ABSTRACT
Taraxasterol (TAL) is a pentacyclic triterpenoid compound, which has anti-inflammatory effect. Cytotoxicity assay was used to determine the optimal concentration of TAL and positive control dipotassium glycyrrhizinate (DG), and the optimal dose of UVB. Experimental data indicate that TAL has scavenging activity against UVB radiation-induced intracellular reactive oxygen species (ROS) compared to UVB controls. The contents of skin barrier-related factors in the groups were detected by Enzyme-linked immunosorbent assay (ELISA), then ELISA and quantitative polymerase chain reaction (qPCR) were used to detect changes in the inflammatory factors, apoptosis factors, and gene levels in the groups. Therefore, TAL stabilised the levels of inflammation, apoptosis, and skin barrier-related factors by regulating Mitogen-activated protein kinases/nuclear factor-k-gene binding (MAPK/NF-κB) signalling pathways, such as jun-amino-terminal kinase (JNK), p38, extracellular signal-regulated kinase (ERK), and NF-κB. These results suggest that TAL repairs UVB-induced skin barrier damage by scavenging reactive oxygen species and regulating the MAPK/NF-κB signalling pathway.
Introduction
The skin is the body's first barrier. The epidermis is the outermost layer of the skin, and keratinocytes are the main constituent cells of the epidermis. Keratinocytes can produce keratinocyte growth factors during differentiation, which can significantly promote the protection and repair of tissue damage (Finch & Rubin, Citation2004; J. Park et al., Citation2020). The skin barrier prevents the entry of harmful substances such as antigens and infectious microorganisms and maintains the body's water balance (Lodén, Citation2003; Yosipovitch et al., Citation2019). When external irritants disrupt the skin barrier, the moisture content in the skin is affected, leading to various skin inflammatory diseases such as atopic dermatitis (AD) and psoriasis in severe cases. Skin inflammation in turn weakens the barrier (Agrawal & Woodfolk, Citation2014; Segre, Citation2006; Vickery, Citation2007).
Aquaporin 3 (AQP3) and FLG are vital markers of skin hydration. AQP3 is a water–glycerol channel protein which can control the flow of water in and out of cells and has a close relationship with cell aging (Tonghui et al., Citation2002) (Hara et al., Citation2002). FLG is involved in the synthesis of natural moisturising factors and promotes epidermal differentiation (S. H. Park et al., Citation2021). KLK7 can hydrolyse desmosome protein, a key factor in skin desquamation, and is involved in the induction of inflammation (Igawa et al., Citation2017). Studies have found that EGF can enhance the vitality and delay the aging of epidermal cells. In addition, it can stimulate the synthesis and secretion of certain extracellular macromolecules (such as hyaluronic acid, collagen, etc.), and moisturise the skin, and is the source of skin vitality and health (Kong et al., Citation1987; Thönes et al., Citation2019).
Ultraviolet B radiation (UVB) is a band of ultraviolet wavelengths at 280–320 nm, which is divided according to the different biological effects. It can cause the sunburn and redness of the skin in a short time and damage the skin barrier function (Diffey, Citation2002; C. Zhang et al., Citation2020). UVB rays stimulate epidermal cells to produce reactive oxygen species (ROS), and ROS generated by UV irradiation is the leading cause of skin inflammation and apoptosis (Piao et al., Citation2012; Rafferty et al., Citation2003).
As shown in . As one of the active components in the whole dandelion plant, taraxasterol (TAL) has anti-inflammatory, anti-tumor, and other effects (Che et al., Citation2019; Chen et al., Citation2020). Studies have shown that TAL has obvious anti-inflammatory effects in vivo in animal models (Wang et al., Citation2016). It not only inhibits LPS-induced inflammatory responses by activating the LXR α-ABCA1 signalling pathway (Liu et al., Citation2018), but has a protective effect on rheumatoid arthritis (Jiang et al., Citation2016). The anti-inflammatory efficacy of TAL is well established. However, little research has been done on the reparative effects of TAL on UVB-induced skin barrier damage.
In this study, we explored the relevant mechanisms of TAL in repairing UVB-induced skin barrier damage by evaluating the cytotoxicity, changes in related cytokines and ROS, and the MAPK/NF-κB signalling pathway .
Experimental
Materials
Taraxasterol and dipotassium glycyrrhizinate: Shanghai Yuanye Biotechnology Co., Ltd.; HaCaT cells: China Institute of Inspection and Quarantine; trypsin-EDTA Solution, phosphate-buffered saline (PBS), fetal bovine serum (FBS) and penicillin–streptomycin solution: Beyotime Biotechnology; BCA Protein Quantification Kit and TNF-a, IL-6, IL-8, IL-1β, ROS, etc. ELISA kits: Biorigin; UVB lightbox: Shenzhen Guanhongrui Technology Co., Ltd.; cell incubator: Shanghai Yiheng Technology Co., Ltd.; 6-well plates and 96-well plates: Corning Inc.; PCR instruments: Applied Biosystems, Inc.
Method
Cell culture
HaCaT cells were grown in DMEM containing 10% FBS and 1% penicillin–streptomycin in flasks. The cells were kept in a constant temperature incubator at 37°C with 5% CO2 for 2–3 days.
Cytotoxicity analysis
The HaCaT cells were counted and added to 96-well culture plates at a density of 1.0 × 104/well, then incubated in a cell incubator for 12 h. The medium was discarded, and the cells were treated with different concentrations of TAL for 12 h, and cytotoxicity tests were conducted according to the instructions of the CCK8 Kit. The same as above, PBS was added to each well after discarding the medium, and the cells were irradiated with different doses of UVB, the PBS was aspirated, the medium was added and cultured for 12 h, and cytotoxicity tests were conducted according to the instructions of the CCK8 Kit. The same again, PBS was added to each well after discarding the medium, and the cells were irradiated with different doses of UVB, the PBS was aspirated, and the cells were treated with TAL at a mass concentration of 15.6 μg/mL for 12 h. The blank group did not undergo any treatment, and the model group was only irradiated with UVB but the samples were not loaded.
Oxidative stress analysis
The cell culture was the same as the above steps. After the culture was complete, the cells were washed with PBS (pH 7.4, 0.01 mol/L), 0.25% trypsin was added to digest the cells from the 6-well plate, and the cell pellets were collected by centrifugation. According to the instructions of the ROS Detection Kit, the fluorescence was detected at an excitation wavelength of 488 nm and emission wavelength of 525 nm, and the fluorescence intensity was recorded.
Enzyme-linked immunosorbent assay (ElISA)
The cell culture was the same as above. After washing the cells with PBS, 100 μL of lysate was added to each well to lyse the cells and obtain cell lysate. It was kept at 4°C and centrifuged at 1.2 × 104 g for 5 min; then the pellets were discarded to obtain the supernatant of the cell lysate. After the cell lysate and supernatant were obtained, the effects of TAL on the content of related factors in HaCaT cells were detected according to the kit.
Quantitative polymerase chain reaction (qPCR) analysis
The HaCaT cells were counted and seeded in 6-well culture plates at a density of 1.0 × 106/well. They were incubated in a cell incubator for 12 h, then the medium was discarded, PBS was added to each well, the cells were irradiated with UVB, the PBS was aspirated, and the cells were treated with TAL at a mass concentration of 15.6 μg/mL for 24 h. RNA was extracted using the Trizol method and stored in a −80°C refrigerator. The cDNA first-strand synthesis reaction was performed using a reverse transcription kit. The primer sequences are shown in :
Table 1. Primer sequences.
Results
Cell viability
The cytotoxicity results determined by the CCK8 assay are shown in . It can be seen in (a) that increasing the concentration of TAL enhances the toxicity of the cells. When the concentration is greater than 15.63 ug/mL, the cell viability decreases to below 80%. (b) shows that HaCaT cells viability were reduced by UVB at different radiation doses. The higher the radiation dose, the lower the cell viability. The UVB model was obtained when the cell survival rate was 50%, and the irradiation intensity reached 19.18 mJ/cm2. After induction, cell viability was significantly reduced, as shown in (c). After irradiation with 19.18 mJ/cm2 UVB, the cells were treated with TAL (3.9, 7.8, 15.6 μg/mL). The results show that cell viability recovered better when the concentration was 3.9 ug/mL. In subsequent experiments, 3.9 ug/mL was selected as the final concentration.
TAL repairs UVB-induced oxidative stress
The generation of intracellular ROS was detected using a DCFH-DA fluorescent probe. As shown in , the blank group has a small amount of fluorescence and low ROS content. After UVB irradiation, the intracellular active oxygen content significantly increases, and the green fluorescence enhancement is shown in the microscope image. The addition of TAL and the positive control DG treatment show that the green fluorescence is significantly weakened in the microscope image, proving that TAL has obvious ROS scavenging effects.
TAL can reduce the content of inflammatory factors induced by UVB
TNF-a, IL-6, IL-8, and IL-1β are significant inflammatory markers. As shown in , the protein levels and gene expressions of the four inflammatory factors were detected using the qPCR and ELISA methods. After the UVB irradiation of the HaCaT cells, the protein and gene expressions of the four inflammatory factors in the cells significantly increased. Compared with the model group, after adding TAL and positive control DG, the expressions of inflammatory factors in the cells were reduced. Compared with the positive control group, TAL and the positive control DG significantly reduced inflammatory factors. Our results show that the contents of TNF-a, IL-6, IL-8, and IL-1β all increased in HaCaT cells after UVB irradiation. Moreover, TAL can reduce inflammatory factors in HaCaT cells.
Figure 4. Regulation of inflammatory factor content in UVB-irradiated cells by TAL: (a, b) Changes in protein and gene levels of TNF-α; (c, d) Changes in protein and gene levels of IL-6; (e, f) Changes in protein and gene levels of IL-1β; (g, h) Changes in protein and gene levels of IL-8. (C: Control group; M: UVB irradiation model group).
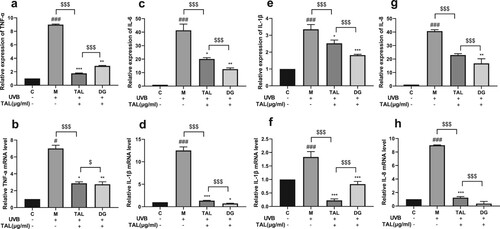
TAL can regulate the content of skin barrier-related factors induced by UVB
KLK7, AQP3, FLG, and EGF are key factors in maintaining a healthy skin barrier. The expression levels of skin barrier-related factor genes in HaCaT cells were detected using qPCR. After UVB irradiation, the relative expression of KLK7 in the cells significantly increased, and the relative expressions of AQP3, FLG, and EGF significantly decreased. The relative expression of KLK7 in the cells was significantly reduced when the damaged cells were treated with TAL and DG, and the relative expression levels of AQP3, FLG, and EGF significantly increased. Compared with the positive control group, TAL and DG can significantly regulate the content of skin barrier-related factors and bring them to normal levels .
TAL can regulate the content of UVB-induced apoptotic factors
The proportional relationship between Bax and Bcl-2 is a key factor in the strength of the inhibitory effect on apoptosis. The qPCR and ELISA methods were used to detect the protein level and gene expression of apoptotic factors in HaCaT cells. After HaCaT cells were irradiated with UVB, the protein and gene expressions of apoptotic factors (capase-3, capase-9) in the cells significantly increased, and the ratio of Bax/Bcl-2 increased. Compared with the model group, after adding TAL and positive control DG, the expression of apoptotic factors in the cells decreased, and the ratio of Bax/Bcl-2 decreased. Compared with the positive control group, TAL and the positive control DG significantly reduced the content of apoptotic factors. Our results show that both the content of apoptotic factors and the Bax/Bcl-2 ratio increased in HaCaT cells after UVB irradiation. Moreover, TAL can normalise the apoptotic factors and Bax/Bcl-2 ratio in HaCaT cells .
Figure 6. Regulation of apoptotic factor content in UVB-irradiated cells by TAL: (a, b) Changes in protein and gene levels of Caspase-3; (c, d) Changes in protein and gene levels of Caspase-9; (e, f) Changes in protein and gene levels of Bax/Bcl-2. (C: Control group; M: UVB irradiation model group).
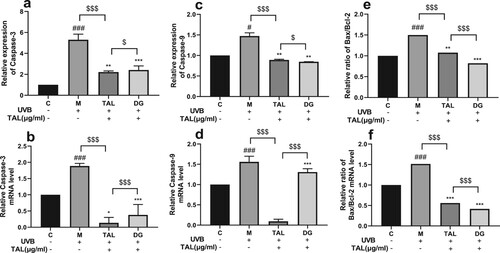
TAL can act on the MAPK/NF-κB pathway
The MAPK and NF-kb pathways are the signature pathways that activate the oxidative stress signal induced by UVB irradiation in HaCaT cells. To further study the mechanism of TAL repairing the damage of HaCaT cells irradiated by UVB, we used qPCR to detect the expression levels of JNK, ERK, P38, and NF-κB genes in the HaCaT cells. After the cells were irradiated with UVB, the gene expression of related proteins significantly increased, and the MAPK/NF-κB signalling pathway was activated. Compared with the model group, the gene expression of related proteins decreased after adding TAL and positive control DG. The results show that the gene expression of associated proteins on the MAPK/NF-κB signalling pathway significantly increased in HaCaT cells after UVB irradiation. Moreover, TAL can significantly regulate the expression of proteins on the MAPK/NF-κB signalling pathway .
Discussion
The skin is the first barrier guarding the functions of the whole human body. With the great depletion of the ozone layer, the UVB reaching the Earth's surface has increased significantly, which damages the skin barrier and causes skin inflammation, aging, oxidative stress, etc. (Hernández et al., Citation2019). However, there are few studies on repairing UVB-induced skin barrier damage in current reports. This study demonstrates that TAL has reparative effects on UVB-induced skin barrier damage in HaCaT cells. In , we also provide information on the mechanism of action (GA).
Keratinocytes are often used as cellular models for studying the mechanisms of inflammatory responses. In the current study, HaCaT cells were used to represent the response of human keratinocytes to UVB radiation in humans (Dias et al., Citation2021). As shown in , TAL was only toxic to cells at higher concentrations and could significantly repair UVB-induced cell damage. The results show that UVB increased ROS content in cells, whereas TAL and positive control DG could significantly reduce ROS content. It is well known that UV radiation exposure can damage the skin barrier by damaging certain important macromolecules in the skin.
In particular, UVB irradiation can increase the content of ROS radicals such as superoxide anion radicals (O2-) and hydroxyl radicals (OH), which can cause significant oxidative stress damage to the related proteins of the skin barrier (Hernández et al., Citation2019). In the epidermis, KLK7, FLG, EGF, and AQP3 proteins are involved in the function and moisturisation of the skin barrier. Studies have shown that the increase in FLG content can alleviate AD-like skin damage; KLK overexpression in the skin can spontaneously induce AD-like diseases (Q. Zhang et al., Citation2021); EGF promotes skin cell metabolism and cell proliferation, activates skin cells, and improves the skin barrier (Sandi & Susiani, Citation2022); and AQP3-promoted water transport is involved in cell migration and accelerated wound healing (Hara-Chikuma et al., Citation2009). We have confirmed that TAL can inhibit KLK7 overexpression and increase the protein levels of FLG, EGF, and AQP3 in cells after UVB damage.
The mechanism of the increase in skin damage and inflammation caused by UVB irradiation is as follows: with the occurrence of inflammation, the content of inflammatory factors secreted by keratinocytes increases, which is also one of the representative immune responses to inflammatory stimulation. IL-1β, IL-6, IL-8, and TNF-α are the most important inflammatory factors responsible for T cell differentiation, immunoglobulin production, neutrophil chemotaxis, and epithelial cell apoptosis induction, respectively (Akdis et al., Citation2016). UVB-induced cell damage leads to the activation of ROS-sensitive signalling pathways such as the MAPK pathway and NF-kb pathway (Im et al., Citation2016, Citation2019; Lingappan, Citation2018; Son et al., Citation2011). MAPK is a family of extracellular signal-regulated kinases (ERK), c-Jun NH2-terminal kinases (JNK), and p38 MAPKs. The NF-κB signalling pathway is induced by extracellular stimuli and plays a key role in regulating the immune response to infection. Inhibiting the activation of these two pathways reduces the inflammatory response, thereby minimising the occurrence of inflammatory diseases (Dias et al., Citation2021). In this study, HaCaT cells were irradiated with UVB and treated with TAL to detect changes in the levels of related genes. TAL was shown to significantly reduce the content of inflammatory factors and inhibit the activation of the MAPK/NF-κB signalling pathway.
Previous studies have shown that TAL has anti-inflammatory effects. However, its relevant mechanisms for repairing the skin barrier after UVB radiation have not been proven. In this study, we have further explored the possible mechanism of TAL in repairing the skin barrier by assessing the content of ROS, inflammatory factors, apoptotic factors, and skin barrier-related factors and restoring the MAPK/NF-κB signalling pathway.
Conclusion
The above results show that TAL can repair UVB-irradiated skin barrier damage by reducing oxidative stress, regulating the contents of inflammatory factors (TNF-a, IL-6, IL-8, and IL-1β), apoptotic factors (capase-3, capase-9, Bax, Bcl-2), and skin barrier-related factors (KLK7, AQP3, FLG, and EGF), and regulating the MAPK/NF-κB signalling pathway. This provides a theoretical basis for the future application of TAL as a cosmetic or medicinal skin barrier repair ingredient. However, more research is needed to flesh out this theory, such as animal experiments, omics, and other techniques.
Acknowledgements
The authors are grateful to the College of Chemistry and Materials Engineering, Beijing Technology and Business University, and Yunnan Baiyao Group Co., Ltd. for providing necessary lab facilities to carry out this work.
Disclosure statement
No potential conflict of interest was reported by the author(s).
References
- Agrawal, R., & Woodfolk, J. A. (2014). Skin barrier defects in atopic dermatitis. Current Allergy and Asthma Reports, 14(5), 1–11. https://doi.org/10.1007/s11882-014-0433-9.
- Akdis, M., Aab, A., Altunbulakli, C., Azkur, K., Costa, R. A., Crameri, R., Duan, S., Eiwegger, T., Eljaszewicz, A., Ferstl, R., Frei, R., Garbani, M., Globinska, A., Hess, L., Huitema, C., Kubo, T., Komlosi, Z., Konieczna, P., Kovacs, N., … Akdis, C. A. (2016). Interleukins (from IL-1 to IL-38), interferons, transforming growth factor β, and TNF-α: Receptors, functions, and roles in diseases. Journal of Allergy and Clinical Immunology, 138(4), 984–1010. https://doi.org/10.1016/j.jaci.2016.06.033
- Che, L., Li, Y., Song, R., Qin, C., Hao, W., Wang, B., Yang, L., Peng, P., & Xu, F. (2019). Anti-inflammatory and anti-apoptosis activity of taraxasterol in ulcerative colitis in vitro and in vivo. Experimental and Therapeutic Medicine, 2019(18), 1745–1751. https://doi.org/10.3892/ETM.2019.7736
- Chen, W., Li, J., Li, C., Fan, H. N., Zhang, J., & Zhu, J. S. (2020). Network pharmacology-based identification of the antitumor effects of taraxasterol in gastric cancer. International Journal of Immunopathology and Pharmacology, 34(600), 1–6. https://doi.org/10.1177/2058738420933107.
- Dias, M. K. H. M., Madusanka, D. M. D., Han, E. J., Kim, H. S., Jeon, Y. J., Jee, Y., Kim, K. N., Lee, K., Fernando, I. P. S., & Ahn, G. (2021). Sargassum horneri (turner) C. Agardh ethanol extract attenuates fine dust-induced inflammatory responses and impaired skin barrier functions in HaCaT keratinocytes. Journal of Ethnopharmacology, 273(January), 114003. https://doi.org/10.1016/j.jep.2021.114003
- Diffey, B. L. (2002). Sources and measurement of ultraviolet radiation. Methods, 28(1), 4–13. https://doi.org/10.1016/S1046-2023(02)00204-9
- Finch, P. W., & Rubin, J. S. (2004). Keratinocyte growth factor/fibroblast growth factor 7, a homeostatic factor with therapeutic potential for epithelial protection and repair. Advances in Cancer Research, 91, 69–136. https://doi.org/10.1016/S0065-230X(04)91003-2
- Hara, M., Ma, T., & Verkman, A. S. (2002). Selectively reduced glycerol in skin of aquaporin-3-deficient mice may account for impaired skin hydration, elasticity, and barrier recovery. Journal of Biological Chemistry, 277(48), 46616–46621. https://doi.org/10.1074/jbc.M209003200
- Hara-Chikuma, M., Takahashi, K., Chikuma, S., Verkman, A. S., & Miyachi, Y. (2009). The expression of differentiation markers in aquaporin-3 deficient epidermis. Archives of Dermatological Research, 301(3), 245–252. https://doi.org/10.1007/s00403-009-0927-9
- Hernández, A. R., Vallejo, B., Ruzgas, T., & Björklund, S. (2019). The effect of UVB irradiation and oxidative stress on the skin barrier—a new method to evaluate sun protection factor based on electrical impedance spectroscopy. Sensors, 19, 2376. https://doi.org/10.3390/s19102376
- Igawa, S., Kishibe, M., Minami-Hori, M., Honma, M., Tsujimura, H., Ishikawa, J., Fujimura, T., Murakami, M., & Ishida-Yamamoto, A. (2017). Incomplete KLK7 secretion and upregulated LEKTI expression underlie hyperkeratotic stratum corneum in atopic dermatitis. Journal of Investigative Dermatology, 137(2), 449–456. https://doi.org/10.1016/j.jid.2016.10.015
- Im, A. R., Park, I., Ji, K. Y., Lee, J. Y., Kim, K. M., Na, M., & Chae, S. (2019). The anti-obesity effects of tongbi-san in a high-fat diet-induced obese mouse model. BMC Complementary and Alternative Medicine, 19(1), 1–9. https://doi.org/10.1186/s12906-018-2420-5
- Im, A. R., Yeon, S. H., Lee, J. S., Um, K. A., Ahn, Y. J., & Chae, S. (2016). Protective effect of fermented cyclopia intermedia against UVB-induced damage in HaCaT human keratinocytes. BMC Complementary and Alternative Medicine, 16(1), 1–10. https://doi.org/10.1186/s12906-016-1218-6
- Jiang, S. H., Ping, L. F., Sun, F. Y., Wang, X. L., & Sun, Z. J. (2016). Protective effect of taraxasterol against rheumatoid arthritis by the modulation of inflammatory responses in mice. Experimental and Therapeutic Medicine, 12(6), 4035–4040. https://doi.org/10.3892/etm.2016.3860
- Kong, Y. C., Keung, W. M., Yip, T. T., Ko, K. M., Tsao, S. W., & Ng, M. H. (1987). Evidence that epidermal growth factor is present in swiftlet’s (collocalia) nest. Comparative Biochemistry and Physiology Part B: Comparative Biochemistry, 87(2), 221–226. https://doi.org/10.1016/0305-0491(87)90133-7
- Lingappan, K. (2018). NF-κB in oxidative stress. Current Opinion in Toxicology, 7, 81–86. https://doi.org/10.1016/j.cotox.2017.11.002
- Liu, B., He, Z., Wang, J., Xin, Z., Wang, J., Li, F., & Fu, Y. (2018). Taraxasterol inhibits LPS-induced inflammatory response in BV2 microglia cells by activating LXRα. Frontiers in Pharmacology, 9(APR), 1–7. https://doi.org/10.3389/fphar.2018.00278
- Lodén, M. (2003). Role of topical emollients and moisturizers in the treatment of Dry skin barrier disorders. American Journal of Clinical Dermatology, 4(11), 771–788. https://doi.org/10.2165/00128071-200304110-00005
- Park, J., Woo, Y. K., & Cho, H. J. (2020). Regulation of anti-oxidative, anti-inflammatory, and anti-apoptotic activity of advanced cooling composition (ACC) in UVB-irradiated human hacat keratinocytes. International Journal of Molecular Sciences, 21(18), 6527–6519. https://doi.org/10.3390/ijms21186527
- Park, S. H., Kim, J. G., Jang, Y. A., Bayazid, A. B., & Ou Lim, B. (2021). Fermented black rice and blueberry withlactobacillus plantarumMG4221 improve UVB-induced skin injury. Food and Agricultural Immunology, 32(1), 499–515. https://doi.org/10.1080/09540105.2021.1967300
- Piao, M. J., Hyun, Y. J., Cho, S. J., Kang, H. K., Yoo, E. S., Koh, Y. S., Lee, N. H., Ko, M. H., & Hyun, J. W. (2012). An ethanol extract derived from bonnemaisonia hamifera scavenges ultraviolet B (UVB) radiation-induced reactive oxygen species and attenuates UVB-induced cell damage in human keratinocytes. Marine Drugs, 10(12), 2826–2845. https://doi.org/10.3390/md10122826
- Rafferty, T. S., Beckett, G. J., Walker, C., Bisset, Y. C., & McKenzie, R. C. (2003). Selenium protects primary human keratinocytes from apoptosis induced by exposure to ultraviolet radiation. Clinical and Experimental Dermatology, 28(3), 294–300. https://doi.org/10.1046/j.1365-2230.2003.01254.x
- Sandi, D. A. D., & Susiani, E. F. (2022). Formulation of edible bird’s nest (aerodramus fuciphagus) from central kalimantan as skin whitening and moisturizing cream. Journal of Pharmacy and Bioallied Sciences, 13, 39–45. https://doi.org/10.4103/jpbs.JPBS
- Segre, J. A. (2006). Epidermal barrier formation and recovery in skin disorders. Journal of Clinical Investigation, 116(5), 1150–1158. https://doi.org/10.1172/JCI28521
- Son, Y., Cheong, Y.-K., Kim, N.-H., Chung, H.-T., Kang, D. G., & Pae, H.-O. (2011). Mitogen-Activated protein kinases and reactive oxygen species: How Can ROS activate MAPK pathways? Journal of Signal Transduction, 2011, 1–6. https://doi.org/10.1155/2011/792639
- Thönes, S., Rother, S., Wippold, T., Blaszkiewicz, J., Balamurugan, K., Moeller, S., Ruiz-Gómez, G., Schnabelrauch, M., Scharnweber, D., Saalbach, A., Rademann, J., Pisabarro, M. T., Hintze, V., & Anderegg, U. (2019). Hyaluronan/collagen hydrogels containing sulfated hyaluronan improve wound healing by sustained release of heparin-binding EGF-like growth factor. Acta Biomaterialia, 86, 135–147. https://doi.org/10.1016/j.actbio.2019.01.029
- Tonghui, M., Hara, M., Sougrat, R., Verbavatz, J. M., & Verkman, A. S. (2002). Impaired stratum corneum hydration in mice lacking epidermal water channel aquaporin-3. Journal of Biological Chemistry, 277(19), 17147–17153. https://doi.org/10.1074/jbc.M200925200
- Vickery, B. P. (2007). Skin barrier function in atopic dermatitis. Current Opinion in Pediatrics, 19(1), 89–93. https://doi.org/10.1097/MOP.0b013e328012315a
- Wang, Y., Li, G. H., Liu, X. Y., Xu, L., Wang, S. S., & Zhang, X. M. (2016). In vivo anti-inflammatory effects of taraxasterol against animal models. African Journal of Traditional, Complementary and Alternative Medicines, 14(1), 43–51. https://doi.org/10.21010/ajtcam.v14i1.6
- Yosipovitch, G., Misery, L., Proksch, E., Metz, M., Ständer, S., & Schmelz, M. (2019). Skin barrier damage and itch: Review of mechanisms, topical management and future directions. Acta Dermato Venereologica, 99(13), 1201–1209. https://doi.org/10.2340/00015555-3296
- Zhang, C., Xie, X., Yuan, Y., Wang, Y., Zhou, M., Li, X., & Zhen, P. (2020). MiR-664 protects against UVB radiation-induced HaCaT cell damage via downregulating ARMC8. Dose-Response, 18(2), 155932582092923–9. https://doi.org/10.1177/1559325820929234
- Zhang, Q., Jiang, H., Liu, M., Li, X., Zhou, M., Lyu, Y., Huang, J., Chen, S., & Wang, L. (2021). Therapeutic effects of quinine in a mouse model of atopic dermatitis. Molecular Medicine Reports, 23(5), 1–9. https://doi.org/10.3892/mmr.2021.11952