Abstract
Context: At variance with steroid administration, the possible effects of leukotrienes inhibition on basal respiratory mechanics and olfactory system function are still unclear.
Objective: To investigate if interference with the leukotrienes activity may influence basal lung mechanics in healthy mammals, as well as the olfactory system.
Materials and methods: We measured lung mechanics by the end-inflation occlusion method in control and in montelukast i.p. treated anaesthetised healthy mice (10 mg/kg/die for a week). A study of olfactory system histology was also conducted.
Results: Elastance and resistive properties of the lung were not affected by montelukast, while a significant increment of lung hysteresis was observed. The analysis of olfactory system histology revealed no significant effects of montelukast compared to controls.
Discussion and conclusions: Leukotrienes’ antagonism does not affect respiratory mechanics in basal conditions, except for a hysteresis increment, which might counteract the increase in expiratory flow in asthmatic subjects assuming montelukast.
Introduction
Cysteinyl-leukotrienes have been shown to exert a potent bronchoconstrictor activity in mammals and are involved in experimental and spontaneous asthma pathogenesisCitation1.
The plasma concentrations of cysteinyl-leukotrienes is increased in different inflammatory diseases, but concentrations values as high as 25–30% of those documented in inflammatory diseases have been measured in basal condition in healthy subjectsCitation2–4.
These data suggest that cysteinyl-leukotrienes may exert biological effects on lung mechanics in basal conditions also, for example contributing in the modulation of airway calibre in normal and healthy mammals.
Experimental data describing the possible effects of leukotrienes on basal lung mechanics are, however, very few in the literature. Thus, in the present experiments, we tested the hypothesis that cysteinyl-leukotriene receptors’ antagonism by montelukast may change lung mechanics in healthy mice in basal conditions.
The end-inflation occlusion method was used to study lung mechanics. This method has been widely applied in humansCitation5–7 and experimental animalsCitation8–10 including miceCitation11–14, and has been shown to obtain reliable results.
Modelling the lungs as consisting of two distinct compartments, the method permits not only the measurement of the ohmic resistance of the airway, but also of the visco-elastic resistance of the lung due to stress relaxation. Ohmic resistance is the normalised-to-flow pressure dissipation due to viscous forces opposing the airflow in the airway, as predicted by Poiseuille law. Visco-elastic resistance is the normalised-to-flow pressure dissipation due to the resistance of respiratory system tissues to deformation during inflation, which is recovered after the arrest of the inspiratory flow (stress relaxation). Lung stress relaxation, whose molecular basis are still incompletely understood, has however been shown to contribute significantly to the overall inspiratory pressure dissipationCitation5,Citation6,Citation9,Citation10, and the possible effects of leukotrienes or of leukotrienes’ antagonism have never been studied before.
Previous measurements reported an increased phosphatidylcholine secretion from type II pneumocytes in the presence of leukotrienesCitation15, and decreased surfactant production in consequence of the administration of leukotriene inhibitorsCitation16. However, no data are available describing possible effects on lung hysteresis. Thus, in the present experiments, inflation–deflation pressure–volume loops were analysed in control and montelukast-treated mice to study the possibility that leukotriene receptors’ antagonism may affect lung hysteresis.
The current asthma therapy is based on steroid treatment, but recently various cysteinyl-leukotrienes inhibitors have been proposed for the treatment of asthmatic childrenCitation17,Citation18. The leukotriene signalling has been documented in various areas of the mammalian brain, noticeably in the olfactory cortexCitation19. In addition, high leukotriene activity, similar to that of leukocytes, was detected in the olfactory bulb, suggesting a possible modulatory role of leukotrienes on the olfactory bulb neuronal functionCitation20. No reports are available on the effects of leukotriene receptors’ antagonism on the olfactory function except for the improvement in olfaction in hyposmic patients due to the reduction in inflammatory processesCitation21,Citation22.
We previously showed that chronic steroid treatment affect the olfactory functionCitation12, therefore the above mentioned data prompted us to investigate a possible effect of leukotrienes’ activity also on the olfactory system.
Here, the possible effects of leukotrienes’ antagonism on olfactory system histology have been investigated for the first time to compare the results with those observed as a consequence of steroid administration.
Methods
Animals and treatment
The experiments were approved by the local competent authorities (CEASA) and conformed to the European law on animal experiments and welfare. Adult CD/1 male mice, three months old, were used. Mice were injected i.p. daily for 7 days, with either saline (9 g/L NaCl, n = 11 controls, mean weight 46.6 ± 0.75 g) or 10 mg/kg body weight montelukast dissolved in saline (n = 11, montelukast-treated, mean weight 46.1 ± 0.8 g). Intraperitoneal montelukast administration is a well documented procedure in mammalsCitation23,Citation24, including miceCitation25.
Lung mechanics
Mice were deeply anesthetised (300 μl, 20% chloral hydrate in water i.p.) and then laid on a heated operating table. After a tracheostomy, a small polyethylene cannula (1 mm i.d, 2.5 cm long) was inserted through an incision in the second tracheal ring and firmly secured in place.
The lungs were exposed to atmospheric pressure by middle thoracotomy, and positive pressure ventilation with a 0.4 mL tidal volume and a 120/min breathing frequency (PEEP 3 cm H2O) (Rodent Ventilator 7025, Basile, Italy) was begun, and consistently maintained throughout the experiment.
Positive pressure ventilation was maintained for 10 min and lung mechanics were then measured using the end-inflation occlusion methodCitation5–7.
The ventilator was disconnected, PEEP was discontinued, and the tracheal cannula was connected to a constant flow pump (SP 2000 Series Syringe Pump sp210iw, World Precision Instruments, USA) set to deliver a tidal volume (VT) of 0.4 mL with a square wave flow (F) of 1 mL/s. The time for the rise and the fall of the flow was approximately 30 ms. The pump setting was carefully checked by directly taking measurements before beginning the experiments.
The lateral tracheal pressure proximal to the tracheal cannula was monitored (142 pc 01d, Honeywell, USA) and continuously recorded (1326 Econo Recorder, Biorad, Italy). Because abrupt changes in diameters were not present in the circuit, errors in flow resistance measurements, such as those reported by Chang and MortolaCitation26, were avoided. The frequency response of the transducer and the pressure measuring system was tested by sinusoidal forcing and found to be flat up to 20 Hz. In accordance with the literatureCitation7,Citation8, this frequency response was adequate to avoid mechanical artefacts in the pressure signal records.
The end-inflation occlusion method was utilised to determine the parameters of lung mechanics: the static elastic pressure of the lungs (Pel,l), the total resistive pressure drop (Pmax,l) and the sudden Newtonian resistive pressure drop at flow interruption (Pmin,l) were measured on adequately magnified tracings (). Pmax,l was calculated as the difference between the maximum value of pressure at end inflation (Pdyn,max) and Pel,l. Pmin,l was calculated as the difference between Pdyn, max and P1, the pressure value immediately after flow interruption ().
Figure 1. Representative tracings of the lateral tracheal pressure upon flow interruption during constant-flow inflation. The relevant pressures used for calculation of lung mechanics are indicated: the maximal pressure at the end of inflation (Pdyn,max), the pressure immediately after flow interruption (P1), the static elastic pressure of the lung (Pel,l), the pressure drop due to the ohmic lung resistance (Pmin,l) and total pressure drop which includes the effects of stress relaxation (Pmax,l).
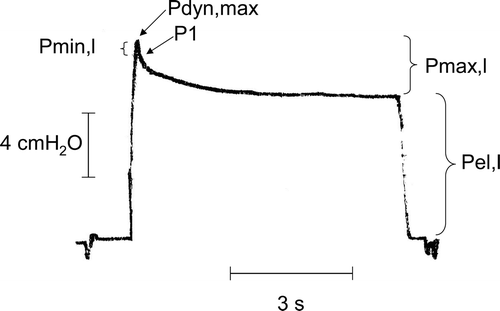
To avoid a viscous pressure component in Pmin,l, P1 values were identified by extrapolating the pressure tracings to the time the flow stoppedCitation27. Thus, Pmin,l represents the nearly instantaneous, Newtonian resistive pressure drop that theoretically occurs at infinite breathing frequencyCitation27 and is considered to be the pressure driving the inspiratory flow through the airways immediately before its interruptionCitation5,Citation6. Pmin,l does not include the visco-elastic pressure drop that results from stress relaxation. In contrast, the visco-elastic pressure drop is included in the Pmax,l value.
The mean pressure data obtained from the 3 to 5 inflations for each mice were used to calculate lung static elastance (Est,l = Pel,l/VT) and the total resistance to airflow of the lung (Rmax,l = Pmax,l/F). The total resistance value includes the Newtonian inspiratory resistance to airflow offered by the airways and the resistance due to the movement of lung tissue (Rmin,l = Pmin,l/F), and the pressure drop resulting from the effect of stress relaxation. This last component of Rmax,l was isolated and quantified as “viscous” resistance (Rvisc,l = Rmax,l – Rmin,l).
The equipment resistance, including the tracheal cannula and the standard three-way stopcock, amounted to 0.4 cmH2O/mL s−1 (Req) and was measured separately at a flow rate of 1 mL/s. All inflations were performed at a fixed flow rate of 1 mL/s, and Req was subtracted from the results, which thus represent intrinsic values.
After lung mechanics were measured, which took 3–5 min, mechanical ventilation was restored and maintained for 5 min. To obtain a constant volume history for the measurement of lung hysteresis, the lung was inflated with a 5-mL syringe to a static elastic pressure of 20–25 cmH2O three times consecutively. After that, the lungs were inflated, and subsequently deflated, five times in 0.4 mL steps using a precision glass syringe. Each volume was maintained for approximately 5–6 s at each step. Boyle’s law-related effect of air compression in the airway was neglected, because it would not be predicted to introduce any systematic error. In fact, it would be expected to affect the measurements taken in control and montelukast-treated animals equally. The pertinent static elastic pressures were measured, and the static inflation–deflation volume–pressure curves were determined. The hysteresis areas (Hy,l) were quantified using electronic digital integration and expressed as cmH2O * mL.
The above described experimental procedure, which lasted less than 1 h, was performed for both control and montelukast-treated animals, and the mean values of lung mechanics parameters were statistically compared (Mann–Whitney).
Olfactory system histology
At the end of the experiment, animals were sacrificed and their nose and brain removed and postfixed in formalin overnight. The noses were then soaked in EDTA until the bones were softened. Tissue were embedded in paraffin and cut with a microtome. Six mice per group had viable tissue for measurements in the olfactory mucosa, and seven per group for measures in the olfactory bulb. The olfactory mucosa was stained with haematoxylin–eosin, images were captured with a 40× objective using the Leica resident software. Three measures were taken, using Scion image, from each mouse in zone 1 of the olfactory mucosa. The mean value from each mouse was used to compare between groups with monovariate ANOVA (controls vs. treated mice).
The olfactory bulb were cut horizontally and stained with Nissl staining. The diameter of 5 lateral and 5 medial glomeruli were measured for each mouse, with Scion image (as above). The mean value for lateral and medial glomeruli were analysed with monovariate ANOVA (controls vs. treated mice).
Results
Lung mechanics
The mean values of lung mechanics parameters in control and montelukast-treated animals are reported in and together with the statistical significances of the differences.
Figure 2. Mean values (vertical bars represent one SE, n = 11) of static lung elastance (Est,l) in control and montelukast-treated mice are not significantly different.
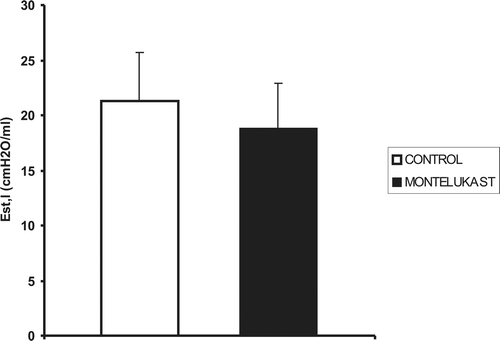
Figure 3. Mean values (vertical bars represent one SE, n = 11) of (a) total (Rmax,l), (b) ohmic airway (Rmin,l) and (c) visco-elastic resistances (Rvisc,l) in control and montelukast-treated mice are not significantly different.
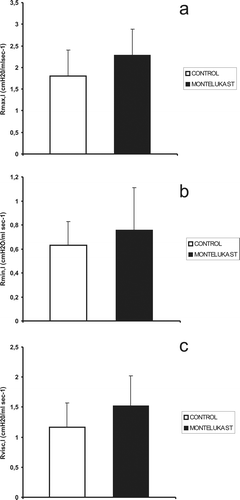
We found that montelukast treatment did not significantly affect any of the measured lung mechanics parameters, except for the extent of the surface of the inflation–deflation loops which reflects lung hysteresis ().
Olfactory system histology
The height of the olfactory mucosa did not differ between controls and montelukast-treated mice, F(1,10) = 0.0198, p = 0.891 (47.56 ± 1.58 µm vs. 47.82 ± 0.98 µm). The diameter of both medial and lateral glomeruli did not differ between control and treated mice, F(1,10) = 0.001, p = 0.974 for lateral glomeruli (12.28 ± 0.23 µm for controls and 12.27 ± 0.18 µm for treated mice), F(1,12) = 0.116, p = 0.739 for medial glomeruli (12.65 ± 0.17 µm for controls and 12.58 ± 0.13 µm for treated mice).
Discussion
Lung mechanics
The mechanical ventilation settings used during these experiments were the same as those described as “non injurious” in the literatureCitation9. In particular, “non injurious” ventilation lasting 1 h has been shown to cause no alterations in respiratory system mechanics, or modifications in hysteresisCitation9.
The mean values of lung mechanics parameters here described are comprised in the range of those previously measured by different laboratories, using the same technique in miceCitation11–14.
Previous experiments demonstrated that montelukast reduces airway resistance in experimental models of asthma or in spontaneous asthma even at much lower dosages than that used hereCitation25,Citation28–32, which hence adequately exerted receptors’ antagonism.
Cysteinyl-leukotrienes’ antagonism has been previously shown to reduce bronchoconstriction and airway resistance in animal models of asthma, as ovalbumin-sensitised guinea pigsCitation33,Citation34 or ratsCitation29,Citation30, and in spontaneous asthma in humansCitation35,Citation36. Similar results have been reported describing the reduction of leukotrienes-induced bronchoconstriction in guinea pigCitation33,Citation34,Citation37 and humansCitation38.
However, we did not find any significant difference between the mean values of lung mechanics parameters including resistive pressure dissipation measured in montelukast-treated and control mice ( and ) in basal conditions.
This result indicates that basal cysteinyl-leukotrienes plasma concentration does not affect lung elastance or any of the measured resistive pressure dissipations in healthy mice. In particular, viscous resistance, which primarily reflects stress relaxationCitation5,Citation6, is not affected by leukotrienes’ antagonism in basal condition.
Stress relaxation is a complex phenomenon exhibited by most tissues, including the lungs, whose molecular basis is still incompletely understood. Due to their visco-elastic properties, the lungs do not maintain constant stress under constant deformation, but slowly relax. The visco-elastic component of the inspiratory work of breathing contributes significantly to the overall pressure dissipation during spontaneous breathingCitation5,Citation6,Citation9,Citation10.
It has been previously shown that stress relaxation pressure dissipation is increased in inflamed lung tissueCitation13,Citation14, particularly as an effect of interleukin-6Citation9, and decreased as an effect of increased body temperatureCitation10. In contrast, the effect on lung stress relaxation of cysteinyl-leukotrienes, or of their antagonism, has never been measured before. Here it is shown that leukotrienes activity has no significant effect, at least in basal condition.
This result is different from what observed as a consequence of chronic steroid treatment, which has been shown to reduce stress relaxation in healthy miceCitation12. Thus, differently from steroid treatment, montelukast administration is not followed by a reduction of the visco-elastic component of the inspiratory work of breathing, in healthy animals.
Since Rmin,l also exhibits non-significant changes with montelukast, our results indicate that cysteinyl-leukotrienes exert their bronchoconstrictor effect in pathological but not in basal conditions, at least in mice. The above cited reports describing reduced bronchoconstriction and airway resistance as an effect of leukotrienes’ antagonism, in fact, regard spontaneous or experimental asthma, or leukotrienes-induced bronchoconstriction.
Thus, basal leukotrienes’ plasma concentration does not contribute to the modulation of airway calibre in basal condition in healthy mice. This finding is in agreement with previous results showing no effects of cysteinyl-leukotrienes’ antagonism on basal airway resistance in humansCitation38–40.
The inflation–deflation loops surface mean values here reported are very similar to those previously observed in analogous experimentsCitation12,Citation41,Citation42. Hence, in our experiment, the mice lung showed typical hysteretic behaviour.
Data have been reported in the literature describing an increment in phosphatidylcholine secretion from type II pneumocytes as an effect of cysteinyl-leukotrienesCitation15, and a reduction of surfactant synthesis induced by leukotrienes’ inhibitorsCitation16. However, direct measurements of their possible effects on the inflation–deflation loop surface have never been performed previously. Here it is shown that cysteinyl-leukotriene receptors’ antagonism by montelukast significantly increases lung hysteresis (), thus indirectly confirming a stimulating effect of leukotrienes on surfactant synthesis. Differently from what observed for other lung mechanics parameters, our data indicate that basal leukotrienes plasma concentration affects lung hysteresis. The same result has not been observed for steroid chronic treatmentCitation12.
This finding has practical consequences. In particular, the deflation limbs of the pressure–volume loops shift to the left in montelukast-treated compared to control mice indicates less static elastic pressure for a given lung volume. Thus, less lung elastic recoil pressure is available to drive the expiratory flow. It is suggested that this effect may counteract, at least in part, the improvement in expiratory flow permitted by the decrease in airway resistance in asthmatic subjects assuming montelukast.
According to Gillfillan et al.Citation15, the stimulating effect of leukotrienes on surfactant synthesis may be observed at a much lower concentration than basal concentration in healthy humansCitation2–4 and miceCitation43, and than nebuliser concentrations of inhaled leukotrienes causing bronchocontrictionCitation44. Thus, basal leukotrienes’ concentration may be effective in affecting surfactant activity but not bronchomotor tone and airway calibre.
Olfactory system histology
Healthy mice chronically treated with the steroid fluticasone propionate present an increase in the thickness of the olfactory mucosaCitation12. Moreover, their olfactory bulbs show slight but consistent differences in staining with Olfactory Marker Protein, and the diameter of their medial glomeruli was largerCitation12. Differently from what previously observed for chronic steroid treatment, leukotrienes receptors’ antagonism does not affect olfactory system histology, suggesting negligible effects on olfactory function.
Conclusions
Cysteinyl-leukotrienes’ antagonism does not change elastic or resistive lung properties, including the ohmic and visco-elastic pressure dissipations, in healthy mice in basal conditions. In addition, apparently it does not affect the olfactory system. These results indicate that basal lung mechanics and olfactory system histology are not affected by cysteinyl-leukotrienes’ activity in healthy mice.
In contrast, the observed lung hysteresis increment and the deflation pressure–volume curve shift to the left suggest that leukotriene antagonism may reduce the lung elastic recoil pressure available for expiratory flow drive in montelukast-treated asthmatic subjects.
Declaration of interest
The Authors have no conflicts of interest to declare.
References
- Drazen JM (1991). Regulation by leukotrienes. In: RG Crystal, TB West, eds. The Lung: Scientific Foundations. New York: Raven Press, 917–926.
- Masclans JR, Sabater J, Sacanell J, Chacon P, Sabin P, Roca O et al. Possible prognostic value of leukotriene B(4) in acute respiratory distress syndrome. Respir Care 2007;52:1695–1700.
- Ono E, Taniguchi M, Mita H, Fukutomi Y, Higashi N, Miyazaki E et al. Increased production of cysteinyl leukotrienes and prostaglandin D2 during human anaphylaxis. Clin Exp Allergy 2009;39:72–80.
- Qadri F, Raqib R, Ahmed F, Rahman T, Wenneras C, Das SK et al. Increased levels of inflammatory mediators in children and adults infected with Vibrio cholerae O1 and O139. Clin Diagn Lab Immunol 2002;9:221–229.
- Bates JH, Rossi A, Milic-Emili J. Analysis of the behavior of the respiratory system with constant inspiratory flow. J Appl Physiol 1985;58:1840–1848.
- Bates JH, Baconnier P, Milic-Emili J. A theoretical analysis of interrupter technique for measuring respiratory mechanics. J Appl Physiol 1988;64:2204–2214.
- D’Angelo E, Calderini E, Torri G, Robatto FM, Bono D, Milic-Emili J. Respiratory mechanics in anesthetized paralyzed humans: effects of flow, volume, and time. J Appl Physiol 1989;67:2556–2564.
- Reta GS, Riva JA, Piriz H, Medeiros AS, Rocco PR, Zin WA. Effects of halothane on respiratory mechanics and lung histopathology in normal rats. Br J Anaesth 2000;84:372–377.
- Rubini A. IL-6 increases airway resistance in the rat. Cytokine 2010;51:266–273.
- Rubini A. The effect of body warming on respiratory mechanics in rats. Respir Physiol Neurobiol 2011;175:255–260.
- Carvalho RS, de Pinho AG, de Andrade AP, Silva CA, Gaio CE, Tavares P. Analysis of pulmonary mechanics in an experimental model of sepsis. J Bras Pneumol 2006;32:316–321.
- Mucignat-Caretta C, Bondí M, Rubini A, Calabrese F, Barbato A. The olfactory system is affected by steroid aerosol treatment in mice. Am J Physiol Lung Cell Mol Physiol 2009;297:L1073–L1081.
- Silva PL, Passaro CP, Cagido VR, Bozza M, Dolhnikoff M, Negri EM et al. Impact of lung remodelling on respiratory mechanics in a model of severe allergic inflammation. Respir Physiol Neurobiol 2008;160:239–248.
- Xisto DG, Farias LL, Ferreira HC, Picanço MR, Amitrano D, Lapa E Silva JR et al. Lung parenchyma remodeling in a murine model of chronic allergic inflammation. Am J Respir Crit Care Med 2005;171:829–837.
- Gilfillan AM, Rooney SA. Leukotrienes stimulate phosphatidylcholine secretion in cultured type II pneumocytes. Biochim Biophys Acta 1986;876:22–27.
- Rooney SA, Gobran LI. Adenosine and leukotrienes have a regulatory role in lung surfactant secretion in the newborn rabbit. Biochim Biophys Acta 1988;960:98–106.
- Iwona S, Tomasz G. Antileukotriene treatment in children with asthma–new patents. Recent Pat Inflamm Allergy Drug Discov 2008;2:202–211.
- Warner JO. The role of leukotriene receptor antagonists in the treatment of chronic asthma in childhood. Allergy 2001;56 Suppl 66:22–29.
- Lammers CH, Schweitzer P, Facchinetti P, Arrang JM, Madamba SG, Siggins GR et al. Arachidonate 5-lipoxygenase and its activating protein: prominent hippocampal expression and role in somatostatin signaling. J Neurochem 1996;66:147–152.
- Shimizu T, Takusagawa Y, Izumi T, Ohishi N, Seyama Y. Enzymic synthesis of leukotriene B4 in guinea pig brain. J Neurochem 1987;48:1541–1546.
- Borish L. The role of leukotrienes in upper and lower airway inflammation and the implications for treatment. Ann Allergy Asthma Immunol 2002;88:16–22.
- Wilson AM, White PS, Gardiner Q, Nassif R, Lipworth BJ. Effects of leukotriene receptor antagonist therapy in patients with chronic rhinosinusitis in a real life rhinology clinic setting. Rhinology 2001;39:142–146.
- Gideroglu K, Yilmaz F, Aksoy F, Bugdayci G, Saglam I, Yimaz F. Montelukast protects axial pattern rat skin flaps against ischemia/reperfusion injury. J Surg Res 2009;157:181–186.
- Ozkan E, Akyüz C, Sehirli AO, Topaloglu U, Ercan F, Sener G. Montelukast, a selective cysteinyl leukotriene receptor 1 antagonist, reduces cerulein-induced pancreatic injury in rats. Pancreas 2010;39:1041–1046.
- Muz MH, Deveci F, Bulut Y, Ilhan N, Yekeler H, Turgut T. The effects of low dose leukotriene receptor antagonist therapy on airway remodeling and cysteinyl leukotriene expression in a mouse asthma model. Exp Mol Med 2006;38:109–118.
- Chang HK, Mortola JP. Fluid dynamic factors in tracheal pressure measurement. J Appl Physiol 1981;51:218–225.
- Bates JH, Hunter IW, Sly PD, Okubo S, Filiatrault S, Milic-Emili J. Effect of valve closure time on the determination of respiratory resistance by flow interruption. Med Biol Eng Comput 1987;25:136–140.
- Gong H Jr, Linn WS, Terrell SL, Anderson KR, Clark KW. Anti-inflammatory and lung function effects of montelukast in asthmatic volunteers exposed to sulfur dioxide. Chest 2001;119:402–408.
- Hele DJ, Birrell MA, Webber SE, Foster ML, Belvisi MG. Mediator involvement in antigen-induced bronchospasm and microvascular leakage in the airways of ovalbumin sensitized Brown Norway rats. Br J Pharmacol 2001;132:481–488.
- Ihaku D, Cameron L, Suzuki M, Molet S, Martin J, Hamid Q. Montelukast, a leukotriene receptor antagonist, inhibits the late airway response to antigen, airway eosinophilia, and IL-5-expressing cells in Brown Norway rats. J Allergy Clin Immunol 1999;104:1147–1154.
- Spahn JD, Covar RA, Jain N, Gleason M, Shimamoto R, Szefler SJ et al. Effect of montelukast on peripheral airflow obstruction in children with asthma. Ann Allergy Asthma Immunol 2006;96:541–549.
- Stelmach I, Grzelewski T, Bobrowska-Korzeniowska M, Stelmach P, Kuna P. A randomized, double-blind trial of the effect of anti-asthma treatment on lung function in children with asthma. Pulm Pharmacol Ther 2007;20:691–700.
- Harrison S, Gatti R, Baraldo S, Oliani KL, Andre E, Trevisani M et al. Montelukast inhibits inflammatory responses in small airways of the Guinea-pig. Pulm Pharmacol Ther 2008;21:317–323.
- Krell RD, Aharony D, Buckner CK, Keith RA, Kusner EJ, Snyder DW et al. The preclinical pharmacology of ICI 204,219. A peptide leukotriene antagonist. Am Rev Respir Dis 1990;141:978–987.
- Kanniess F, Richter K, Böhme S, Jörres RA, Magnussen H. Montelukast versus fluticasone: effects on lung function, airway responsiveness and inflammation in moderate asthma. Eur Respir J 2002;20:853–858.
- Nieto A, Pamies R, Oliver F, Medina A, Caballero L, Mazon A. Montelukast improves pulmonary function measured by impulse oscillometry in children with asthma (Mio study). Respir Med 2006;100:1180–1185.
- Muraki M, Imbe S, Sato R, Ikeda Y, Yamagata S, Iwanaga T et al. Inhaled montelukast inhibits cysteinyl-leukotriene-induced bronchoconstriction in ovalbumin-sensitized guinea-pigs: the potential as a new asthma medication. Int Immunopharmacol 2009;9:1337–1341.
- Smith LJ, Geller S, Ebright L, Glass M, Thyrum PT. Inhibition of leukotriene D4-induced bronchoconstriction in normal subjects by the oral LTD4 receptor antagonist ICI 204,219. Am Rev Respir Dis 1990;141:988–992.
- Barnes N, Piper PJ, Costello J. The effect of an oral leukotriene antagonist L-649,923 on histamine and leukotriene D4-induced bronchoconstriction in normal man. J Allergy Clin Immunol 1987;79:816–821.
- Israel E, Juniper EF, Callaghan JT, Mathur PN, Morris MM, Dowell AR et al. Effect of a leukotriene antagonist, LY171883, on cold air-induced bronchoconstriction in asthmatics. Am Rev Respir Dis 1989;140:1348–1353.
- Sly PD, Collins RA, Thamrin C, Turner DJ, Hantos Z. Volume dependence of airway and tissue impedances in mice. J Appl Physiol 2003;94:1460–1466.
- Wagers S, Lundblad L, Moriya HT, Bates JH, Irvin CG. Nonlinearity of respiratory mechanics during bronchoconstriction in mice with airway inflammation. J Appl Physiol 2002;92:1802–1807.
- Matsumoto J, Ishikawa S, Doi M, Kishida T, Ebihara K. Protease-resistant fraction of smoked, dried bonito alleviates atopic dermatitis-like skin lesions in NC/Nga mice. J Nutr Sci Vitaminol 2007;53:451–456.
- Drazen JM, Austen KF. Leukotrienes and airway responses. Am Rev Respir Dis 1987;136:985–998.