemia (HTG), low HDL-C and high levels of circulating small dense LDL-C (sdLDL) (Citation5,Citation6) (). Hypercholesterolemia (HCH), more specifically high levels of circulating low-density lipoprotein cholesterol (LDL-C), has been described as a therapeutic target for atherogenic dyslipidemia (Citation6,Citation7). Moreover, patients with MetS demonstrate an progressive trend towards lower antioxidant activity with onset of MetS co-morbidities (Citation8). Finally, antioxidant status has been associated with the pathogenesis of IR and hypertension (Citation9). Clinical guidelines such as The National Cholesterol Education Program (NCEP) and The Joint National Committee on Prevention, Detection, Evaluation, and Treatment of High Blood Pressure (JNCBP) recommend a spectrum of life-style and pharmaceutical therapies for treating specific disorders of MetS (Citation7,Citation10). While life-style interventions are emphasized during the initial stages of treatment for MetS-related disorders, dietary interventions, specifically FFN, become overshadowed by pharmacological agents as risk factors for chronic disease accumulate over time. For example, current NCEP guidelines recommend plant sterols and stanols (PS) as therapeutic options to clinicians for reducing circulating levels of LDL-C. However, PS are not emphasized as a constituent of the Therapeutic Life-style Change Diet or during the later stages of HCH treatment (Citation7). Similarly, the JNCBP's algorithm for the treatment of hypertension accentuates a combination of pharmacological agents when blood pressure levels (BP) are <140/90 mmHg with no mention of FFN (Citation10). In addition, the JNCBP advocates the use of additional drugs until an ideal BP is achieved. That being said, specific FFN have been identified as efficacious and safe adjunctive therapies for disorders of MetS. Noteworthy are supplemental marine-derived omega-3 fatty acids (MOM-3) and PS. The former has been shown to be a potent treatment for HTG as well as possess secondary cardio-protective benefits including improvements in antioxidant status, vascular function, and inflammatory biomarkers. The latter is an efficacious treatment for HCH, a fact that is supported by over 50 years of research. Other FFN showing promise include supplemental fiber for treating IR and tomato extracts for hypertension.
Figure 1. MetS as a multifaceted disease encompassing central obesity, atherogenic dyslipidemia, hypertension, and insulin resistance. Low antioxidant status can contribute to the development of hypertension and insulin resistance, while hypercholesterolemia, specifically high LDL-C, is a primary target for atherogenic dyslipidemia.
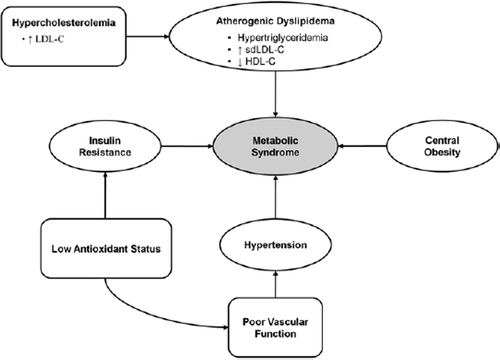
Accordingly, the first objective of this review is to describe the efficacy of MOM-3, PS, fiber, and tomato extract in reducing HTG, HCH, IR, oxidative stress, hypertension, and improving vascular function (). The second objective is to provide a comparison of physiological, metabolic, and molecular targets between the described FFN and corresponding prescription medications currently used for treating disorders of MetS. Finally, this review will discuss studies that have examined the use of pharmacological agents in conjunction with FFN therapies for treating HTG, HCH, IR, and hypertension.
Marine-derived omega-3 fatty acids, triglycerides, and vascular health
Marine-derived omega-3 fatty acids for the treatment of hypertriglyceridemia
Supplemental MOM-3, eicosapentaenoic acid (C20: 5n-3) (EPA), and docohexaenoic acid (22:6n-3) (DHA) have been shown to be efficacious in treating HTG (Citation11). Effects of MOM-3 on circulating triglyceride (TG) concentrations are dose-dependent, with TG-lowering efficacy being demonstrated with dosages ranging from 1.3 to 12.0 g/d (Citation12–15). In a comprehensive review of 65 human clinical studies, Harris et al. (Citation16) demonstrated that MOM-3 consistently reduce TG levels by 25% and 34% in normal and HTG patients, respectively, concluding that additional trials are no longer needed to demonstrate MOM-3's TG-lowering efficacy. Concerns regarding the safety of supplemental MOM-3 stem from Greenland Inuit peoples having significantly longer bleeding times (Citation17). However, in a dose-dependent study whereby MOM-3 was administered at 4.5, 7.5, and 12 g/d, blood coagulation times only increased when volunteers received the highest dose of MOM-3 (Citation14). Hence, 3–4 g/d of supplemental MOM-3 can be regarded as a safe and efficacious treatment for HTG.
Medications versus marine-derived omega-3s for the treatment of hypertriglyceridemia: mechanisms of action
Primary medications used to treat HTG include statins and fibrates. As 3-hydroxy-3-methylgutaryl-coenzyme A (HMG-CoA) reductase inhibitors, statins inhibit hepatic cholesterol synthesis. Unlike statins, fibrates are ligands for nuclear receptors, activating hepatic peroxisome proliferator-activated receptor-α (PPAR-α) and inhibiting hepatocyte nuclear factor 4α (HNF-4α), resulting in higher lipoprotein lipase levels, high-density lipoprotein cholesterol (HDL-C) levels, fatty acid β-oxidation, as well as reduced very-low-density lipoprotein (VLDL) secretion and lower expression of genes involved in carbohydrate and lipid metabolism (Citation18–20) ().
Table I. Summary comparison of triglyceride-lowering mechanisms of action between marine-derived omega-3 fatty acids and prescription medications.
MOM-3 reduce TG levels by modulating the expression of nuclear receptors. Alongside an increase in PPAR-α and inhibition of HNF-4α gene expression, MOM-3 up-regulate and down-regulate farnesol X receptor (FXR) and liver X receptor (LXR) expression, respectively, resulting in an inhibition of sterol regulatory element-binding protein 1c (SREBP 1c) activity and expression (Citation21). SREBP 1c up-regulates the expression of genes involved i n TG synthesis (Citation21–23). Together, the effects of MOM-3 on FXR and PPAR-α also enhance hepatic TG uptake and TG oxidation, respectively (Citation21). Altogether, MOM-3 act on similar molecular targets to pharmaceutical therapy to modulate intracellular TG metabolism and decrease circulating TG levels ().
Medications versus marine-derived omega-3 fatty acids on oxidant status, vascular function, and inflammation
Statins have been shown to reduce C-reactive protein by 67% (Citation24), DNA damage by 20% to 40% (Citation25,Citation26), superoxide anion by 36% (Citation27), auto- antibodies against LDL oxidation by 19% (Citation28), and conjugated diene production by 41% (Citation29). Increases in glutathione peroxidase activity by 38% (Citation29), paraoxonase levels by 32% (Citation30), α-tocopherol levels by 29% and retinol levels by 40% (Citation31) have also been observed with statin therapy. Statins enhance vascular function by inhibiting thromboxane A2 production and inducing vasodilation via increasing nitric oxide synthase levels (Citation32). Additional cardio-protective effects of statins include increased circulating HDL-C levels 3% to 10% (Citation33,Citation34) as well as reductions in sdLDL 24% to 57% (Citation35–38). Compared to large LDL subfractions, sdLDL are better able to infiltrate arteriole walls, possess higher susceptibility to oxidation, and thus initiate development of atherosclerotic plaques. Given that oxidative stress promotes inflammation and has been linked to atherosclerosis (Citation39) and cancer (Citation40,Citation41), statins have been given considerable attention for promoting improvements in oxidative biomarkers in conjunction with their lipid-lowering properties.
Similar to statins, MOM-3 supplementation has been shown to improve antioxidant status via increasing glutathione peroxidase by 30% (Citation42). Supplemental MOM-3 have also been shown to reduce lipid peroxidation by 19% (Citation42), ultraviolet light-induced DNA damage by 70%, and p53 gene expression by 50% (Citation43). MOM-3 consumption also fosters the production of anti-inflammatory signaling molecules including trienoic prostaglandins and 5-series leukotrienes while reducing levels of urinary F2-isoprostanes (Citation44). Products of omega-6 fatty acid oxidation, F2-isoprostanes are biomarkers for the production of pro-inflammatory dienoic prostaglandins (Citation45). MOM-3 promote vasodilation by inhibiting thromboxane A2 production and promoting thromboxane A3 and B3 synthesis (Citation45,Citation46). In addition, Nomura et al. (Citation47) showed that supplementation with 1.8 g/d EPA significantly reduced markers of coagulation including CD62P, CD63, annexin V, as well as platelet and monocyte-derived microparticles, which are prothrombotic. In the same study, EPA decreased E-selectin levels, a biomarker of vascular damage (Citation47). Finally, MOM-3 have been shown to reduce levels of sdLDL by 21% to 37% (Citation48,Citation49) and remnant-like lipoprotein particles by 21% (Citation50), which, as outlined above, are both atherosclerotic. Therefore, MOM-3 can be considered a valuable means for improving vascular function.
Combining marine-derived omega-3 fatty acids and medications as adjunctive treatments for hypertriglyceridemia and other risk factors for cardiovascular disease
Recent studies have emerged advocating adjunctive statin/MOM-3 therapy for treating HTG. The extent to which MOM-3 amplify the TG-lowering effects of statin monotherapy is demonstrated in , showing that combination MOM-3/statin treatments reduce TG an additional 10% to 30% compared with statin therapy alone (Citation1,Citation51–57). Combination therapy has also been shown to further reduce levels of total circulating cholesterol (−4% to −30%) (Citation1,Citation52,Citation54,Citation55), VLDL-C (−20% to −40%) (Citation1,Citation52,Citation53), VLDL-TG (−53%) (Citation56), ApoB100 (−32%), and ApoB48 (−36%) (Citation56), as well as increase HDL-C levels (4% to 18%) (Citation1,Citation52,Citation56) compared to statins alone. These findings indicate that MOM-3 therapy may help improve patients’ overall lipid profiles. In addition, combining MOM-3 with pitavastatin was shown to synergistically improve vascular function by reducing prothrombotic platelet-derived microparticles as well as increasing levels of adiponectin (Citation58). Adiponectin increases NO levels, promoting vasodilation (Citation59,Citation60), as well as decreases monocytes from adhesion to endothelial cells (Citation61). Furthermore, 3.6 g/d MOM-3 further reduced TG and homocysteine levels 28% and 29%, respectively, in patients already receiving statin and fibrate therapy (Citation57). Recently Lovaza® became the first prescription MOM-3 supplement approved by the U.S. Food and Drug Administration (FDA), substantiating the notion that FFN are potent therapies for treating disorders of MetS. The above studies highlight MOM-3 as a potent complementary therapy to pharmacological medications for HTG and other cardiovascular risk factors.
Table II. Summary of studies MOM-3 as a beneficial adjunctive therapy to statin therapy when treating hypertriglyceridemia.
Plant sterols/stanols and hypercholesterolemia
Plant sterols and stanols as treatments for hypercholesterolemia
In 1954, Best et al. (Citation62) first demonstrated that supplemental PS reduce total cholesterol levels in humans. Since then, over 100 clinical trials have shown that PS consumption significantly decreases LDL-C by 5% to 15% (Citation63). A recent meta-analysis confirmed that PS-derived reductions in LDL-C are dose-dependent with maximum efficacy peaking at 2.0–2.5 g/d (Citation64). Typically, food-based vehicles are used to administer PS. However, PS capsules are available and have also been shown to be efficacious for reducing LDL-C (Citation65,Citation66).
Medications versus plant sterols for the treatment of hypercholesterolemia: mechanisms of action
Statins, bile acid resins, niacin, and cholesterol absorption inhibitors (ezetimibe) are among the most popular treatments for HCH (). As outlined above, statins inhibit hepatic cholesterol synthesis, while bile acid resins bind bile and inhibit cholesterol reabsorption in the large intestine (Citation67). Niacin reduces hepatic lipid synthesis and adipocyte lipolysis (Citation68). Ezetimibe reduces biliary cholesterol absorption by interacting with enterocyte Niemann-Pick C1-like protein 1 transporters, resulting in an increase in hepatic LDL uptake (Citation67). Similar to ezetimibe PS are believed to displace dietary cholesterol from being incorporated into mixed micelles in the small intestine (Citation69) (). Absorption of PS into the circulation is poor at 0.1%–1.9% (Citation70) thus the majority of PS action occurs in the lumen through competition. Evidence suggests that PS act as LXR agonists and induce the expression of enterocyte ATP-binding cassette G transporters (Citation71) which subsequently pump PS from the enterocytes back into the gastrointestinal lumen (Citation72).
Table III. Summary comparison of LDL-lowering mechanisms of action between plant sterols and stanols and prescription medications.
Medications and plant sterols: mechanisms for reducing vascular plaque formation
The LDL-C-lowering effects of PS and statins may also confer protection against the formation of atherosclerotic plaques via nuclear factor kappa-light-chain-enhancer of activated B cells (NFκB) and monocyte chemoattractant protein-1 (MCP-1) pathways. LDL-cholesterol levels have been shown to increase MCP-1 expression directly as well as indirectly via NFκB. Endothelial expression of MCP-1 has been implicated for increasing vascular lipid accumulation, macrophage recruitment, and atherosclerotic plaque growth (Citation73). Studies demonstrate that statin treatment decreases atherosclerotic plaque formation alongside reduced NFκB and MCP-1 expression (Citation74–76). Although not demonstrated in vivo, in vitro experiments show that PS inhibited MCP-1 production in umbilical venous endothelial cells treated with oxidized LDL (Citation77). The authors hypothesize that PS could modulate NFκB activity or expression and would suggest that, similar to statins, PS reduce MCP-1 through LDL-C-lowering and NFκB-related pathways. Further studies are needed to determine if combination PS/statin therapy can produce an additive effect on MCP-1 expression.
Combining plant sterols/stanols and prescription medications as adjunctive treatments for hypercholesterolemia
The magnitude of PS/statin therapy on LDL-C is demonstrated in . Studies reveal that when PS are combined with statins, LDL-C levels are reduced an additional 5% to 17%, compared to statins alone (Citation3,Citation65,Citation78–82). PS efficacy is not affected by the type or dose of statin administered, with LDL-C reductions being observed in patients taking simvastatin, lovastatin, atorvastatin, pravastatin, fluvastatin, rosuvastatin, and cerivastatin (Citation3,Citation65,Citation78–82). Simons et al. (Citation81) demonstrated that combination PS/statin therapy is additive, reducing LDL-C levels by 39%, which is equivalent to the effect of doubling the dose of statins. Furthermore, the proportion of patients with LDL-C levels <4 mmol/L increased from 33% in subjects receiving statins alone to 67% and 72% for patients receiving combination statin/plant sterol or statin/plant stanol therapy, respectively (Citation80). Plant sterols should be touted as a safe and efficacious adjunctive therapy to statin treatment for HCH, a major contributor to the development of MetS.
Table IV. Summary of studies showing plant sterols and stanols as a beneficial adjunctive therapy to statin therapy for the treatment of hypercholesterolemia.
The area of concomitant PS therapy with other HCH medications has been understudied. Ezetimibe/PS therapy has been found to reduce LDL-C levels 25%, but was insignificant compared to ezetimibe therapy alone (222%) (Citation83). Given that PS and ezetimibe both inhibit cholesterol absorption it is possible that the effects of PS on LDL-C were overshadowed by the action of ezetimibe. Conversely, PS/fibrate therapy has been shown to reduce LDL-C levels 11.1% compared to 7.7% after PS therapy alone (Citation84). Studies combining PS with bile acid sequestrants or niacin have yet to be published. Undoubtedly, further research exploring the benefits of using PS together with lipid-lowering medications other than statins is warranted. Until then, patients prescribed statin medications can be advised, with confidence, to ingest PS to produce greater reductions in LDL-C compared to statins alone.
Fiber and insulin resistance
Soluble and insoluble fiber and resistant starch as treatments for insulin resistance
Recently soluble fiber, insoluble fiber, and resistant starches have been explored for their effects on IR. Insulin resistance, a MetS co-morbidity, has been long associated with the development of other metabolic disorders and disease risk factors, including polycystic ovary syndrome, type II diabetes, cardiovascular disease (CVD), hypertension, obesity, and inflammation (Citation85,Citation86). As such, in 1992, Rupp et al. (Citation87) emphasized the need for dietary interventions that target and subsequently reduce IR. Recent insights into the biological effects of non-digestible carbohydrates suggest fibers can significantly improve IR.
Epidemiological studies have found associations between fiber intake and IR. A case-control study showed that higher consumption of soluble fiber amongst vegetarians from whole grains, pulses, oats, and barley preserved insulin sensitivity independent of age (Citation88). On the other hand, non-vegetarians began to show evidence of IR between 31 and 40 years of age (Citation88). Cross-sectional data from the Framingham Offspring Cohort noted an inverse association between cereal fiber intake and IR (Citation89), while a cross-sectional analysis of men and women from the Inter99 study showed a relationship between low-fiber consumption and the probability of developing IR (Citation90).
An association between supplemental fiber intake and IR has also been shown in randomized clinical trials. Studies utilizing euglycemic-hyperinsulinemic clamps demonstrate a 14% and 8% decrease in IR with resistant starch and cereal fiber, respectively (Citation91,Citation92). Moreover, homeostasis-modeling assessment, a non-invasive method for measuring IR using fasted blood samples, showed a 33% and 24% reduction in IR after 3 weeks of consuming 3 g/d β-glucan and 4 wks of a fiber-rich (20 g/d) meal replacement beverage, respectively (Citation93,Citation94). Similarly, despite no effect on postprandial glycemia, high-fiber bread increased postprandial insulin economy (Citation95). Furthermore, using the minimal model index as a measure of IR, 60 g/d resistant starch ingested 24 hrs prior to a fiber-free meal was shown to decrease postprandial IR 69%, compared to controls (Citation96). Resistant starch has also been shown to increase muscle and adipose glucose clearance by 44% and 87%, respectively (Citation91). In another study, lack of effect of 1.5 g/d β-glucan on IR could have been secondary to the low dose administered to subjects (Citation97). An acute dose-dependent study demonstrated that only the maximum dose of 10 g/d β-glucan was able to blunt postprandial insulin responses, despite no effect on postprandial glucose response (Citation98). Overall, data suggest that the dose and duration of intake of fiber impact when and if changes in IR will be observed.
Fibers versus prescription medications for treating insulin resistance: mechanisms of action
In addition to treating hyperglycemia, metformin and thiazolidinediones are prescription medications that have also been shown to decrease IR. The exact mechanisms by which metformin reduces IR remain to be completely understood. Studies suggest that metformin stimulates adenosine monophosphate (AMP)- activated protein kinase (AMPK) (Citation99–101), tyrosine kinase phosphorylation at the β-subunit on the insulin receptor (Citation102–104), as well as muscle atypical protein kinase C activation (Citation105). Similar to metformin, thiazolidinediones activate AMPK (Citation106) (). Moreover, thiazolidinediones target adipose tissue and act as a PPAR-γ ligand (Citation107), reducing free fatty acid release (Citation108) as well as the expression of IR-related substances such as leptin (Citation109), tumor necrosis factor (TNF)-α (Citation110,Citation111), and resistin (Citation112). It has also been suggested that thiazolidinediones decrease inhibitory insulin receptor substrate protein 1 serine phosphorylation (Citation113) and increase glucose disposal via higher expression of glucose transporter (GLUT)-1 in adipose (Citation114) and skeletal muscle (Citation115).
Table V. Summary comparison of insulin resistance-lowering mechanisms of action between fiber and prescription medications.
Short-chain fatty acids (SCFA), primarily acetate, butyrate, and propionate, are metabolites of microbial fermentation of dietary fiber in the large intestine and are thought to be important signaling molecules responsible for fiber-mediated decreases in IR (Citation116). Since high levels of circulating non-esterified free fatty acids (NEFA) have been linked to IR (Citation117), it has been suggested that SCFA reduce circulating NEFA (). Conclusive evidence of this phenomenon has yet to be presented in vivo. However, compared to controls, Robertson et al. (Citation91) observed a significant increase in SCFA production in subjects consuming 30 g/d RS alongside an increase in propionate and acetate uptake by adipose and muscle tissue, respectively. At the same time, subjects noted a decrease in adipose-derived NEFA and an increase in skeletal muscle and adipose glucose clearance. Interestingly, Robertson et al. also observed an increase in circulating ghrelin after resistant starch (RS) supplementation (Citation91) and noted that higher ghrelin levels associate with reduced IR (Citation118–120). Rat adipocytes showed an increase in PPAR-γ expression and lipogenesis after cells were treated with ghrelin (Citation121). Moreover, recent studies point towards SCFA acting as ligands for adipose G-coupled proteins (GPR). Robertson (Citation116) has also suggested that interactions between SCFA and adipose GPR43 could be of importance in decreasing NEFA levels while stimulating adipogenesis (Citation122) and adipocyte differentiation (Citation123). Adipocyte differentiation has been implicated in decreasing IR, since adipocyte size inversely correlates with IR (Citation124,Citation125). SCFA secondary to microbial fermentation of supplementary fiber may thus modulate IR by targeting adipose tissue metabolism.
Muscle and hepatic carbohydrate metabolism have also been implicated as possible sites of action for fiber-derived SCFA for decreasing IR. In fact, Thorburn et al. (Citation126) attributed colonic carbohydrate fermentation of barley after an evening meal to a reduction in hepatic glucose output during an oral glucose tolerance test the following morning. Rats fed psyllium showed a significant reduction in IR combined with an increase in skeletal muscle GLUT-4 transporters (Citation127). Enhanced skeletal muscle 5’ AMP-activated protein kinase in mice supplemented with butyrate was reflected with an increase in adaptive thermogenesis, fat oxidation, mitochondrial activity, and biogenesis in muscle and brown adipose as well as reduced adiposity (Citation128). Overall, available evidence suggests that SCFA produced during colonic fermentation of fibers reduces IR by mechanisms acting in adipose, liver, and muscle.
Combining fibers and prescription medications as adjunctive treatments for insulin resistance
To date, combining fiber and prescription medication for treating IR has yet to be explored. It is therefore worth noting that testing such a combination for potential additive or synergistic effects on adipose, muscle, and hepatic tissues would be highly important for developing treatment strategies for MetS. Studies outlined above support the notion that dietary fibers possess similar as well as diverse molecular targets compared to medications, suggesting that combination fiber/pharmaceutical therapies would positively modulate various facets of insulin metabolism and reduce IR.
Tomato extract and hypertension
Tomato extract as a treatment for hypertension
Having an elevated blood pressure ≥135/85 mmHg is considered a co-morbidity for MetS (Citation5). Recently, tomato extract has shown promise as a treatment for grade 1 hypertensive and pre-hypertensive patients. Following a 4 wk placebo, grade 1 hypertensive patients were given a tomato extract for 8 wks. The major constituent of the 250 mg supplement was lycopene at 15 mg/capsule suspended in tomato oil. In addition, the lycopene content in one capsule is reported to be equivalent to six large tomatoes. Other substances included vitamin E (6 mg), β-carotene (0.4 mg), and PS (1.5 mg). The tomato-based supplement reduced systolic (SBP) and diastolic blood pressure (DBP) 7% and 6%, respectively (Citation129). When given the placebo for a second time, blood pressure reverted back to levels observed prior to receiving the tomato-based treatment, strongly suggesting that the tomato extract was the efficacious agent (Citation129). In a follow-up study by Paran et al. (Citation2), the same tomato-derived supplement reduced SBP from 139.4 mmHg to 130.0 mmHg (26%) and DBP from 79.8 to 76.0 (-5%). Subjects who received the tomato supplement during the first treatment phase had reduced SBP and DBP, only to have BP increase during phase two while on the placebo. The reverse was observed in patients receiving the placebo followed by the treatment. Researchers also noted a negative correlation between blood lycopene levels and SBP (Citation2). In addition to hypertension being an risk factor for myocardial infarction (MI), results from the EURAMIC study concluded that lycopene was the only carotenoid that significantly and independently lowered the risk of MI, producing an odds ratio of 0.5 (Citation130). A recent investigation into the relationship between lipid-soluble antioxidants and atherosclerosis risk showed a negative correlation between lycopene and carotid intima-media thickness in hypertensive patients (Citation131). These data demonstrate the potential for using tomato extracts for the treatment of hypertension.
Medications versus tomato extract for the treatment of hypertension: mechanism of action
Current prescription medications used for treating hypertension include angiotensin-converting enzyme (ACE) inhibitors, angiotensin II receptor antagonists, calcium blockers, β-blockers, and thiazides (). ACE inhibitors prevent the production of angiotensin II, a potent vasoconstrictor (Citation132). Angiotensin II receptor antagonists block angiotensin II from binding to its receptor in vascular smooth muscle, while calcium blockers decrease cardiac and arteriole muscle contraction. β-Blockers reduce cardiac output, inhibit kidney-mediated renin release, and inhibit vasoconstriction (Citation132). Finally, thiazides are diuretics that inhibit sodium reabsorption in the kidney, promoting diuresis and a decrease in blood volume (Citation132).
Table VI. Summary comparison of blood pressure-lowering mechanisms between lycopene and tomato extracts and prescription medications.
Researchers hypothesize that high levels of antioxidants found in tomato extracts account for their hypotensive properties (Citation2,Citation129) (). Pro-oxidation is a known risk factor for hypertension, with nicotinamide adenine dinucleotide phosphate (NADPH) oxidase, xanthin oxidase, mitochondrial-derived oxidants, and uncoupled endothelial nitric oxide synthase being amongst a few of the contributors to the production of reactive oxygen species (Citation133). Lycopene is considered the most potent antioxidant amongst carotenoids (Citation134). Bose et al. (Citation135) showed that 200 g/d cooked tomatoes improved antioxidant defense systems in hypertensive patients by increasing activities of superoxide dismutase, glutathione, glutathione reductase, and glutathione peroxidase. A recent editorial proposed that inhibition of peroxynitrate formation secondary to nitric oxide oxidation as a plausible mechanism for tomato's hypotensive effects since nitric oxide is a known vasodilator (Citation136). Furthermore, mice fed an atherosclerotic diet with tomato had reduced lipid peroxide levels and maintained acetylcholine-mediated vasodilation compared to mice not receiving tomato (Citation137).
Lycopene may work in concert with other tomato-derived bioactives including flavonoids, vitamins, other carotenoids, and minerals, such as potassium, and may produce better results than supplements with lycopene alone (Citation2,Citation136). A study examining the phytochemical content of the DASH diet attributed its hypotensive effects to higher levels of flavonoids and carotenoids, including lycopene (Citation138), indicating high intakes of tomatoes. In general, the antioxidant action of tomato extract ascribes to its hypotensive effects. Additional research is, however, needed to define specific mechanisms of action.
Medications versus tomato extract as adjunctive therapies for hypertension
To date, only one study has examined the combination of hypotensive medications and tomato extracts for treatment of hypertension. The Paran et al. (Citation2) study described above recruited subjects on low-dose calcium blockers and angiotensin-converting enzyme inhibitors alone, or in combination with diuretics. In the same study, it should be noted that the 6% and 5% reductions in SBP and DBP observed with tomato extract are comparable to average reductions in SBP and DBP with prescription medications at 8.8% and 4.4% for thiazides, 9.2% and 6.7% for β-blockers, 8.5% and 4.7% for ACE inhibitors, 10.3% and 5.7% for angiotensin II-converting enzyme antagonists, and 8.8 and 5.9% for calcium blockers, respectively (Citation139)—thus suggesting that concentrated tomato extract can complement hypotensive medications. Although current research regarding tomato extract as a treatment for hypertension is still in its infant stages, present evidence suggests that the hypotensive mechanisms of tomato extracts are distinct from those induced by pharmaceutical agents and their combination could be additive.
Safety and regulatory aspects of implementing functional foods and nutraceuticals as adjunctive therapies to pharmacotherapy
In the present review, FFN have been described as efficacious adjuncts to pharmaceuticals in treating disease. Hence, concerns over safety are well founded, especially since FFN modulate biological pathways. The position that a biological compound is safe because it is ‘natural’ or ‘food-derived’ is misleading. Hence, it is our position that therapeutic FFN must be evaluated by the appropriate regulatory agencies to establish dosages for efficacy and toxicity, indications for treatment, FFN–drug and FFN–food interactions, and distribution. The last-mentioned is a complicated issue. Part of the FFN appeal is their potential for circumventing high costs associated with pharmaco-monotherapy (Citation140) and, in some instances, the need for a physician's prescription. However, the question remains: ‘should FFN only be available through a physician, or incorporated into food products for purchase in supermarkets?’ Obviously, safety is a corner-stone to this debate and, as for medications that are available over the counter or with a prescription, FFN must be evaluated on an individual basis regarding efficacy, effectiveness, and ultimately safety. Indeed, regulatory agencies are beginning to recognize FFN as therapeutic agents. Canada, Europe, China, Japan, and the United States have all implemented stringent regulatory guidelines for health claims surrounding FFN (Citation141–146). However, for FFN to be recognized as treatments for disease, regulatory agencies responsible for approving drugs should also bear the responsibility for evaluating therapeutic FFN. As described, the recent approval of Lovaza®, a fish-oil supplement that is available in the United States only with a prescription with an indication for treating HTG, is evidence that FFN are gaining recognition by the medical community.
Regarding the FFN described in the present review, both fish-oil and PS have undergone rigorous assessment by regulatory agencies. In addition to their availability with a prescription in the United States, fish-oil supplements are also sold over the counter. Moreover, DHA and EPA are added to foods as ingredients. Whether fish-oil supplements are consumed with or without a prescription is dependent on how the fish-oil will be utilized. As prescription fish-oil supplements are indicated as a treatment for HTG, over-the-counter supplements and functional foods containing fish-oil are likely utilized by the public for the purpose of preventing disease.
Many countries, including the United States, the European Union, Australia, Japan, Switzerland, South Africa, Turkey, Israel, Norway, and Brazil, have approved PS as a safe LDL-C-lowering agent and allow PS to be incorporated into food-based matrices for sale in supermarkets. Thus, the next steps are to heighten the medical community's awareness to PS LDL-C-lowering efficacy so that they are implemented as a key therapy in treatment regimens for HCH. As for fiber and tomato extracts, their use as insulin sensitizers and hypotensive agents represent newer developments in FFN research and have yet to undergo reviews for health claims. The current literature suggests that doses of lycopene and fiber discussed in the present review possess minimal safety concerns (Citation147,Citation148).
Similar to prescription medications, FFN–food interactions must be thoroughly evaluated by regulatory agencies. Rats fed high doses of lycopene alongside alcohol demonstrated greater levels of hepatic CYP2E1 protein, TNF-α mRNA, and histological evidence of hepatic inflammation compared to rats fed lycopene alone (Citation149). Given that FFN will likely be utilized as adjuncts to prescription medications, FFN–drug indications must also be examined. Grapefruit is well known for its ability to produce drug toxicity when combined with certain medicines (Citation150). A recent review has identified over 80 herbal remedies that demonstrate ill effects when taken together with certain prescription medications (Citation151). Further work is required in this area.
The debate surrounding the regulatory aspects and logistics for implementation of FFN into treatment guidelines is beyond the scope of the present review. Using MetS as an example, the goal of this review was to demonstrate that FFN are viable, efficacious adjuncts to pharmacotherapy that deserve attention by the medical community. Nonetheless, new efficacious FFN entities must be evaluated by regulatory agencies, establishing dosages, proper utilization by physicians and patients, as well as safety to ensure FFN are implemented to treatment guidelines with confidence.
Summary and conclusions
The present review demonstrates that FFN should be viewed as more than life-style interventions, but as adjunctive therapies to pharmacological agents for treating disease. In addition, this review substantiates FFN ability to target molecular processes that foster the development of disease. In the context of MetS, MOM-3 modulate the expression of transcription factors that affect TG metabolism. Consequently, studies repeatedly show that combination MOM-3/statin therapy is more effective than statins alone for treating HTG. MOM-3 also improve overall vascular health, a risk factor for hypertension. Over 50 years of research supports the use of PS for treating HCH. Similar to MOM-3, therapeutic strategies that utilize both PS and statins are more effective than statins alone for reducing circulating LDL-C levels, a primary target for decreasing atherogenic dyslipidemia. Moreover, Volpe et al. (Citation152) suggest that the use of PS may delay the onset or reduce the dosage of prescriptions required for treating HCH. The observation that fiber-fermentation products modulate IR at multiple sites of glucose disposal supports the notion that the benefits of fiber consumption extend beyond that of gastrointestinal health. Although studies that examine fiber/metformin and fiber/thiazolidinedione therapies are required, it is hypothesized that fiber intake would complement pharmacological strategies for treating IR since fiber and corresponding medications target different facets of IR and glucose disposal. Finally, the observation that tomato extracts lower blood pressure to the same extent as hypertensive medication is encouraging. Even more encouraging is the observation that tomato extracts were able to reduce hypertension in subjects already taking one or more hypertensive medications.
In conclusion, efficacious FFN can modulate clinical end-points associated with disease. Thus, FFN should be emphasized throughout all stages of treatment as adjuncts to pharmacotherapy. For this to happen, however, efficacious FFN must be acknowledged by medical and regulatory agencies and undergo proper review to ensure their safety. In the end, new developments in FFN research must be communicated to health care practitioners so that they may be utilized by modern medicine as tools for combating disease.
Declaration of interest: The authors report no conflicts of interest. The authors alone are responsible for the content and writing of the paper.
References
- Maki KC, McKenney JM, Reeves MS, Lubin BC, Dicklin MR. Effects of adding prescription omega-3 acid ethyl esters to simvastatin (20 mg/day) on lipids and lipoprotein particles in men and women with mixed dyslipidemia. Am J Cardiol. 2008;102:429–33.
- Paran E, Novack V, Engelhard YN, Hazan-Halevy I. The effects of natural antioxidants from tomato extract in treated but uncontrolled hypertensive patients. Cardiovasc Drugs Ther. 2009;23:145–51.
- Blair SN, Capuzzi DM, Gottlieb SO, Nguyen T, Morgan JM, Cater NB. Incremental reduction of serum total cholesterol and low-density lipoprotein cholesterol with the addition of plant stanol ester-containing spread to statin therapy. Am J Cardiol. 2000;86:46–52.
- Ervin RB. Prevalence of metabolic syndrome among adults 20 years of age and over, by sex, age, race and ethnicity, and body mass index: United States, 2003–2006. Natl Health Stat Report. 2009;1–8.
- Cornier MA, Dabelea D, Hernandez TL, Lindstrom RC, Steig AJ, Stob NR, . The metabolic syndrome. Endocr Rev. 2008;29:777–822.
- Grundy SM, Cleeman JI, Daniels SR, Donato KA, Eckel RH, Franklin BA, . Diagnosis and management of the metabolic syndrome: an American Heart Association/National Heart, Lung, and Blood Institute Scientific Statement. Circulation. 2005;112:2735–52.
- Jehle AJ. Third Report of the National Cholesterol Education Program (NCEP) Expert Panel on Detection, Evaluation, and Treatment of High Blood Cholesterol in Adults (Adult Treatment Panel III). National Institutes of Health and National Heart, Lung, and Blood Institute; 2002.
- Senti M, Tomas M, Fito M, Weinbrenner T, Covas MI, Sala J, . Antioxidant paraoxonase 1 activity in the metabolic syndrome. J Clin Endocrinol Metab. 2003;88:5422–6.
- Roberts CK, Sindhu KK. Oxidative stress and metabolic syndrome. Life Sci. 2009;84:705–12.
- Chobanian AV, Bakris GL, Black HR, Cushman WC, Green LA, Izzo JL Jr, . The Seventh Report of the Joint National Committee on Prevention, Detection, Evaluation, and Treatment of High Blood Pressure: the JNC 7 report. JAMA. 2003;289:2560–72.
- Kris-Etherton PM, Harris WS, Appel LJ. Fish consumption, fish oil, omega-3 fatty acids, and cardiovascular disease. Arterioscler Thromb Vasc Biol. 2003;23:e20–30.
- Blonk MC, Bilo HJ, Nauta JJ, Popp-Snijders C, Mulder C, Donker AJ. Dose-response effects of fish-oil supplementation in healthy volunteers. Am J Clin Nutr. 1990;52: 120–7.
- Davidson MH, Maki KC, Kalkowski J, Schaefer EJ, Torri SA, Drennan KB. Effects of docosahexaenoic acid on serum lipoproteins in patients with combined hyperlipidemia: a randomized, double-blind, placebo-controlled trial. J Am Coll Nutr. 1997;16:236–43.
- Harris WS, Rothrock DW, Fanning A, Inkeles SB, Goodnight SH Jr, Illingworth DR, . Fish oils in hypertriglyceridemia: a dose-response study. Am J Clin Nutr. 1990;51: 399–406.
- Meyer BJ, Hammervold T, Rustan AC, Howe PR. Dose-dependent effects of docosahexaenoic acid supplementation on blood lipids in statin-treated hyperlipidaemic subjects. Lipids. 2007;42:109–15.
- Harris WS. n-3 fatty acids and serum lipoproteins: human studies. Am J Clin Nutr. 1997;65:1645S–54S.
- Dyerberg J, Bang HO. Haemostatic function and platelet polyunsaturated fatty acids in Eskimos. Lancet. 1979;2:433–5.
- Auwerx J, Schoonjans K, Fruchart JC, Staels B. Regulation of triglyceride metabolism by PPARs: fibrates and thiazolidinediones have distinct effects. J Atheroscler Thromb. 1996;3:81–9.
- Staels B, Dallongeville J, Auwerx J, Schoonjans K, Leitersdorf E, Fruchart JC. Mechanism of action of fibrates on lipid and lipoprotein metabolism. Circulation. 1998;98: 2088–93.
- Hertz R, Sheena V, Kalderon B, Berman I, Bar-Tana J. Suppression of hepatocyte nuclear factor-4alpha by acyl-CoA thioesters of hypolipidemic peroxisome proliferators. Biochem Pharmacol. 2001;61:1057–62.
- Davidson MH. Mechanisms for the hypotriglyceridemic effect of marine omega-3 fatty acids. Am J Cardiol. 2006;98: 27i–33i.
- Kim HJ, Takahashi M, Ezaki O. Fish oil feeding decreases mature sterol regulatory element-binding protein 1 (SREBP-1) by down-regulation of SREBP-1c mRNA in mouse liver. A possible mechanism for down-regulation of lipogenic enzyme mRNAs. J Biol Chem. 1999;274:25892–8.
- Lund EK, Harvey LJ, Ladha S, Clark DC, Johnson IT. Effects of dietary fish oil supplementation on the phospholipid composition and fluidity of cell membranes from human volunteers. Ann Nutr Metab. 1999;43:290–300.
- Ge CJ, Lu SZ, Chen YD, Wu XF, Hu SJ, Ji Y. Synergistic effect of amlodipine and atorvastatin on blood pressure, left ventricular remodeling, and C-reactive protein in hypertensive patients with primary hypercholesterolemia. Heart Vessels. 2008;23:91–5.
- Mastalerz-Migas A, Reksa D, Pokorski M, Steciwko A, Muszynska A, Bunio A, . Comparison of a statin vs. hypolipemic diet on the oxidant status in hemodialyzed patients with chronic renal failure. J Physiol Pharmacol. 2007;58 Suppl 5:363–70.
- Cangemi R, Loffredo L, Carnevale R, Pignatelli P, Violi F. Statins enhance circulating vitamin E. Int J Cardiol. 2008; 123:172–4.
- Weitberg AB. The antioxidant effect of lovastatin on phagocyte-induced DNA damage: implications for cancer prevention. J Exp Clin Cancer Res. 2007;26:583–6.
- Orem C, Orem A, Uydu HA, Celik S, Erdol C, Kural BV. The effects of lipid-lowering therapy on low-density lipoprotein auto-antibodies: relationship with low-density lipoprotein oxidation and plasma total antioxidant status. Coron Artery Dis. 2002;13:65–71.
- Molcanyiova A, Stancakova A, Javorsky M, Tkac I. Beneficial effect of simvastatin treatment on LDL oxidation and antioxidant protection is more pronounced in combined hyperlipidemia than in hypercholesterolemia. Pharmacol Res. 2006;54:203–7.
- Muacevic-Katanec D, Bradamante V, Poljicanin T, Reiner Z, Babic Z, Simeon-Rudolf V, . Clinical study on the effect of simvastatin on paraoxonase activity. Arzneimittelforschung. 2007;57:647–53.
- Shin MJ, Chung N, Lee JH, Jang Y, Park E, Jeon KI, . Effects of simvastatin on plasma antioxidant status and vitamins in hypercholesterolemic patients. Int J Cardiol. 2007;118:173–7.
- de Sotomayor MA, Perez-Guerrero C, Herrrera MD, Jimenez L, Marin R, Marhuenda E, . Improvement of age-related endothelial dysfunction by simvastatin: effect on NO and COX pathways. Br J Pharmacol. 2005;146:1130–8.
- Branchi A, Fiorenza AM, Torri A, Muzio F, Berra C, Colombo E, . Effects of low doses of simvastatin and atorvastatin on high-density lipoprotein cholesterol levels in patients with hypercholesterolemia. Clin Ther. 2001;23: 851–7.
- Wierzbicki AS, Mikhailidis DP. Dose-response effects of atorvastatin and simvastatin on high-density lipoprotein cholesterol in hypercholesterolaemic patients: a review of five comparative studies. Int J Cardiol. 2002;84:53–7.
- Ai M, Otokozawa S, Asztalos BF, Nakajima K, Stein E, Jones PH, . Effects of maximal doses of atorvastatin versus rosuvastatin on small dense low-density lipoprotein cholesterol levels. Am J Cardiol. 2008;101:315–8.
- Empen K, Geiss HC, Lehrke M, Otto C, Schwandt P, Parhofer KG. Effect of atorvastatin on lipid parameters, LDL subtype distribution, hemorrheological parameters and adhesion molecule concentrations in patients with hypertriglyceridemia. Nutr Metab Cardiovasc Dis. 2003;13:87–92.
- Guerin M, Egger P, Soudant C, Le Goff W, van Tol A, Dupuis R, . Dose-dependent action of atorvastatin in type IIB hyperlipidemia: preferential and progressive reduction of atherogenic apoB-containing lipoprotein subclasses (VLDL-2, IDL, small dense LDL) and stimulation of cellular cholesterol efflux. Atherosclerosis. 2002;163:287–96.
- Karalis DG, Ishisaka DY, Luo D, Ntanios F, Wun CC. Effects of increasing doses of atorvastatin on the atherogenic lipid subclasses commonly associated with hypertriglyceridemia. Am J Cardiol. 2007;100:445–9.
- Wilson PW. Evidence of systemic inflammation and estimation of coronary artery disease risk: a population perspective. Am J Med. 2008;121:S15–20.
- Serafini M, Villano D, Spera G, Pellegrini N. Redox molecules and cancer prevention: the importance of understanding the role of the antioxidant network. Nutr Cancer. 2006;56:232–40.
- Federico A, Morgillo F, Tuccillo C, Ciardiello F, Loguercio C. Chronic inflammation and oxidative stress in human carcinogenesis. Int J Cancer. 2007;121:2381–6.
- Kesavulu MM, Kameswararao B, Apparao C, Kumar EG, Harinarayan CV. Effect of omega-3 fatty acids on lipid peroxidation and antioxidant enzyme status in type 2 diabetic patients. Diabetes Metab. 2002;28:20–6.
- Rhodes LE, Shahbakhti H, Azurdia RM, Moison RM, Steenwinkel MJ, Homburg MI, . Effect of eicosapentaenoic acid, an omega-3 polyunsaturated fatty acid, on UVR-related cancer risk in humans. An assessment of early genotoxic markers. Carcinogenesis. 2003;24:919–25.
- Mori TA, Puddey IB, Burke V, Croft KD, Dunstan DW, Rivera JH, . Effect of omega 3 fatty acids on oxidative stress in humans: GC-MS measurement of urinary F2-isoprostane excretion. Redox Rep. 2000;5:45–6.
- Schmitz G, Ecker J. The opposing effects of n-3 and n-6 fatty acids. Prog Lipid Res. 2008;47:147–55.
- Sametz W, Jeschek M, Juan H, Wintersteiger R. Influence of polyunsaturated fatty acids on vasoconstrictions induced by 8-iso-PGF(2alpha) and 8-iso-PGE(2). Pharmacology. 2000; 60:155–60.
- Nomura S, Kanazawa S, Fukuhara S. Effects of eicosapentaenoic acid on platelet activation markers and cell adhesion molecules in hyperlipidemic patients with Type 2 diabetes mellitus. J Diabetes Complications. 2003;17:153–9.
- Kelley DS, Siegel D, Vemuri M, Mackey BE. Docosahexaenoic acid supplementation improves fasting and postprandial lipid profiles in hypertriglyceridemic men. Am J Clin Nutr. 2007;86:324–33.
- Nordoy A, Hansen JB, Brox J, Svensson B. Effects of atorvastatin and omega-3 fatty acids on LDL subfractions and postprandial hyperlipemia in patients with combined hyperlipemia. Nutr Metab Cardiovasc Dis. 2001;11:7–16.
- Kelley DS, Siegel D, Vemuri M, Chung GH, Mackey BE. Docosahexaenoic acid supplementation decreases remnant-like particle-cholesterol and increases the (n-3) index in hypertriglyceridemic men. J Nutr. 2008;138:30–5.
- Contacos C, Barter PJ, Sullivan DR. Effect of pravastatin and omega-3 fatty acids on plasma lipids and lipoproteins in patients with combined hyperlipidemia. Arterioscler Thromb. 1993;13:1755–62.
- Davidson MH, Stein EA, Bays HE, Maki KC, Doyle RT, Shalwitz RA, . Efficacy and tolerability of adding prescription omega-3 fatty acids 4 g/d to simvastatin 40 mg/d in hypertriglyceridemic patients: an 8-week, randomized, double-blind, placebo-controlled study. Clin Ther. 2007;29: 1354–67.
- Durrington PN, Bhatnagar D, Mackness MI, Morgan J, Julier K, Khan MA, . An omega-3 polyunsaturated fatty acid concentrate administered for one year decreased triglycerides in simvastatin treated patients with coronary heart disease and persisting hypertriglyceridaemia. Heart. 2001; 85:544–8.
- Hong H, Xu ZM, Pang BS, Cui L, Wei Y, Guo WJ, . Effects of simvastain combined with omega-3 fatty acids on high sensitive C-reactive protein, lipidemia, and fibrinolysis in patients with mixed dyslipidemia. Chin Med Sci J. 2004;19: 145–9.
- Nordoy A, Bonaa KH, Nilsen H, Berge RK, Hansen JB, Ingebretsen OC. Effects of simvastatin and omega-3 fatty acids on plasma lipoproteins and lipid peroxidation in patients with combined hyperlipidaemia. J Intern Med. 1998;243:163–70.
- Valdivielso P, Rioja J, Garcia-Arias C, Sanchez-Chaparro MA, Gonzalez-Santos P. Omega 3 fatty acids induce a marked reduction of apolipoprotein B48 when added to fluvastatin in patients with type 2 diabetes and mixed hyperlipidemia: a preliminary report. Cardiovasc Diabetol. 2009;8:1.
- Zeman M, Zak A, Vecka M, Tvrzicka E, Pisarikova A, Stankova B. N-3 fatty acid supplementation decreases plasma homocysteine in diabetic dyslipidemia treated with statin-fibrate combination. J Nutr Biochem. 2006;17: 379–84.
- Nomura S, Inami N, Shouzu A, Omoto S, Kimura Y, Takahashi N, . The effects of pitavastatin, eicosapentaenoic acid and combined therapy on platelet-derived microparticles and adiponectin in hyperlipidemic, diabetic patients. Platelets. 2009;20:16–22.
- Chen H, Montagnani M, Funahashi T, Shimomura I, Quon MJ. Adiponectin stimulates production of nitric oxide in vascular endothelial cells. J Biol Chem. 2003;278:45021–6.
- Hattori Y, Suzuki M, Hattori S, Kasai K. Globular adiponectin upregulates nitric oxide production in vascular endothelial cells. Diabetologia. 2003;46:1543–9.
- Ouchi N, Kihara S, Arita Y, Maeda K, Kuriyama H, Okamoto Y, . Novel modulator for endothelial adhesion molecules: adipocyte-derived plasma protein adiponectin. Circulation. 1999;100:2473–6.
- Best MM, Duncan CH, Van Loon EJ, Wathen JD. Lowering of serum cholesterol by the administration of a plant sterol. Circulation. 1954;10:201–6.
- Berger A, Jones PJ, Abumweis SS. Plant sterols: factors affecting their efficacy and safety as functional food ingredients. Lipids Health Dis. 2004;3:5.
- Abumweis SS, Barake R, Jones PJ. Plant sterols/stanols as cholesterol lowering agents: A meta-analysis of randomized controlled trials. Food Nutr Res. 2008;52 doi: 10.3402/fnr.v52i0.1811. Epub 2008 Aug 18.
- Goldberg AC, Ostlund RE Jr, Bateman JH, Schimmoeller L, McPherson TB, Spilburg CA. Effect of plant stanol tablets on low-density lipoprotein cholesterol lowering in patients on statin drugs. Am J Cardiol. 2006;97:376–9.
- McPherson TB, Ostlund RE, Goldberg AC, Bateman JH, Schimmoeller L, Spilburg CA. Phytostanol tablets reduce human LDL-cholesterol. J Pharm Pharmacol. 2005;57:889–96.
- Hou R, Goldberg AC. Lowering low-density lipoprotein cholesterol: statins, ezetimibe, bile acid sequestrants, and combinations: comparative efficacy and safety. Endocrinol Metab Clin North Am. 2009;38:79–97.
- Pandian A, Arora A, Sperling LS, Khan BV. Targeting multiple dyslipidemias with fixed combinations–focus on extended release niacin and simvastatin. Vasc Health Risk Manag. 2008;4:1001–9.
- Marinangeli CP, Varady KA, Jones PJ. Plant sterols combined with exercise for the treatment of hypercholesterolemia: overview of independent and synergistic mechanisms of action. J Nutr Biochem. 2006;17:217–24.
- Ostlund RE Jr, McGill JB, Zeng CM, Covey DF, Stearns J, Stenson WF, . Gastrointestinal absorption and plasma kinetics of soy Delta(5)-phytosterols and phytostanols in humans. Am J Physiol Endocrinol Metab. 2002;282:E911–6.
- Plat J, Nichols JA, Mensink RP. Plant sterols and stanols: effects on mixed micellar composition and LXR (target gene) activation. J Lipid Res. 2005;46:2468–76.
- Plat J, Mensink RP. Increased intestinal ABCA1 expression contributes to the decrease in cholesterol absorption after plant stanol consumption. FASEB J. 2002;16:1248–53.
- Shin WS, Szuba A, Rockson SG. The role of chemokines in human cardiovascular pathology: enhanced biological insights. Atherosclerosis. 2002;160:91–102.
- Bustos C, Hernandez-Presa MA, Ortego M, Tunon J, Ortega L, Perez F, . HMG-CoA reductase inhibition by atorvastatin reduces neointimal inflammation in a rabbit model of atherosclerosis. J Am Coll Cardiol. 1998;32:2057–64.
- Lewandowski M, Kornacewicz-Jach Z, Millo B, Zielonka J, Czechowska M, Kaliszczak R, . The influence of low dose atorvastatin on inflammatory marker levels in patients with acute coronary syndrome and its potential clinical value. Cardiol J. 2008;15:357–64.
- Ortego M, Bustos C, Hernandez-Presa MA, Tunon J, Diaz C, Hernandez G, . Atorvastatin reduces NF-kappaB activation and chemokine expression in vascular smooth muscle cells and mononuclear cells. Atherosclerosis. 1999; 147: 253–61.
- Bustos P, Duffau C, Pacheco C, Ulloa N. beta-Sitosterol modulation of monocyte-endothelial cell interaction: a comparison to female hormones. Maturitas. 2008;60:202–8.
- Castro Cabezas M, de Vries JH, Van Oostrom AJ, Iestra J, van Staveren WA. Effects of a stanol-enriched diet on plasma cholesterol and triglycerides in patients treated with statins. J Am Diet Assoc. 2006;106:1564–9.
- de Jong A, Plat J, Lutjohann D, Mensink RP. Effects of long-term plant sterol or stanol ester consumption on lipid and lipoprotein metabolism in subjects on statin treatment. Br J Nutr. 2008;100:937–41.
- Ketomaki A, Gylling H, Miettinen TA. Non-cholesterol sterols in serum, lipoproteins, and red cells in statin-treated FH subjects off and on plant stanol and sterol ester spreads. Clin Chim Acta. 2005;353:75–86.
- Simons LA. Additive effect of plant sterol-ester margarine and cerivastatin in lowering low-density lipoprotein cholesterol in primary hypercholesterolemia. Am J Cardiol. 2002;90:737–40.
- Takeshita M, Katsuragi Y, Kusuhara M, Higashi K, Miyajima E, Mizuno K, . Phytosterols dissolved in diacylglycerol oil reinforce the cholesterol-lowering effect of low-dose pravastatin treatment. Nutr Metab Cardiovasc Dis. 2008;18: 483–91.
- Jakulj L, Trip MD, Sudhop T, von Bergmann K, Kastelein JJ, Vissers MN. Inhibition of cholesterol absorption by the combination of dietary plant sterols and ezetimibe: effects on plasma lipid levels. J Lipid Res. 2005;46:2692–8.
- Nigon F, Serfaty-Lacrosniere C, Beucler I, Chauvois D, Neveu C, Giral P, . Plant sterol-enriched margarine lowers plasma LDL in hyperlipidemic subjects with low cholesterol intake: effect of fibrate treatment. Clin Chem Lab Med. 2001;39:634–40.
- Ginsberg HN. Insulin resistance and cardiovascular disease. J Clin Invest. 2000;106:453–8.
- Watson KE, Peters Harmel AL, Matson G. Atherosclerosis in type 2 diabetes mellitus: the role of insulin resistance. J Cardiovasc Pharmacol Ther. 2003;8:253–60.
- Rupp H. Insulin resistance, hyperinsulinemia, and cardiovascular disease. The need for novel dietary prevention strategies. Basic Res Cardiol. 1992;87:99–105.
- Valachovicova M, Krajcovicova-Kudlackova M, Blazicek P, Babinska K. No evidence of insulin resistance in normal weight vegetarians. A case control study. Eur J Nutr. 2006;45:52–4.
- McKeown NM, Meigs JB, Liu S, Saltzman E, Wilson PW, Jacques PF. Carbohydrate nutrition, insulin resistance, and the prevalence of the metabolic syndrome in the Framingham Offspring Cohort. Diabetes Care. 2004;27: 538–46.
- Lau C, Faerch K, Glumer C, Tetens I, Pedersen O, Carstensen B, . Dietary glycemic index, glycemic load, fiber, simple sugars, and insulin resistance: the Inter99 study. Diabetes Care. 2005;28:1397–403.
- Robertson MD, Bickerton AS, Dennis AL, Vidal H, Frayn KN. Insulin-sensitizing effects of dietary resistant starch and effects on skeletal muscle and adipose tissue metabolism. Am J Clin Nutr. 2005;82:559–67.
- Weickert MO, Mohlig M, Schofl C, Arafat AM, Otto B, Viehoff H, . Cereal fiber improves whole-body insulin sensitivity in overweight and obese women. Diabetes Care. 2006;29:775–80.
- Liatis S, Tsapogas P, Chala E, Dimosthenopoulos C, Kyriakopoulos K, Kapantais E, . The consumption of bread enriched with betaglucan reduces LDL-cholesterol and improves insulin resistance in patients with type2 diabetes. Diabetes Metab. 2009;35:115–20.
- Rave K, Roggen K, Dellweg S, Heise T, tom Dieck H. Improvement of insulin resistance after diet with a whole-grain based dietary product: results of a randomized, controlled cross-over study in obese subjects with elevated fasting blood glucose. Br J Nutr. 2007;98:929–36.
- Ostman EM, Frid AH, Groop LC, Bjorck IM. A dietary exchange of common bread for tailored bread of low glycaemic index and rich in dietary fibre improved insulin economy in young women with impaired glucose tolerance. Eur J Clin Nutr. 2006;60:334–41.
- Robertson MD, Currie JM, Morgan LM, Jewell DP, Frayn KN. Prior short-term consumption of resistant starch enhances postprandial insulin sensitivity in healthy subjects. Diabetologia. 2003;46:659–65.
- Kohl A, Gogebakan O, Mohlig M, Osterhoff M, Isken F, Pfeiffer AF, . Increased interleukin-10 but unchanged insulin sensitivity after 4 weeks of (1, 3)(1, 6)-beta-glycan consumption in overweight humans. Nutr Res. 2009;29:248–54.
- Kim H, Stote KS, Behall KM, Spears K, Vinyard B, Conway JM. Glucose and insulin responses to whole grain breakfasts varying in soluble fiber, beta-glucan: a dose response study in obese women with increased risk for insulin resistance. Eur J Nutr. 2009;48:170–5.
- Fryer LG, Parbu-Patel A, Carling D. The Anti-diabetic drugs rosiglitazone and metformin stimulate AMP-activated protein kinase through distinct signaling pathways. J Biol Chem. 2002;277:25226–32.
- Schimmack G, Defronzo RA, Musi N. AMP-activated protein kinase: Role in metabolism and therapeutic implications. Diabetes Obes Metab. 2006;8:591–602.
- Zhou G, Myers R, Li Y, Chen Y, Shen X, Fenyk-Melody J, . Role of AMP-activated protein kinase in mechanism of metformin action. J Clin Invest. 2001;108:1167–74.
- Santos RF, Nomizo R, Oliveira E, Ursich M, Wajchenberg B, Reaven GM, . Erythrocyte insulin receptor tyrosine kinase activity is increased in glyburide-treated patients with type 2 diabetes in good glycaemic control. Diabetes Obes Metab. 2000;2:237–41.
- Santos RF, Nomizo R, Wajhenberg BL, Reaven GM, Azhar S. Changes in insulin receptor tyrosine kinase activity associated with metformin treatment of type 2 diabetes. Diabetes Metab. 1995;21:274–80.
- Stith BJ, Goalstone ML, Espinoza R, Mossel C, Roberts D, Wiernsperger N. The antidiabetic drug metformin elevates receptor tyrosine kinase activity and inositol 1,4,5-trisphosphate mass in Xenopus oocytes. Endocrinology. 1996; 137: 2990–9.
- Luna V, Casauban L, Sajan MP, Gomez-Daspet J, Powe JL, Miura A, . Metformin improves atypical protein kinase C activation by insulin and phosphatidylinositol-3,4,5-(PO4)3 in muscle of diabetic subjects. Diabetologia. 2006;49:375–82.
- Fediuc S, Pimenta AS, Gaidhu MP, Ceddia RB. Activation of AMP-activated protein kinase, inhibition of pyruvate dehydrogenase activity, and redistribution of substrate partitioning mediate the acute insulin-sensitizing effects of troglitazone in skeletal muscle cells. J Cell Physiol. 2008;215:392–400.
- Stumvoll M, Haring HU. Glitazones: clinical effects and molecular mechanisms. Ann Med. 2002;34:217–24.
- Oakes ND, Thalen PG, Jacinto SM, Ljung B. Thiazolidinediones increase plasma-adipose tissue FFA exchange capacity and enhance insulin-mediated control of systemic FFA availability. Diabetes. 2001;50:1158–65.
- Williams LB, Fawcett RL, Waechter AS, Zhang P, Kogon BE, Jones R, . Leptin production in adipocytes from morbidly obese subjects: stimulation by dexamethasone, inhibition with troglitazone, and influence of gender. J Clin Endocrinol Metab. 2000;85:2678–84.
- Derosa G, Salvadeo SA, D’Angelo A, Fogari E, Ragonesi PD, Ciccarelli L, . Rosiglitazone therapy improves insulin resistance parameters in overweight and obese diabetic patients intolerant to metformin. Arch Med Res. 2008; 39:412–9.
- Wu ZH, Zhao SP, Chu LX, Ye HJ. Pioglitazone reduces tumor necrosis factor-alpha serum concentration and mRNA expression of adipose tissue in hypercholesterolemic rabbits. Int J Cardiol. 2010;138:151–6.
- Majuri A, Santaniemi M, Rautio K, Kunnari A, Vartiainen J, Ruokonen A, . Rosiglitazone treatment increases plasma levels of adiponectin and decreases levels of resistin in overweight women with PCOS: a randomized placebo-controlled study. Eur J Endocrinol. 2007;156:263–9.
- Jiang G, Dallas-Yang Q, Biswas S, Li Z, Zhang BB. Rosiglitazone, an agonist of peroxisome-proliferator-activated receptor gamma (PPARgamma), decreases inhibitory serine phosphorylation of IRS1 in vitro and in vivo. Biochem J. 2004;377:339–46.
- Tafuri SR. Troglitazone enhances differentiation, basal glucose uptake, and Glut1 protein levels in 3T3-L1 adipocytes. Endocrinology. 1996;137:4706–12.
- Park KS, Ciaraldi TP, Abrams-Carter L, Mudaliar S, Nikoulina SE, Henry RR. Troglitazone regulation of glucose metabolism in human skeletal muscle cultures from obese type II diabetic subjects. J Clin Endocrinol Metab. 1998;83:1636–43.
- Robertson MD. Metabolic cross talk between the colon and the periphery: implications for insulin sensitivity. Proc Nutr Soc. 2007;66:351–61.
- Carpentier A, Mittelman SD, Lamarche B, Bergman RN, Giacca A, Lewis GF. Acute enhancement of insulin secretion by FFA in humans is lost with prolonged FFA elevation. Am J Physiol. 1999;276:E1055–66.
- Barazzoni R, Zanetti M, Stulle M, Mucci MP, Pirulli A, Dore F, . Higher total ghrelin levels are associated with higher insulin-mediated glucose disposal in non-diabetic maintenance hemodialysis patients. Clin Nutr. 2008;27:142–9.
- Liu J, Lin H, Cheng P, Hu X, Lu H. Effects of ghrelin on the proliferation and differentiation of 3T3-L1 preadipocytes. J Huazhong Univ Sci Technolog Med Sci. 2009;29: 227–30.
- Pagotto U, Gambineri A, Vicennati V, Heiman ML, Tschop M, Pasquali R. Plasma ghrelin, obesity, and the polycystic ovary syndrome: correlation with insulin resistance and androgen levels. J Clin Endocrinol Metab. 2002;87:5625–9.
- Choi K, Roh SG, Hong YH, Shrestha YB, Hishikawa D, Chen C, . The role of ghrelin and growth hormone secretagogues receptor on rat adipogenesis. Endocrinology. 2003;144:754–9.
- Ge H, Li X, Weiszmann J, Wang P, Baribault H, Chen JL, . Activation of G protein-coupled receptor 43 in adipocytes leads to inhibition of lipolysis and suppression of plasma free fatty acids. Endocrinology. 2008;149: 4519–26.
- Hong YH, Nishimura Y, Hishikawa D, Tsuzuki H, Miyahara H, Gotoh C, . Acetate and propionate short chain fatty acids stimulate adipogenesis via GPCR43. Endocrinology. 2005;146:5092–9.
- Weyer C, Foley JE, Bogardus C, Tataranni PA, Pratley RE. Enlarged subcutaneous abdominal adipocyte size, but not obesity itself, predicts type II diabetes independent of insulin resistance. Diabetologia. 2000;43:1498–506.
- Lonn M, Mehlig K, Bengtsson C, Lissner L. Adipocyte size predicts incidence of type 2 diabetes in women. FASEB J. 2010.24:326–31.
- Thorburn A, Muir J, Proietto J. Carbohydrate fermentation decreases hepatic glucose output in healthy subjects. Metabolism. 1993;42:780–5.
- Song YJ, Sawamura M, Ikeda K, Igawa S, Yamori Y. Soluble dietary fibre improves insulin sensitivity by increasing muscle GLUT-4 content in stroke-prone spontaneously hypertensive rats. Clin Exp Pharmacol Physiol. 2000;27: 41–5.
- Gao Z, Yin J, Zhang J, Ward RE, Martin RJ, Lefevre M, . Butyrate improves insulin sensitivity and increases energy expenditure in mice. Diabetes. 2009.;58:1509–17.
- Engelhard YN, Gazer B, Paran E. Natural antioxidants from tomato extract reduce blood pressure in patients with grade-1 hypertension: a double-blind, placebo-controlled pilot study. Am Heart J. 2006;151:100.
- Kohlmeier L, Kark JD, Gomez-Gracia E, Martin BC, Steck SE, Kardinaal AF, . Lycopene and myocardial infarction risk in the EURAMIC Study. Am J Epidemiol. 1997;146:618–26.
- Gianetti J, Pedrinelli R, Petrucci R, Lazzerini G, De Caterina M, Bellomo G, . Inverse association between carotid intima-media thickness and the antioxidant lycopene in atherosclerosis. Am Heart J. 2002;143:467–74.
- Canadian Pharmacists Association. CPS Compendium of pharmaceuticals and specialties. 9th. Ottawa: Canadian Pharmacists Association; 2003.
- Delles C, Miller WH, Dominiczak AF. Targeting reactive oxygen species in hypertension. Antioxid Redox Signal. 2008;10:1061–77.
- Di Mascio P, Kaiser S, Sies H. Lycopene as the most efficient biological carotenoid singlet oxygen quencher. Arch Biochem Biophys. 1989;274:532–8.
- Bose KS, Agrawal BK. Effect of lycopene from tomatoes (cooked) on plasma antioxidant enzymes, lipid peroxidation rate and lipid profile in grade-I hypertension. Ann Nutr Metab. 2007;51:477–81.
- de Leeuw PW, Bast A. Tomato extract for hypertension? Editorial to ‘The effects of natural antioxidants from tomato extract in treated but uncontrolled hypertensive patients’ by E. Paran et al. Cardiovasc Drugs Ther. 2009;23: 107–8.
- Suganuma H, Inakuma T. Protective effect of dietary tomato against endothelial dysfunction in hypercholesterolemic mice. Biosci Biotechnol Biochem. 1999;63:78–82.
- Most MM. Estimated phytochemical content of the dietary approaches to stop hypertension (DASH) diet is higher than in the Control Study Diet. J Am Diet Assoc. 2004;104:1725–7.
- Law M, Wald N, Morris J. Lowering blood pressure to prevent myocardial infarction and stroke: a new preventive strategy. Health Technol Assess. 2003;7:1–94.
- Jones PJ, Asp NG, Silva P. Evidence for health claims on foods: how much is enough? Introduction and general remarks. J Nutr. 2008;138:1189S–91S.
- Asp NG, Bryngelsson S. Health claims in Europe: new legislation and PASSCLAIM for substantiation. J Nutr. 2008; 138:1210S–5S.
- Hasler CM. Health claims in the United States: an aid to the public or a source of confusion? J Nutr. 2008;138: 1216S–20S.
- L’Abbe MR, Dumais L, Chao E, Junkins B. Health claims on foods in Canada. J Nutr. 2008;138:1221S–7S.
- Tapsell LC. Evidence for health claims: a perspective from the Australia-New Zealand region. J Nutr. 2008;138: 1206S–9S.
- Yamada K, Sato-Mito N, Nagata J, Umegaki K. Health claim evidence requirements in Japan. J Nutr. 2008;138: 1192S–8S.
- Yang Y. Scientific substantiation of functional food health claims in China. J Nutr. 2008;138:1199S–205S.
- Gustin DM, Rodvold KA, Sosman JA, Diwadkar- Navsariwala V, Stacewicz-Sapuntzakis M, Viana M, . Single-dose pharmacokinetic study of lycopene delivered in a well-defined food-based lycopene delivery system (tomato paste-oil mixture) in healthy adult male subjects. Cancer Epidemiol Biomarkers Prev. 2004;13:850–60.
- Jonker D, Kuper CF, Fraile N, Estrella A, Rodriguez Otero C. Ninety-day oral toxicity study of lycopene from Blakeslea trispora in rats. Regul Toxicol Pharmacol. 2003;37: 396–406.
- Veeramachaneni S, Ausman LM, Choi SW, Russell RM, Wang XD. High dose lycopene supplementation increases hepatic cytochrome P4502E1 protein and inflammation in alcohol-fed rats. J Nutr. 2008;138:1329–35.
- Bressler R. Grapefruit juice and drug interactions. Exploring mechanisms of this interaction and potential toxicity for certain drugs. Geriatrics. 2006;61:12–8.
- Izzo AA. Herb-drug interactions: an overview of the clinical evidence. Fundam Clin Pharmacol. 2005;19:1–16.
- Volpe R, Niittynen L, Korpela R, Sirtori C, Bucci A, Fraone N, . Effects of yoghurt enriched with plant sterols on serum lipids in patients with moderate hypercholesterolaemia. Br J Nutr. 2001;86:233–9.