Abstract
Branched-chain amino acids (BCAAs) exhibit many physiological functions. However, the potential link and mechanism between BCAA and skin function are unknown. We examined the effects of deletion of branched-chain α-keto acid dehydrogenase kinase (BDK), a key enzyme in BCAA catabolism, on type I and III tropocollagen syntheses in mice. Leucine and isoleucine levels were significantly lower in the skin of BDK-KO mice compared with wild-type mice. No changes in valine concentrations were observed. The levels of type I and III tropocollagen proteins and mRNAs (COL1A1 and COL3A1) were significantly lower in the skin of BDK-KO mice compared with wild-type mice. The phosphorylation of p70 S6 kinase, which indicates mammalian target of rapamycin (mTOR) activation, was reduced in the skin of BDK-KO mice compared with wild-type mice. These findings suggest that deficiencies of leucine and isoleucine reduce type I and III tropocollagen syntheses in skin by suppressing the action of mTOR.
Graphic Abstract
The skin levels of type I and III tropocollagen proteins and COL1A1 and COL3A1 mRNAs were significantly lower in BDK-KO mice compared with WT mice.
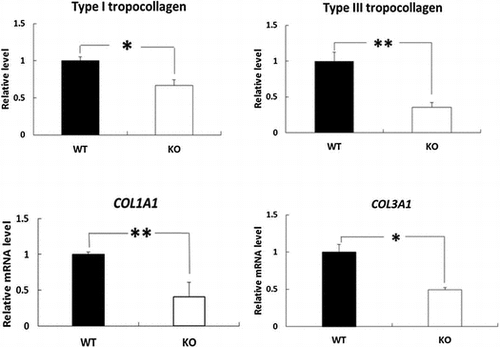
Keywords:
The skin contains large amounts of extracellular matrix components, such as collagen, which are produced mainly by fibroblasts and protect the body from various external insults. Type I and III collagen form the relatively broad extracellular fibers that are primarily responsible for the tensile strength of the skin. Type I collagen is the most abundant in normal skin, comprising more than 90% of its dry weight [Citation1]. Type III collagen, which is genetically distinct from type I collagen, represents approximately 10% of the total collagen in the adult human dermis [Citation2]. Type I collagen comprises two α1 and one α2 chains, and type III collagen contains three identical α1 chains [Citation3]. Previous studies showed that low levels of type I and III collagen correlated with the clinical severity of photoaged skin [Citation4,5].
Molecules involved in skin functionality are greatly affected by the levels of specific amino acids, which have been traditionally classified as nutritionally essential or nonessential for animals and humans. Essential amino acids (valine, leucine, isoleucine, threonine, methionine, phenylalanine, tryptophan, lysine, arginine, histidine, and proline) are not synthesized by animal cells and therefore need to be provided from the diet. In contrast, nonessential amino acids (glycine, alanine, serine, cysteine, tyrosine, asparagine, glutamate, glutamine, and aspartic acid) are synthesized de novo. Some researchers have shown that essential amino acids influence the homeostasis of collagen in the skin. Bellon et al. [Citation6] demonstrated that glutamine increases procollagen mRNA levels in human fibroblasts. Sazok et al. [Citation7] reported that exogenous proline stimulates type I collagen expression in human skin fibroblasts.
BCAA is abundant ingredients in the human diet accounting for nearly 20% of total protein intake [Citation8]. BCAA has important functions as nutrient signals promoting protein synthesis [Citation9] and as donors of nitrogen to Ala and Gln synthesis in various tissues [Citation10,11]. In patients with chronic liver disease, BCAA supplementation improved homeostasis model assessment of insulin resistance [Citation12] and decreased plasma glucose levels in glucose tolerance test [Citation13]. Because BCAA is essential amino acid, their homeostasis is regulated by catabolism. BCAA catabolism is potently regulated by mitochondrial branched-chain α-ketoacid dehydrogenase (BCKDH) complex (BCKDC). BCKDC activity is subject to regulation through phosphorylation (inactivation) and dephosphorylation (activation) by a specific kinase (BCKDH kinase: BDK) and a specific phosphatase (BCKDH phosphatase), respectively [Citation14]. A previous report has shown that plasma BCAA concentrations in mice with whole body BDK deficiency (BDK-KO mice) were decreased to 50% to control mice [Citation15].
Despite the evidence linking amino acids with collagen homeostasis, few studies have focused on the effects of BCAAs (leucine, isoleucine, and valine) on collagen synthesis in the skin. In the present study, we used BDK-KO mice to examine the potential link between BCAA with the production of type I and III collagen in the skin.
Materials and methods
Materials
Protease inhibitor cocktail was obtained from Sigma-Aldrich Corp. (St. Louis, MO, USA). PhosSTOP phosphatase inhibitor cocktail tablets were purchased from Roche Diagnostics Corp. (Indianapolis, IN, USA). RC DC protein assay was purchased from Bio-Rad Laboratories Inc. (Hercules, CA, USA). An anti-rat type I collagen polyclonal antibody and an anti-rat type III collagen polyclonal antibody were obtained from Rockland Immunochemicals, Inc. (Gilbertsville, PA, USA). Phospho-p70 S6K (Thr389) antibody and p70 S6K antibody were obtained from Cell Signaling Technology, Inc. (Beverly, MA, USA). ECL™ anti-rabbit IgG-horseradish peroxidase-linked whole antibody was obtained from Rockland Immunochemicals, Inc. (Gilbertsville, PA, USA). Hybond-ECL, Amersham Hybond-P PVDF membrane, and Amersham ECLTM Select Western Blotting Detection Reagents were obtained from GE Healthcare UK Ltd. (Little Chalfont, UK).
Generation of BDK-KO mice
The BDK-KO mice were generated as described previously [Citation16]. Animal procedures were approved by the Animal Care Committee of Nagoya University Graduate School of Bioagricultural Sciences and were performed in accordance with the relevant guidelines.
Measurement of skin BCAA concentrations
The skin samples were homogenized with an equal volume of 3% (w/v) sulfosalicylic acid and then centrifuged at 12,000×g at 4 °C for 15 min to remove precipitated proteins. The amino acid concentrations in the supernatants were analyzed using the JLC-500/V amino acid autoanalyzer (JEOL, Tokyo, Japan).
Immunoblot analysis
To extract neutral salt-soluble collagen (tropocollagen) from the dermal skin, the skin was homogenized in physiological saline at 0 °C, shaken for 20 h at 0 °C, and finally centrifuged as described by Niedermüller et al. [Citation17]. To the phosphorylation status of p70 S6K, tissue lysates were extracted from the skin prepared using radioimmunoprecipitation assay (RIPA) buffer supplemented with a protease inhibitor cocktail and a phosphatase inhibitor cocktail. The total amount of protein in the supernatant (tropocollagen) and the lysates was measured with an RC DC protein assay reagent. Equal amounts of protein were loaded and electrophoresed on a tris-glycine polyacrylamide gel in SDS and tris-glycine electrophoresis buffer according to the standard protocol. After the electrophoresis, proteins were transferred to a membrane by using a transfer buffer. The membrane was then blocked and incubated with anti-rat type I collagen polyclonal antibody (1:5000 dilution in 1% (w/v) nonfat dried milk) or phospho-p70 S6K (Thr389) polyclonal antibody (1:1000 dilution in Can Get Signal® Solution 1) at 4 °C overnight, washed 5 times, and incubated with anti-rabbit IgG-horseradish peroxidase (1:2500 dilution in the blocking buffer) for 1 h. After washing the membranes, the immunoreactive bands were visualized using Amersham™ ECL™ Select Western Blotting Detection Reagents. Reprobing was performed with 1:5000 of anti-rat type III collagen polyclonal antibody or 1:1000 of p70 S6K antibody. The amount of tropocollagen in the skin was assessed by analyzing the intensity of the collagen α-chain band that corresponds to a single-chain component, and the phosphorylation status of p70 S6K was quantified by using Amersham Imager 600 (GE Healthcare UK Ltd., Little Chalfont, UK). The protein level for BDK-KO mice was expressed as a relative value to that of WT mice.
RNA extraction and quantitative PCR
Total RNA was extracted from the skin using ISOGEN (Nippon Gene Co., Ltd., Tokyo, Japan), according to the manufacturer’s instructions. Reverse transcription was performed using a High Capacity cDNA Reverse Transcription Kit under the following conditions: 37 °C for 2 h and 85 °C for 5 min after preheating at 25 °C for 10 min. The amplification products obtained were detected using the THUNDERBIRD™ SYBER® qPCR Mix (Toyobo Co., Osaka, Japan) and StepOnePlus Real-Time PCR System (Applied Biosystems, Foster City, CA, USA). Amplifications were performed under the following conditions: 38 cycles of 95 °C for 5 s and 60 °C for 30 s after preheating at 95 °C for 30 s, 95 °C for 15 s, and 60 °C for 1 min. The relative expression levels of each target gene were calculated using the comparative Ct method using glyceraldehyde-3-phosphate dehydrogenase (GAPDH) as an internal control. The primer sequences were as follows: GAPDH forward, 5′-CGACTTCAACAGCAACTCCCACTCTTCC-3′ and GAPDH reverse, 5′-TGGGTGGTCCAGGGTTTCTTACTCCTT-3′; COL1A1 forward, 5′-TGCCTCAGAAGAACTGGTACATCA-3′ and COL1A1 reverse, 5′-ATCGGTCATGCTCTCTCCAAA-3′; COL3A1 forward, 5′-TGAAACCCCAGCAAAACAAAA-3′ and COL3A1 reverse, 5′-TTGGTCACTTGCACTGGTTGAT-3′
Statistical analysis
Results are expressed as mean ± SE. Statistically significant differences between the two groups were determined using the Student’s t-test. *p < 0.05 and **p < 0.01 indicate values that are significantly different from WT mice.
Results
Body weight
No significant differences in body weight were observed in BDK-KO mice when compared with WT mice (Figure ).
Effects of deletion of BDK on BCAA concentrations in the skin
We first confirmed the concentrations of BCAAs in the skin of BDK-KO mice and WT mice using the JLC-500/V amino acid autoanalyzer (Figure ). Consistent with the previous study, the results showed that levels of leucine and isoleucine were significantly lower in the skin of BDK-KO mice compared with WT mice. No significant differences in valine concentrations were observed.
Effects of deletion of BDK on the skin levels of type I and III tropocollagen and COL1A1 and COL3A1 mRNAs
We next investigated the levels of type I and III tropocollagen, which polymerizes to microfibrillar collagen to initiate aggregation, in BDK-KO mice compared with WT mice using immunoblot analysis. The results showed that both type I and III tropocollagen were significantly reduced in the skin of BDK-KO mice compared with WT mice (Figure (a) and (b)). We also measured the mRNA levels of the genes involved in synthesizing type I and III collagen in the skin of BDK-KO mice. Quantitative PCR analysis revealed that both COL1A1 and COL3A1 mRNA levels were also significantly lower in the skin of BDK-KO mice than that in WT mice (Figure (c) and (d)).
Figure 3. Effects of deletion of BDK on the levels of type I and type III tropocollagen proteins and COL1A1 and COL3A1 mRNAs in the skin. Type I tropocollagen (a) and type III tropocollagen (b) in the skin were evaluated by Western blotting and quantified. Density was expressed as a fold change relative to levels detected in WT mice. The mRNA levels of COL1A1 (c) and COL3A1 (d) in the rat skin were measured by quantitative PCR, and the values are expressed as the value relative to that of GAPDH. Data are expressed as mean ± SE (n = 4). *p < 0.05 and **p < 0.01 compared with WT mice.
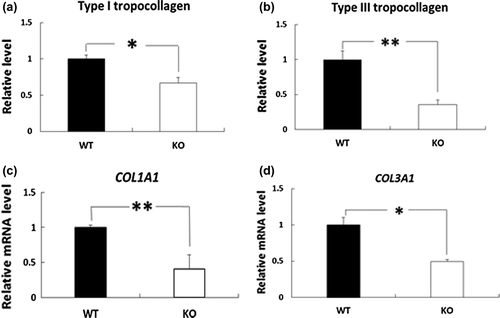
Effects of deletion of BDK on the phosphorylation status of p70 S6K in the skin
To clarify the mechanism underlying the reduction in COL1A1 and COL3A1 gene expression in the skin of BDK-KO mice, we next evaluated mammalian target of rapamycin complex (mTOR) activity. A previous study showed that BCAAs signal to mTOR, which activates 70 kDa ribosomal protein S6 kinase (S6K1) to promote mRNA translation [Citation18]. Thus, we next performed immunoblot analysis to measure the phosphorylation status of p70 S6K in BDK-KO mice compared with WT mice (Figure ). Compared with WT mice, the phosphorylation levels of p70 S6K were significantly reduced in the skin of BDK-KO mice.
Discussion
Collagen synthesis in the skin is greatly affected by essential amino acids [Citation6,7]. Several studies have shown that BCAAs, which are components of proteins, can also function in cellular signals [Citation9]. However, the potential influence of BCAA on skin function remains unclear.
In the present study, we examined the changes in the synthesis of type I and III tropocollagens, which are related to skin function, in the skin of BDK-KO mice. BDK-KO mice, in which the BCKDC is almost fully activated in all tissues, showed significantly low concentrations of plasma BCAA [Citation15]. BCAA concentrations in brain, kidney, heart, and muscle are reduced by approximately 50% in BDK-KO mice compared with WT mice [Citation19]. Our results were consistent with these studies, showing that levels of leucine and isoleucine were significantly lower in the skin of BDK-KO mice compared with WT mice. No changes were observed in valine levels, suggesting that the regulation of BCAA catabolism in the skin may be different from that in other tissues, although the detailed mechanism remained to be clarified. We observed by RT-PCR analysis that the BDK genes were expressed in mice skin (data not shown). Therefore, we propose that BDK is a potent regulator of key enzymes that are involved in controlling leucine and isoleucine levels in the skin. The relationship between deletion of BDK and valine concentrations in the skin is unclear.
Our results showed that the skin levels of type I and III tropocollagen proteins and COL1A1 and COL3A1 mRNAs were reduced in BDK-KO mice compared with WT mice. These results indicate that the downregulation of type I and III collagen syntheses, possibly due to the reduction in leucine and isoleucine, probably occurs at the transcriptional level. To clarify the mechanism underlying the reduction in mRNAs levels of COL1A1 and COL3A1 in BDK-KO mice, we assessed mTOR activity. mTOR is a serine–threonine protein kinase that controls translation initiation and is responsive to cellular levels of free amino acids [Citation20]. BCAAs are physiologically active amino acids, because as they are not only essential amino acids, but also signal to mTOR, which in turn activates S6K1 to promote mRNA translation [Citation18]. This mTOR pathway is activated by BCAAs, with leucine being the most effective [Citation21]. Poulalhon et al. [Citation22] showed that mTOR inhibition by rapamycin leads to downregulation of COL1A1 and COL3A1 gene expression in fibroblasts. Our results show that the phosphorylation of p70 S6K was reduced in the skin of BDK-KO mice. Taken together, this suggests that one reason for the reduction in syntheses of type I and III tropocollagen may be the suppression of BCAA-dependent activation of mTOR/p70 S6K in the skin in BDK-KO mice.
In summary, here, we showed that deletion of BDK results in reduction in the syntheses of type I and III tropocollagen in skin by suppressing the action of mTOR. Several researchers have demonstrated that amino acids have multiple effects on skin functions. Murakami et al. [Citation23] reported that the combination of BCAA and Gln is a key factor for the enhancement of skin collagen synthesis in protein-malnourished rats. Our previous study showed that Ile, Leu, and Val deficiency resulted in a pronounced decrease in hyaluron production in dermal fibroblasts [Citation24]. We believe that our findings help establish a new concept of functional BCAAs that can regulate metabolic pathways to improve skin health.
Author contributions
T. Y. conceived and designed the experiments. M. S. performed the experiments. T. Y. and M. S. wrote the manuscript. M. S. analyzed the data. K. I. and Y. O. contributed reagents/materials/analysis tools.
Disclosure statement
No potential conflict of interest was reported by the authors.
References
- Nigel PB. Inherited diseases of collagen, elastin and keratin. Medicine. 2005;33:83–86.
- Epstein EH Jr. (Alpha1(3))3 human skin collagen. Release by pepsin digestion and preponderance in fetal life. J Biol Chem. 1974;249:3225–3231.
- Baumann L, Kaufman J, Saghari S. Collagen fillers. Dermatol Ther. 2006;19:134–140.10.1111/dth.2006.19.issue-3
- Griffiths CE, Russman AN, Majmudar G, et al. Restoration of collagen formation in photodamaged human skin by tretinoin (retinoic acid). N Engl J Med. 1993;329:530–535.10.1056/NEJM199308193290803
- Talwar HS, Griffiths CE, Fisher GJ, et al. Reduced Type I and Type III procollagens in photodamaged adult human skin. J Invest Dermatol. 1995;105:285–290.10.1111/1523-1747.ep12318471
- Bellon G, Chaqour B, Wegrowski Y, et al. Glutamine increases collagen gene transcription in cultured human fibroblasts. Biochim Biophys Acta. 1995;1268:311–323.10.1016/0167-4889(95)00093-8
- Szoka L, Karna E, Hlebowicz-Sarat K, et al. Exogenous proline stimulates type I collagen and HIF-1α expression and the process is attenuated by glutamine in human skin fibroblasts. Mol Cell Biochem. Forthcoming.
- Harris RA, Joshi M, Jeoung NH, et al. Overview of the molecular and biochemical basis of branched-chain amino acid catabolism. J Nutr. 2005;135:1527S–1530S.
- Kimball SR, Jefferson LS. Signaling pathways and molecular mechanisms through which branched-chain amino acids mediate translational control of protein synthesis. J Nutr. 2006;136:227S–231S.
- Garber AJ, Karl IE, Kipnis DM. Alanine and glutamine synthesis and release from skeletal muscle. II. The precursor role of amino acids in alanine and glutamine synthesis. J Biol Chem. 1976;251:836–843.
- Garber AJ, Karl IE, Kipnis DM. Alanine and glutamine synthesis and release from skeletal muscle. I. Glycolysis and amino acid release. J Biol Chem. 1976;251:826–835.
- Kawaguchi T, Nagao Y, Matsuoka H, et al. Branched-chain amino acid-enriched supplementation improves insulin resistance in patients with chronic liver disease. Int J Mol Med. 2008;22:105–112.
- Korenaga K, Korenaga M, Uchida K, et al. Effects of a late evening snack combined with alpha-glucosidase inhibitor on liver cirrhosis. Hepatol Res. 2008;38:1087–1097.10.1111/hep.2008.38.issue-11
- Shimomura Y, Obayashi M, Murakami T, et al. Regulation of branched-chain amino acid catabolism: nutritional and hormonal regulation of activity and expression of the branched-chain alpha-keto acid dehydrogenase kinase. Curr Opin Clin Nutr Metab Care. 2001;4:419–423.10.1097/00075197-200109000-00013
- Joshi MA, Jeoung NH, Obayashi M, et al. Impaired growth and nurological abnormalities in branched-chain α-ketoacid dehydrogenase kinase-deficient mice. Biochem J. 2006;400:153–162.10.1042/BJ20060869
- Ishikawa T, Kitaura Y, Kadota Y, et al. Muscle-specific deletion of BDK amplifies loss of myofibrillar protein during protein undernutrition. Sci Rep. 2017;7:39825.10.1038/srep39825
- Niedermüller H, Skalicky M, Hofecker G, et al. Investigations on the kinetics of collagen-metabolism in young and old rats. Exp Gerontol. 1977;12:159–168.10.1016/0531-5565(77)90001-8
- Proud CG. Regulation of mammalian translation factors by nutrients. Eur J Biochem. 2002;269:5338–5349.10.1046/j.1432-1033.2002.03292.x
- Watford M. Lowered concentrations of branched-chain amino acids result in impaired growth and neurological problems: insights from a branched-chain alpha-keto acid dehydrogenase complex kinase-deficient mouse model. Nutr Rev. 2007;65:167–172.10.1301/nr.2007.apr.167-172
- Kimball SR, Jefferson LS. Control of translation initiation through integration of signals generated by hormones, nutrients, and exercise. J Biol Chem. 2010;285:29027–29032.10.1074/jbc.R110.137208
- Fumarola C, La Monica S, Guidotti GG. Amino acid signaling through the mammalian target of rapamycin (mTOR) pathway: role of glutamine and of cell shrinkage. J Cell Physiol. 2005;204:155–165.10.1002/(ISSN)1097-4652
- Poulalhon N, Farge D, Roos N, et al. Modulation of collagen and MMP-1 gene expression in fibroblasts by the immunosuppressive drug rapamycin A direct role as an antifibrotic agent? J Biol Chem. 2006;281:33045–33052.10.1074/jbc.M606366200
- Murakami H, Shimbo K, Takino Y, et al. Combination of BCAAs and glutamine enhances dermal collagen protein synthesis in protein-malnourished rats. Amino Acids. 2013;44:969–976.10.1007/s00726-012-1426-4
- Yamane T, Inoue Y, Kobayashi-Hattori K, et al. Effects of an amino acid deficiency on hyaluronan synthesis in human dermal fibroblasts. Food Sci Technol Res. 2016;19:979–982.