ABSTRACT
Women are under-represented in science fields; this poses a significant challenge considering the emerging digital revolution. The intersectionality theory suggests that women whose social identities intersect across gender and class have distinct experiences; affecting their science aspirations. This research examined gender and class intersections, measuring science experiences and aspirations of working-class girls versus those who are not. A total of 421 female students (ages 15–18) from Irish secondary schools answered questions about their science experiences and views. The findings revealed that working-class girls displayed lower levels of confidence and interest in pursuing science; additionally, they felt that their ability in science was lower than other students. These findings provide highlight the impact of gender and class on science aspirations, showing the value of considering intersectionality when addressing unequal participation in science. Science interventions should consider the intersectional layers of disadvantage which can affect young women's science aspirations.
Introduction
Gender inequity is pervasive across science education and employment fields; less than one-third of engineering and one-fifth of computer science undergraduates are female, and at age fifteen only 4.7% of females compared to 18% of males show interest in science, even amongst the highest-grade pupils (OECD, Pisa, Citation2014). And in the world of professional research, the gender gap is clear and startling, in the one hundred and twenty years of the Nobel Prize, only 23 women have received the award in Chemistry, Medicine or Physics compared with 599 men (Lunnemann et al., Citation2019). While women comprise the largest underrepresented group in all science fields, we see further stratification along multiple social axes, including class, race and ability (Wang & Degol, Citation2017). As it currently stands the dominant group is the one which most closely aligns with the historical norm of a scientist–the white, middle-class men. This is an important social justice issue, the insufficient number of students graduating from science is a concern for the future supply of skilled workforce and it has the potential to impact the economic competitiveness of countries and the social mobility of families. Understanding the science experiences and aspirations of women who are under-represented in science is an essential step in ensuring gender equality in science participation.
Science decision-making
The umbrella term used to refer to the progressive loss of women in various science areas science over time has long been referred to as the ‘Leaky Pipeline’ (Alper, Citation1993). According to research conducted by Dasgupta and Stout, girls’ interest in science and related science fields shifts at many stages from early childhood to adulthood (Dasgupta & Stout, Citation2014). The stereotypes associated with gender in science are a factor which contributes to the gender inequality seen in the field. From the age of two children understand that sex differences exist and the stereotypes that are attached to them, and children form preconceptions of their own gender and the norms associated with it (Blakemore, Citation2003). Girls and boys assign themselves roles that are reflected by family, friends, and their culture; scientists’ mathematicians and engineers are almost always men (Chambers, Citation1983). Furthermore, negative stereotypes associated with girls’ intellectual abilities are observed in children as young as six years old. When asked to identify the gender of someone who is ‘Really, really smart,’ girls are more to answer ‘boys’ than their own gender (Bian et al., Citation2017).
By early adolescence interest in science-related areas science is established, suggesting that interventions are needed across childhood to grow the interest in science (Maltese & Tai, Citation2010). It can be argued that the first step in fostering this early interest is to create environments that pique the situational interests of young girls. Using a variety of learning experiences both informal and formal allows the seeds of personal interest to grow (Haden, Citation2010). The combined efforts of the immediate learning environment, such as discussions with parents, visits to exhibitions and extracurricular activities provide children with opportunities to explore science and technology in a way that holds child’s attention and interests (Pinkard et al., Citation2017). Girls often have little to no exposure to female role models in science before entering third-level education, and even then, if they do progress to science courses female faculty members are less than 25% making it hard to see themselves in professions (Harford, Citation2018).
Another factor that influences the number of girls displaying an interest in science is the lack of opportunities to experience science and technology in school (Master et al., Citation2017). Schools can be short-staffed or under-resourced, in some cases they do not offer even the three basic sciences at the secondary school level, further inhibiting the chance of any meaningful connection being made for students to the sciences. This shortage of staff along with a lack of academic preparation is clearly seen in the number of children who take science subjects in Second Level education. In Ireland, we see that over 72% of boys take a science subject in their final year compared to just over 39% of girls (Department of Education and Skills, Citation2019) (excluding biology and maths). One study found students in same-sex schools, 91% of boys had access to physics classes as compared with 84% to applied maths (55% versus 38%), showing that the gender divide in science is influenced by school subject (Delaney & Devereux, Citation2019).
Gender and class
When considering how young people from diverse socio-economic backgrounds make decisions about the courses or careers that they will take there are a myriad of influences. Theories of social and cultural capital state that who you know and what you know implicitly within your family impact the type of careers and courses you will aspire to (O'Sullivan et al., Citation2019). Children from ‘middle-class’ backgrounds are more likely to develop and sustain science aspirations which reflect their family practices, values and science capital (Archer et al., Citation2015) science capital is the material and cultural science-related resources that families have access to. Research has shown that children from higher social classes have more access to more science capital in the form of science-related qualifications and contacts (Archer et al., Citation2012). Research conducted with 11-year-old children by (Archer et al., Citation2013) observed that working-class girls had different science aspirations than middle-class girls which were related specifically to the capital within their families- and the girls who did speak about having science aspirations tended to be the middle-class girls who had ‘undertaken considerable identity “work” to reconcile their science aspirations with acceptable discourses of femininity’ (Archer et al., Citation2013, p. 179).
While we have begun to understand the personal and structural barriers that stop women from progressing in science courses and careers, developing interventions that challenge socialisation processes and encourage women to see science an option for them, we must also consider the implicit assumption that underpins such research- that all women are the same. Research on diversity within diversity in science participation is a new field (Chaudhary & Dutt, Citation2022) and there is limited research thus far which has comprehensively compared the science experiences and aspirations of girls from different socio-economic backgrounds. Gender barriers are undoubtedly significant when considered alone, but social identities, like gender and class, can and do intersect with gender identities to produce different experiences (Rosette & Tost, Citation2010). All our social identities have their own stereotypes which impact science participation, these identities make it easier for some people to identify with science roles than others. As it currently stands the dominant normative associations with science include being rational, clever, and objective, we use words like ‘geek’ and ‘boffin’ to describe scientists, and a man in a white jacket, square-rimmed glasses, in a lab being the normative stereotype. We associate these words, and science itself, with the expression of masculinity (Makarova et al., Citation2019; Mendick & Francis, Citation2012). These associations can make it difficult to identify with the scientistscience for those who perform feminine social roles; science and its related fields science are not ‘girly’ ‘pretty’ or ‘glamourous’ enough to fit with the roles that society prescribes to those who identify as female (Moote et al., Citation2020). The challenges of seeing themselves in science rolesscience are further increased for girls from working-class communities. Labels like ‘geek’ which is commonly associated with those who are ‘good’ in school, and good at science, contribute to the undesirability of these science subjects for girls who are from communities where being ‘good’ at school is less valued. These layers of intersectional values and beliefs contribute to working-class girls being the least likely to participate in science courses and careers (Codiroli McMaster & Cook, Citation2019). Thus far, researchers in this area have reduced the issue of inequalities in science participation science to a single factor (gender) and have not fully facilitated the understanding of distinct experiences from inextricably linked social identities (Grabe, Citation2020). As such, we have begun to understand the factors that influence ALL women's decision to participate in science but the intersection between gender and social class is under-explored. As a result, our current understanding of this subject is largely constrained and may marginalise social groups which intersect across gender and class.
Theoretical frame
Intersectionality is a theoretical and empirical paradigm (Hancock, Citation2007) which rejects the ‘single-axis framework’ put forward by feminist scholars. It states that an over-focus on one social identity category pushes more vulnerable groups, especially those who inhabit dual social identities, to the margins (Crenshaw, Citation1989). The single category approach seeks to explain the inequality from the dominant groups’ perspective; in the case of science participation, it will often identify the gender problem, and develop solutions which fit women as if they are all the same, leaving the overall sciences of socialisation unchanged- and failing to consider the experiences of diverse groups of women. The intersectional approach challenges the idea of a universal female voice which speaks for all women. That voice often speaks for just a subset of women- the most advantaged- which can hide or conflate intragroup differences (Crenshaw, Citation1991). In setting some characteristics of a particular group as fixed and certain, the members of that group who are different in other aspects of their identity (e.g. class) can be marginalised or made to feel like they are invisible (Hancock, Citation2007). Even where differences are acknowledged, the implications of this can sometimes be lost, or referenced only in passing rather than identifying how very real differences can be made invisible (Symington, Citation2004). Intersectionality provides an alternative framework to consider how the different social identities attributed to class and gender impact upon.
In the current study we use intersectionality as the frame with which to consider the science experiences and aspirations of girls in secondary schools across the Republic of Ireland. Like other countries, Ireland has a legacy of structural disadvantage. This is particularly evident in education; schools that are in disadvantaged areas struggle with low levels of numeracy and literacy, and there is a higher concentration of children who have less informed educational and employment aspirations (Smyth et al., Citation2015). These schools are operating in a very competitive education environment where more advantaged, middle-class families, have social, cultural, and economic resources to support their children to succeed (O’Sullivan et al., Citation2019). Poverty and inequality have increased in recent years and considerable attention has been paid to the huge increase in child poverty as well as the decrease in the upward mobility of certain groups in Irish society. This has resulted in educational initiatives which have attempted to address educational disadvantage; several national initiatives have been implemented in recent years to promote equality across education. The DEIS (Delivering Equality of Opportunity in Schools) is the most significant national initiative that targets socio-economically disadvantaged communities.
It was introduced to primary and secondary schools in Ireland in 2005, based on the premise that socio-economic disadvantage is spatially concentrated. Schools located in disadvantaged areas receive ring-fenced funding to support education engagement. In 2021 183,000 pupils were attending 896 DEIS programme schools across the country including 700 primary schools and 198 post-primary schools. The process through which a school is identified and categorised as a DEIS school is carried out using an identification model using Department of Education enrolment data and data from the most recent census as represented by the Pobal HP Deprivation Index (Nelis et al., Citation2021). The model has been modified in recent years to account for a broader definition of disadvantage and accounts for the severity of disadvantage through a new assessment process. Within such defined schools, principles report that on average 60% or 3 in 5 children come from a disadvantaged background, compared with 20% in non-DEIS schools (Nelis et al., Citation2021). The use of DEIS schools as a measure of disadvantage also helps to reflect the impact of socioeconomic factors on educational outcomes (Banerjee, Citation2016). Such disadvantages for students include lower mean scores of family wealth measures, being less likely to have a parent who has attended third-level education and higher rates of unemployment among parents of DEIS students (Nelis et al., Citation2021).
The aim of the current study is to explore the science experiences and aspirations of girls who are of a lower socio-economic class and those that are not versus girls who are not. In the context of this study, we have defined working-class girls as those who are attending a post-primary school which has DEIS status. For a school to be categorised as DEIS they require evidence that they have historically experienced educational problems (e.g. below-average percentage of student’s retention rates) and had above average percentage enrolment of students from poorer backgrounds (e.g. larger percentages of families in receipt of welfare supports, or living in areas that high concentration of socio-economic disadvantage). The use of DEIS as a proxy for a social class has been supported by research, the mean score for parental occupational status is significantly lower in DEIS schools compared to non-DEIS schools, and more non-DEIS students have a parent who has a university degree over half compared to one-third in DEIS schools- both indicators of lower social class (Gilleece et al., Citation2020). We use the assumption that many girls in DEIS schools are from a lower socio-economic class and that those in other schools are other classes.
To examine how class and gender intersect to impact science participation we have taken a broad approach to understanding. We have included questions about science career and college aspirations, science perceptions and beliefs and science networks and experiences, to explore if there are differences between girls from working-class communities and girls from those not. The research provides insight into intersectionality and predicts that girls from lower-socio-economic backgrounds with have less access to science resources and information and that their perceptions will be different to those of girls from other backgrounds.
Materials and methods
Data
The data used in this study form part of a large-scale longitudinal study entitled the STEM Passport for Inclusion, a longitudinal study following 1000 young women from Ireland over 3 years. The data used in this study were the year one data (collected in 2021). The STEM Passport for Inclusion recruited schools from across Munster and Leinster, by sending email invitations to a list of all secondary schools taken from a national database of schools. The email invited schools to volunteer a group of students to participate in the STEM Passport for Inclusion programme of research and engagement, 55 schools expressed interest and 28 schools volunteered to participate. Fifteen schools were Deis schools and 13 were non-Deis. In each school a lead teacher selected students to participate in the study science. No guidance was offered on who should participate or the number of students. The STEM Passport collects data at three time points: September 2021, May 2022 and September 2022. The data used for this study were the September 2021 survey data collected from students at the time of recruitment and involved an online survey design using the platform Qualtrics.
Participants
All participants in this study were female students attending an Irish secondary school in September 2021. They were in the senior cycle of the Irish education system, aged between 15 and 18. The senior cycle comprises of 4th year, 5th year and the final year of secondary school 6th year. Information sheets were sent to the lead teacher in each school, and they were distributed to students and their parents/guardians before participating in the survey. Students were provided with consent forms which were emailed to the school, these were signed by both student and guardian and returned via the school. Four hundred and twenty-one senior cycle girls (age 16–18 years), from 28 schools, completed the survey with 45.4% (n = 191) being from non-DEIS schools and 54.6% (n = 230) being from DEIS schools. Participants were provided no incentives to participate; if a student did not wish to participate in the survey it did not affect their participation in the STEM Passport for Inclusion.
Measures
The survey used was created through an online platform called Qualtrics which students had access to through an emailed link to them. It included an information sheet; a consent and debriefing form and the survey questions themselves. Demographic questions including school subjects studied were gathered from participants. Students’ confidence and interest in science courses and careers were also explored. The scales used in this study include the science Career Interests questionnaire, the Motivational Belief Constructs Scale, Assessments of self and task perceptions in the domain of science and an adapted gender stereotype scale.
Science definition
At the start of the survey, we defined STEM as ‘education and employment opportunities, experiences or courses which relate to four core areas-Science Technology Engineering and Math (science)’. We asked students to respond to the term STEM in the survey as a general reference to science. While we recognise that the generality of the umbrella term ‘STEM’ as a synonym for such a diverse group of skills and academic fields poses various problems, we felt that this definition would ensure the students thought beyond the main science subjects on offer in school (biology, physics and chemistry) including technology engineering and math. For consistency, we have used STEM as the umbrella term which students respond to in the survey.
Demographic information
Demographic information included questions about subjects taken followed by questions surrounding science beliefs and experiences. Questions about students’ knowledge of science courses and their networks were included, these questions were categorical and included the following questions; ‘I know what science is’, ‘I want to know more about science careers’, ‘I want to know more about science courses’, ‘I know a woman or women from my own community who work in Science Technology Engineering or Math?’, ‘I have a family member who works in science’, ‘I know a woman in science’, ‘I have visited a science job’, ‘I know what a science job looks like’, ‘Have attended a computer science course’, ‘Have basic computing skills’, ‘Have attended a coding course’, ‘One of my parents went to university’, these questions were scored as a yes or no response.
Confidence and interest in science technology engineering or math courses and careers
Four questions were asked about science studying and career interest and confidence, they included ‘I am interested in studying science in future’, ‘I am interested in working in science in future’, ‘I am confident I can study science in future’, ‘I am confident I can work in science in future,’ – these were scored using a 5-point Likert scale from strongly disagree (1) to strongly agree (5).
Career interest questionnaire (CIQ)
The CIQ is a Likert-designed survey which is a 9-item measure scored 1 = strongly disagree to 5 = strongly agree. It was adapted from a longer scale developed to help promote science engagement in students (Bowdich, Citation2009). The CIQ measures students’ opinions on having a science career in the future and measuring external factors such as parental encouragement in science Interests. Some examples of questions given include ‘I would like to have a career in science’ and ‘My family is interested in the science subjects I study’ This scale allowed researchers to evaluate a student's current science career beliefs and intentions.
Motivational belief constructs
The motivational belief scale uses three subscales consisting of 20 items to measure students’ motivation towards science studies (Wang & Liou, Citation2017). The subscales of Self-Concept consist of nine items: Intrinsic Values consisting of five questions and Utility Values consisting of six questions were used and three perspectives were given to understand students’ science beliefs.
The self-concept subscale consists of measures of a student's expectancy beliefs and how they view their own abilities in science. For example, ‘I usually do well in science Technology Engineering and Maths subjects’. The intrinsic value subscale measures a student's engagement with science based on their enjoyment of the subject. For example, the question ‘I enjoy learning about science Technology Engineering and Maths’. Finally, the utility value subscale measures the usefulness of science in terms of a student's future goals/intentions. For example, the question ‘I think science Technology Engineering and Maths will help me in my daily life’. Students rated their level of agreement to statements on a 5-point Likert scale from 1 = Strongly Disagree to 5 = Strongly agree. Reverse coding was utilised for questions 2, 3, 5, and 9 on the self-concept sub-scale and questions 2 and 3 on the intrinsic value subscale. This was to ensure that a higher number would indicate greater self-concept, intrinsic and utility beliefs.
Perceived task value items
The perceived task value items have been adapted from a study by Eccles and Wigfield (Citation1995). The scale was used to measure the value an individual places on their science studies through their intrinsic interest values (their enjoyment of the subject), the importance the subjects have towards their future intentions and their extrinsic utility values (how well they believe they perform in comparison with their peers). The 7-item scale gives an overall perspective of how an individual values their science studies, and the motivating factors behind this perceived value. Questions asked include, ‘In general I find working on science assignments … ’. Students rated their agreement or disagreement with a statement on a 5-point Likert scale which was coded to indicate increasing perceived values of their science subjects. Responses varied depending on the question, for example in the previous example question provided students were provided options from Very Boring (Scored as 1) to Very Interesting (Scored as 5).
Ability expectancy-related items
The ability expectancy scale is a 5-question scale which was adapted from Eccles and Wigfield (Citation1995) and used to measure how students expect to perform in their science subjects and how they compare themselves to their peers in their science studies. Questions include, ‘Compared to other students how well do you expect to do in your science subjects this year?’. Responses provided vary depending on the question, for example, in the previous example question the responses would range from Much Worse (coded as 1) to Much Better (coded as 5). The answers were coded accordingly so that a higher score overall would indicate that a student was more confident in their abilities and expected to do well in the subject in future.
Perceived task difficulty Items
These items were adapted from a study by Eccles and Wigfield (Citation1995) to allow researchers to investigate science perceptions. The 7-item scale was used to measure an individual's perception of science subjects in relation to their task difficulty and the effort required to complete the task. Questions include ‘In general, how hard are science subjects for you?’. Students were provided with multiple choice answers on a 5-point Likert scale and the responses provided varied depending on the question, for example, the previous example was scored Very Hard-1 to Very Easy-5. This allowed researchers to associate increasing scores with students finding science subjects as less difficult and as having more self-confidence in their abilities compared with their peers.
Gender stereotypes
The gender stereotype items were adapted from a maths scale (Li, Citation2007). Three items were used and scored on a five-point Likert scale according to how much a student agrees with the statement, for example, ‘science is more important for boys,’ 1-Strongly Agree to 5-Strongly Disagree. This allowed researchers to measure students’ internalised stereotypes of science education/careers. A higher score overall would indicate that a student does not agree with stereotypical statements.
Procedure
This study received ethical approval from the Maynooth Universities Social Research Ethics Sub Committee (2,424,733). The STEM Passport for Inclusion is an initiative which provides the opportunity for science engagement, training and mentoring for young girls in school. Schools are recruited through email invitation and students are invited to participate through information sheets provided to the lead teacher from each school. They are informed of the programme’s focus, aims and length as well as the purpose of the research survey. Participating teachers are informed of what participation would require on their behalf, including ensuring the distribution and collection of consent forms from students and parents/guardians, and their return to the coordinating researcher. Teachers will be reminded that participation in the STEM Passport for Inclusion project is not dependent on participating in the research and this will also be made clear to the students.
All students were provided with information sheets and consent forms which illustrated what exactly the students would be partaking in, their privacy and how their responses would be used. In addition, parental consent forms and information sheets were also distributed, to participate both student and legal guardian were informed and provided consent for participation. Anonymity was ensured for both student and guardian and participants were reminded of their ability to withdraw from participation at any point. With the possibility of students becoming uncomfortable or upset while evaluating their perceived science abilities and confidence, students were reminded that their responses were not a reflection of their abilities. Once consent was provided students began the study by filling out the survey once before participation and again once the programme concluded, students were also debriefed at each stage.
The STEM Passport for Inclusion uses an Industry-Education approach to science equity; providing underserved girls with meaningful opportunities to develop science Capital- that can be used to progress to science courses and careers. There are three elements of the STEM Passport for Inclusion project in which students took part in:
Science: Prepared: Students participated in a science skills programme delivered by Microsoft and Accenture to provide skills in coding, AI, and computer science. Its focus was on developing twenty-first-century skills necessary for science jobs. In partnership with Maynooth University and Munster Technological University the science Passport skill modules will be accredited by the universities, ensuring students who complete the programme will receive a university qualification (level 6 NFQ, 5 credits) they can use alongside their CAO to access science courses. This is the first award of its kind in Ireland, giving a 50-point Leaving Cert Bonus in Munster Technological University for STEM Passport graduates.
Students also participated in 6 group mentoring sessions and 3 full-day DreamSpace activities in RDI Hub Kerry, and Microsoft Headquarters, Dublin. The science mentors are made up of a team of working professional women from Microsoft Ireland and Accenture staff. The students receive one mentoring class every science Skills Module lab day, to support the girls in viewing science as more accessible. Mentoring allows for the opportunity to uncover new skills and exposes mentees to socially desirable or high-achieving peer groups with whom they can identify.
Finally, the STEM Passport platform brings together all the science programmes on offer across Ireland, housing information about all activities in every county and region. It will include a repository of information about what is on offer in every town and city in Ireland, classifying these activities in terms of content and quality. The platform also houses information about who has participated in the STEM-Preparation activities, coded and used to create an SPSS file for quantitative analysis. Students’ identities were protected by creating a unique ID number systemscience; additionally, the data were stored on a password-protected computer.
Data analysis
The quantitative survey responses obtained from Qualtrics were exported through and appropriately recorded and organised. Once this data were cleaned it was exported to IBM SPSS Statistics Version 26, where the responses could be processed, and analysed. Before conducting the analysis an a priori sample size calculation was conducted to determine the sample size needed for sufficient statistical power. The minimum sample size based on an a-priori, assuming an anticipated Cohen’s d of .3 desired Statistical Power Level of .8 and Probability Level of .05, was 352 participants.
Preliminary analysis of the data identified the frequencies for relevant categorical variables. Total values for continuous variables were calculated and descriptive statistics were analysed. These frequencies were also investigated as a split file, by separating DEIS and non-DEIS students’ researchers could compare average scores between groups. Independent sample t-tests were used to understand the differences among groups on various continuous variables.
Results
Analysis
In total there were 429 responses submitted to the online survey sent out, 8 responses were removed from the dataset for analysis as they submitted an empty survey with no responses past the consent form or had completed the survey twice (n = 8). Leaving n = 421 valid responses for analysis. A preliminary descriptive analysis found no significant differences in the number of participants who completed the relevant scales.
Demographics
The frequency statistics of the relevant categorical variables are listed in . The sample consisted of female secondary school students, 191 from a non-DEIS secondary school and 230 from a DEIS secondary school.
Table 1. Frequency statistics of categorical variables.
Science subjects
includes the percentage of students studying science subjects for the Leaving Certificate. This shows that non-DEIS students are taking more science subjects than DEIS students- although the percentage difference was small.
Table 2. Percentage of students across schools studying leaving certificate science subject.
Science knowledge and networks
A descriptive comparison of the percentage of ‘yes’ responses to questions about students’ science knowledge and science networks. All students were interested in learning more about science courses and careers, and there were no large differences between DEIS and Non-DEIS students in knowing a woman in science areas, or having a family member in the science field . Low girls across both groups have visited a science job ().
Table 3. Percentage of students across schools with science knowledge & networks.
Independent T-Tests
To identify the differences between DEIS and non-DEIS students on science attitudes, interests, and confidence a series of independent t-tests were conducted.
Interest in studying and working in science
To understand science interests independent sample t-tests were undertaken on these scales. illustrates student responses to this question-DEIS students reported significantly less interest in studying science in the future as compared to Non-DEIS students. DEIS students also reported significantly less interest in working in science in the future than Non-DEIS students ().
Figure 1. Differences in DEIS and non-DEIS students’ science study and career interest and confidence.
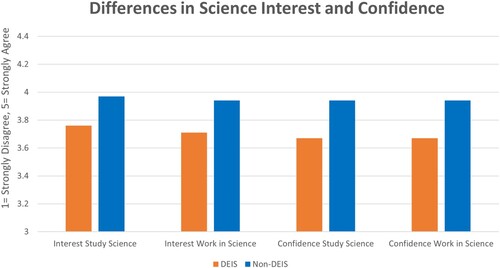
Table 4. Differences in DEIS and non-DEIS students’ science study and career confidence and interest.
Science utility beliefs
To understand science Utility Beliefs, which are students’ views on the usefulness of science in terms of a student's future goals/intentions, independent sample t-tests were undertaken on the survey scales. shows that DEIS students reported significantly lower science utility beliefs than Non-DEIS students ().
Table 5. Differences in DEIS and non-DEIS students’ science beliefs, expectations and perceptions.
Science ability expectations
To understand science Ability Expectations, which is how students perceive their own science Abilities, independent sample t-tests were undertaken on the average survey scales. shows that DEIS students reported more negative perceptions of their science abilities than Non-DEIS students ().
Science perceived task difficulty
To understand science Perceived Task Difficulty, which is how difficult a student finds science compared to other subjects and how much effort they must put into doing well in science, independent sample t-tests were undertaken on the average survey scales. shows that DEIS students reported less science perceived task difficulty than Non-DEIS students ().
Career interest scale
To understand differences in the science career interest scale independent sample t-tests on the items in the scale were undertaken. reports the only scales which had significant differences; the results show that DEIS students would enjoy a career in science less than non-DEIS students, the DEIS students reported lower agreement with the question about their family interest in their science courses than the non-DEIS students, they also said they were less likely to like a have a career in science than the non-DEIS students ().
Table 6. Differences in DEIS and Non-DEIS students’ science career interest scales.
Discussion
This research demonstrates that science beliefs, perceived abilities, interest and confidence to engage in science courses and careers are influenced by gender and social class. The research acknowledges that individuals who inhabit multiple social identities (i.e. both the identity of being female and working class) have intersectional layers of disadvantage which impact can impact their education and employment aspirations and outcomes. Using DEIS school status as a proxy for working-class students the research demonstrates differences in female students’ science beliefs, perceptions, interests and confidence across social class lines.
Science experiences and exposure
Demographic analysis of student responses demonstrated that there were no class-based differences in the science subjects being taken in school or in girls’ interest to learn science. Nearly all students, irrespective of social class, wanted to know more about science. This shows that young women want to learn about and explore science- regardless of whether they choose to pursue science in future education. Acknowledging this interest to learn and harnessing this curiosity may be key to increasing the rate of young women becoming involved in science. The number of students who know a woman in science was low (55%) which may indicate that young women lack access to female role models in science. There were no class differences observed which suggests this may just be a gender issue, rather than a social class insight, research has shown that access to female role models in science can create opportunities for girls to feel they belong (Bertrand and Duflo, Citation2017). Our research suggests that only half of the young women in the current study knew a woman in science, which may have a negative effect on their science aspirations. Only 24% of students overall had visited a science job (20% DEIS, 28% Non-DEIS). Research has shown that having first-hand experience in fields such as industry and technology can change student’s perceptions and stereotypes of such science careers (Eidelman et al., Citation2011). Being able to sit with professionals, ask questions and experience the environment of the industry opens students to the possibility of continuing science education in future.
Interest and confidence in science
The study examined students’ confidence and interest in studying and working in science in future- results show that students from DEIS schools rated their confidence and interest in science significantly lower than students from non-DEIS schools. science education research has noted various factors which overlap to create ‘science capital’, one being socio-economic disadvantage. Students from working-class, or lower-class communities, have less access to ‘science capital’ which is defined as a person's exposure to and knowledge of science careers, their involvement in out-of-school science activities, and their perception of the value and utility of science (Archer et al., Citation2015). These findings further emphasise the influence of ‘science capital’ on a child's science aspirations; however, there is still an opportunity to even the odds for all students. By providing the opportunity to learn about science, providing mentors for young women and creating a supportive environment students can become more confident in their abilities and increase their interest in science.
Career interest scale
The Career Interests Questionnaire was utilised to understand the students’ attitudes towards and factors impacting their pursual of a science career in future. Students from DEIS schools reported lower perceived enjoyment of science and decreased intentions of pursuing a science career. Previous research has found that students' enjoyment of studying science is positively correlated with scientific achievement (Wang et al., Citation2021). If students don’t find a subject interesting or engaging this can negatively influence their career opinions and aspirations. From early school ages, girls can identify science stereotypes and begin to view science as a male-oriented field, impeding their future career aspirations (Boston and Cimpian, Citation2018). For young women from lower socioeconomic backgrounds, communication surrounding the utility of science subjects is often lacking- additionally, they are much less likely to be encouraged to persist in their science studies than boys (Bystydzienski, Citation2009). DEIS students expressed that their family was less interested in their science studies than non-DEIS students. These findings support previous research which notes that student achievement is impacted by parental educational involvement (Rowan-Keynon et al., Citation2010). Previous research has found that children of more highly educated parents take more mathematics and science-based subjects at secondary school (Harackiewicz et al., Citation2012). Acknowledging such factors around the effect of parental interest in education provides further insight for researchers and policy-makers.
Usefulness
While measuring students’ motivations for studying science, DEIS students had lower utility belief scores than non-DEIS students- meaning DEIS students felt that studying science was less useful for their future intentions. This may overlap with the lack of enjoyment and parental involvement noted previously. For students who already experience stereotypes connected to science and are given less encouragement to pursue a career in the field, it is not unexpected they would not value a career in science. This is a key observation as utility scores are a predictor of science career aspirations- more so than other motivational belief constructs (Watt et al., Citation2012). The marked differences between groups indicate an intersectional influence on students’ beliefs of science. Students may aware of the stereotypes linked to science careers- the masculine and cold associations which exist may leave students pulling away from the field before they get the opportunity to learn more. Furthermore, the intersection of the class creates a more intricate situation for researchers to interpret. When considering how individuals from diverse social backgrounds make decisions about future careers, a myriad of influences must first be considered. Continued research into such factors will help future policy-makers and researchers to support students in viewing science education as an option which will open doors in their futures.
Perceived science Ability/Difficulty
Finally, the girls were asked to rate how they perceived their own abilities in science- DEIS students reported their science abilities as lower, and difficulties as higher in science than non-DEIS students. Results such as this are reflective of a student’s experiences and internalised beliefs surrounding science. Current predominant beliefs surrounding science still maintain stereotypes of highly intelligent men experiencing high levels of stress in their careers. Such high expectations of themselves and a negative view of the field can lead young women to move away from science (Aschbacher et al., Citation2013). Previous studies have shown that while female students typically had higher math and verbal scores on tests, they rated their ability and confidence as lower than male classmates (Wang et al., Citation2013). However, less research has been conducted to investigate the influence of class on women pursuing science. These findings highlight the need for further research into the influence of social class on perceived science abilities.
Conclusion
This research demonstrates the importance of intersectionality as an approach to understanding young women’s experiences of science education in Ireland. These findings have highlighted how students experiencing intersectional layers of disadvantage perceive and experience science education differently from their peers. Such research highlights the need for tailored intervention strategies for young women who inhabit multiple social identities. By employing intersectionality as a theoretical framework this research encourages future researchers to consider the nuanced experiences and perspectives of young women from varying social classes. Considering such experiences allows researchers to aid young women with the appropriate resources to explore science education and potentially support their journey into science as a career. An important consideration for the results is how and what students perceive science to be. In this research we introduce science as the overarching term for subjects, courses and jobs which relate to science technology engineering and math; however, there are challenges with simplifying complex concepts insofar as it may mean that students assign different meanings and interpretations to the overall term (Marginson et al., Citation2013). Future research should consider establishing the components within science that are distinct and asking intersectional groups to share their interpretation or meaning of science. Despite this challenge, the current research demonstrates that there are intersectional factors which impact young women's science experiences and that social class is something that should be considered in the development of broad science-related gender interventions.
Ethics statement
This research received ethical approval from the Social Research Ethics Sub-Committee at Maynooth University (247075)
Disclosure statement
No potential conflict of interest was reported by the author(s).
Data availability statement
The data that support the findings of this study are available on request from the corresponding author. The data are not publicly available due to privacy or ethical restrictions.
Additional information
Funding
References
- Alper, J. (1993). The pipeline is leaking women all the way along. Science, 260(5106), 409–411. https://doi.org/10.1126/science.260.5106.409
- Archer, L., Dawson, E., DeWitt, J., Seakins, A., & Wong, B. (2015). “Science capital”: A conceptual, methodological, and empirical argument for extending bourdieusian notions of capital beyond the arts. Journal of Research in Science Teaching, 52(7), 922–948. https://doi.org/10.1002/tea.21227
- Archer, L., DeWitt, J., Osborne, J., Dillon, J., Willis, B., & Wong, B. (2012). Science aspirations, capital, and family habitus. American Educational Research Journal, 49(5), 881–908. https://doi.org/10.3102/0002831211433290
- Archer, L., DeWitt, J., Osborne, J., Dillon, J., Willis, B., & Wong, B. (2013). ‘Not girly, not sexy, not glamorous’: Primary school girls’ and parents’ constructions of science aspirations. Pedagogy, Culture & Society, 21(1), 171–194. https://doi.org/10.1080/14681366.2012.748676
- Aschbacher, P. R., Ing, M., & Tsai, S. M. (2013). Boosting student interest in science. Phi Delta Kappan, 95(2), 47–51. https://doi.org/10.1177/003172171309500211
- Banerjee, P. A. (2016). A systematic review of factors linked to poor academic performance of disadvantaged students in science and maths in schools. Cogent Education, 3(1), 1178441. https://doi.org/10.1080/2331186X.2016.1178441
- Bertrand, M., & Duflo, E. (2017). Handbook of economic field experiments. Handbook of Economic Field Experiments, 1, 309–393. https://doi.org/10.1016/bs.hefe.2016.08.004
- Bian, L., Leslie, S. J., & Cimpian, A. (2017). Gender stereotypes about intellectual ability emerge early and influence children’s interests. Science, 355(6323), 389–391. https://doi.org/10.1126/science.aah6524
- Blakemore, J. E. O. (2003). Children's beliefs about violating gender norms: Boys shouldn't look like girls, and girls shouldn't act like boys. Sex Roles, 48(9), 411–419. https://doi.org/10.1023/A:1023574427720
- Boston, J. S., & Cimpian, A. (2018). How do we encourage gifted girls to pursue and succeed in science and engineering? Gifted Child Today, 41(4), 196–207. https://doi.org/10.1177/1076217518786955
- Bowdich, S. (2009, February). Analysis of research exploring culturally responsive curricula in Hawaii. In Hawaii Educational Research Association Annual Conference (Vol. 7).
- Bystydzienski, J. M. (2009). Why so few women? Explaining gendered occupational outcomes in science, technology, engineering and mathematics fields. Sex Roles, 60(9-10), 751–753. https://doi.org/10.1007/s11199-008-9548-6
- Chambers, D. W. (1983). Stereotypic images of the scientist: The draw-a-scientist test. Science Education, 67(2), 255–265. https://doi.org/10.1002/sce.3730670213
- Chaudhary, N., & Dutt, A. (2022). Women as agents of change: Exploring women leaders’ resistance and shaping of gender ideologies in Pakistan. Frontiers in Psychology, 13, 800334–800334. https://doi.org/10.3389/fpsyg.2022.800334
- Codiroli McMaster, N., & Cook, R. (2019). The contribution of intersectionality to quantitative research into educational inequalities. Review of Education, 7(2), 271–292. https://doi.org/10.1002/rev3.3116
- Crenshaw, K. (1989). Demarginalizing the intersection of race and sex: A black feminist critique of antidiscrimination doctrine, feminist theory and antiracist politics. University of Chicago Legal Forum, 1989(1), 139–167.
- Crenshaw, K. (1991). Mapping the margins: Intersectionality, identity politics, and violence against women of color. Stanford Law Review, 43, 1241–1299. http://dx.doi.org/10.2307/1229039.
- Dasgupta, N., & Stout, J. G. (2014). Girls and women in science, technology, engineering, and mathematics. Policy Insights from the Behavioral and Brain Sciences, 1(1), 21–29. https://doi.org/10.1177/2372732214549471
- Delaney, J. M., & Devereux, P. J. (2019). Understanding gender differences in STEM: Evidence from college applications✰. Economics of Education Review, 72, 219–238. https://doi.org/10.1016/j.econedurev.2019.06.002
- Department of Education and Skills. (2019). A report on science, technology, engineering and mathematics (STEM) education. The STEM Education Review Group, November 2016, Ireland.
- Eccles, J. S., & Wigfield, A. (1995). In the mind of the actor: The structure of adolescents’ achievement task values and expectancy-related beliefs. Personality and Social Psychology Bulletin, 21(3), 215–225. https://doi.org/10.1177/0146167295213003
- Eidelman, L., Hazzan, O., Lapidot, T., Matias, Y., Raijman, D., & Segalov, M. (2011). Mind the (gender) gap. ACM Inroads, 2(3), 64–70. https://doi.org/10.1145/2003616.2003637
- Gilleece, L., Nelis, S. M., Fitzgerald, C., & Cosgrove, J. (2020). Reading, mathematics and science achievement in DEIS schools: Evidence from PISA 2018. Educational Research Centre.
- Grabe, S. (2020). Research methods in the study of intersectionality in psychology: Examples informed by a decade of collaborative work with majority world women’s grassroots activism. Frontiers in Psychology, 11, 494309. https://doi.org/10.3389/fpsyg.2020.494309
- Haden, C. A. (2010). Talking about science in museums. Child Development Perspectives, 4(1), 62–67.
- Hancock, A. M. (2007). When multiplication doesn't equal quick addition: Examining intersectionality as a research paradigm. Perspectives on Politics, 5(1), 63–79. https://doi.org/10.1017/S1537592707070065
- Harackiewicz, J. M., Rozek, C. S., Hulleman, C. S., & Hyde, J. S. (2012). Helping parents to motivate adolescents in mathematics and science. Psychological Science, 23(8), 899–906. https://doi.org/10.1177/0956797611435530
- Harford, J. (2018). The perspectives of women professors on the professoriate: A missing piece in the narrative on gender equality in the university. Education Sciences, 8(2), 50. https://doi.org/10.3390/educsci8020050
- Li, Q. (2007). Mathematics, science, and technology in secondary schools: Do gender and region make a difference? Canadian Journal of Learning and Technology, 33(1), 44–57. https://doi.org/10.21432/T2N018.
- Lunnemann, P., Jensen, M. H., & Jauffred, L. (2019). Gender bias in Nobel prizes. Palgrave Communications, 5(1), 1–4. https://doi.org/10.1057/s41599-019-0256-3
- Makarova, E., Aeschlimann, B., & Herzog, W. (2019). The gender gap in STEM fields: The impact of the gender stereotype of math and science on secondary students’ career aspirations. In Frontiers in Education (Vol. 4, p. 60). Frontiers Media SA.
- Maltese, A. V., & Tai, R. H. (2010). Eyeballs in the fridge: Sources of early interest in science. International Journal of Science Education, 32(5), 669–685. https://doi.org/10.1080/09500690902792385
- Marginson, S., Tytler, R., Freeman, B., & Roberts, K. (2013). SCIENCE: Country comparisons: Final report. Australian Council of Learned Academies.
- Master, A., Cheryan, S., Moscatelli, A., & Meltzoff, A. N. (2017). Programming experience promotes higher STEM motivation among first-grade girls. Journal of Experimental Child Psychology, 160, 92–106. https://doi.org/10.1016/j.jecp.2017.03.013
- Mendick, H., & Francis, B. (2012). Boffin and geek identities: Abject or privileged? Gender and Education, 24(1), 15–24. https://doi.org/10.1080/09540253.2011.564575
- Moote, J., Archer, L., DeWitt, J., & MacLeod, E. (2020). Science capital or STEM capital? Exploring relationships between science capital and technology, engineering, and maths aspirations and attitudes among young people aged 17/18. Journal of Research in Science Teaching, 57(8), 1228–1249. https://doi.org/10.1002/tea.21628
- Nelis, S. M., Gilleece, L., Fitzgerald, C., & Cosgrove, J. (2021). Beyond achievement: Home, school and wellbeing findings from PISA 2018 for students in DEIS and non-DEIS schools. Educational Research Centre.
- OECD, Pisa. (2014). Results: What students know and can do-student performance in mathematics reading, and science (Vol. 1, revised edition). OECD Publishing.
- O'Sullivan, K., Bird, N., Robson, J., & Winters, N. (2019). Academic identity, confidence and belonging: The role of contextualised admissions and foundation years in higher education. British Educational Research Journal, 45(3), 554–575. https://doi.org/10.1002/berj.3513
- Pinkard, N., Erete, S., Martin, C. K., & McKinney de Royston, M. (2017). Digital youth divas: Exploring narrative-driven curriculum to spark middle school girls’ interest in computational activities. Journal of the Learning Sciences, 26(3), 477–516. https://doi.org/10.1080/10508406.2017.1307199
- Rosette, A. S., & Tost, L. P. (2010). Agentic women and communal leadership: How role prescriptions confer advantage to top women leaders. Journal of Applied Psychology, 95(2), 221–235. https://doi.org/10.1037/a0018204
- Rowan-Kenyon, H. T., Swan, A. K., Deutsch, N. L., & Gansneder, B. (2010). Academic success for working adult students. In Understanding the working college student: New research and its implications for policy and practice (pp. 93–112). Stylus.
- Smyth, E., McCoy, S., & Kingston, G. (2015). Learning from the evaluation of DEIS. Economic and Social Research Institute.
- Symington, A. (2004). Intersectionality: A tool for gender and economic justice. Women’s Rights and Economic Change, 9, 1–8.
- Wang, C. L., & Liou, P. Y. (2017). Students’ motivational beliefs in science learning, school motivational contexts, and science achievement in Taiwan. International Journal of Science Education, 39(7), 898–917. https://doi.org/10.1080/09500693.2017.1310410
- Wang, H. H., Lin, H. S., Chen, Y. C., Pan, Y. T., & Hong, Z. R. (2021). Modelling relationships among students’ inquiry-related learning activities, enjoyment of learning, and their intended choice of a future STEM career. International Journal of Science Education, 43(1), 157–178. https://doi.org/10.1080/09500693.2020.1860266
- Wang, M. T., & Degol, J. L. (2017). Gender gap in science, technology, engineering, and mathematics (STEM): Current knowledge, implications for practice, policy, and future directions. Educational Psychology Review, 29(1), 119–140. https://doi.org/10.1007/s10648-015-9355-x
- Wang, M. T., Eccles, J. S., & Kenny, S. (2013). Not lack of ability but more choice. Psychological Science, 24(5), 770–775. https://doi.org/10.1177/0956797612458937
- Watt, H. M., Shapka, J. D., Morris, Z. A., Durik, A. M., Keating, D. P., & Eccles, J. S. (2012). Gendered motivational processes affecting high school mathematics participation, educational aspirations, and career plans: A comparison of samples from Australia, Canada, and the United States. Developmental Psychology, 48(6), 1594–1611. https://doi.org/10.1037/a0027838