Abstract
The glass transition temperature (Tg) of seed coat was studied in relation to water uptake behavior. Tg values of seed coats were determined using differential scanning calorimetry (DSC) for selected pea and bean varieties. Rapidly hydrating samples showed a sharp Tg near ambient temperature with a range spanning 4–10°C. Samples that demonstrated a lag and consequently slow hydration exhibited a broad Tg above ambient temperature with a range spanning 22–33°C. Slow hydrating samples were processed via cyclic heating and cooling above and below Tg, resulting in elimination of the lag phase and markedly improved hydration rates. This research showed that Tg can be related to water uptake behavior and can be used as a parameter to determine processing conditions for improving water uptake in seeds.
Keywords:
INTRODUCTION
The permeability of the seed coats of the Leguminoseae family has been studied extensively as poor hydration behavior is commonly observed in seeds belonging to this family.[Citation1] Poorly hydrating seeds exhibit a barrier to water permeability and can be considered impermeable or hard seeds. A permeable (normal) seed imbibes water readily when available while an impermeable (hard) seed does not take up water for hours, days or even longer. However, despite a long, variable lag before the start of imbibition, once water uptake begins the rate is comparable to that of permeable seeds.[Citation2] Thus, it is the time needed before the start of water uptake that determines seed permeabiltiy. Biologically, hard seededness is the reason for seed coat imposed dormancy and it is biologically beneficial for long term seed survival in adverse growing conditions.[Citation3]
However, hard seededness is undesirable for the food processing industry as rapidly and uniformly hydrating seeds are desirable. Commercial processing of dry peas and beans usually involves soaking the seeds overnight (12–16 h) in water at ambient temperature to encourage maximum water uptake.[Citation4] Although water is a relatively inexpensive ingredient, time and costs associated with transporting hydrated seeds (typically canned) are relatively expensive factors. Therefore, seed coat permeability is an issue that is important to both the scientific and industrial communities and much research has been devoted to understanding the cause and ultimate control of hard seededness.[Citation2, Citation4, Citation5, Citation6]
There is agreement in the literature indicating that the seed coat of a seed is the principle barrier to water uptake. The work of King and Ashton [Citation7] indicated that soybeans with partially abraded seed coats exhibited a higher rate of water absorption than soybeans with whole seed coats. Also, in order to understand differences in seed permeability, the physical, morphological, and chemical characteristics of the seed coat has been extensively studied. A review of the literature shows that seed permeability, with respect to seed coat structure, has been studied from a food science and a plant anatomy perspective. Work in food science has accounted for physical differences between impermeable and permeable seeds, while work in botany has investigated differences between the morphology and anatomy of permeable and impermeable seeds. In the food science literature, Reyes-Moreno et al. [Citation8] reported that permeable seeds exhibited a lower percentage of seed coat relative to whole seed mass, thinner seed coats, and a larger surface area than hard seeds. Sefa-Dedeh and Stanley [Citation9] performed a study on cowpeas and attributed differences in thickness and topography of seed coats to differences between permeable and impermeable seeds.
Botanists have studied the morphology and anatomy of a seed coat with the aim of understanding water uptake in seeds. There are two opinions regarding the role of the seed coat on water uptake. One is that the seed coat has specialized regions for water loss and uptake such as the hilum, micropyle, and lens while the second opinion is that the whole seed coat is involved in water exchange.[Citation6] Recent work by Ma et al. [Citation3] and Zeng et al. [Citation6] have stated that the whole seed coat should be regarded as an integrated system responsible for water absorbing properties as the palisade layer, which develops from the outer epidermis of the seed coat, plays a significant role in water uptake. In fact, the cuticle of the palisade layer has been implicated as the key factor that determines the permeability of the soybean seed coat.[Citation3] Work of Rangaswamy and Nandakumar [Citation1] has also attributed impermeability of seeds to differences in the seed coat structures such as contracted palisade cells and a thick cuticle.
There are numerous reports in the literature citing chemical differences in the seed coats of impermeable and permeable seeds.[Citation1, Citation2, Citation5, Citation8] Understanding the chemical composition of the cuticle may help to provide the most information on seed coat permeability due to its role in seed coat permeability. Egerton-Warburton [Citation10] has described the seed coat cuticle as being functionally analogous to the cuticular layers in other organs of plants, such as leaves. Cuticles are chemically heterogeneous consisting mainly of a wax fraction, soluble in common organic solvents, and an insoluble lipophilic matrix, namely cutin, which constitutes the framework of the cuticle.[Citation11] Cutin is a high molecular weight polyester composed of various inter-esterified C16 and C18 hydroxyalkanoic acids. Structural and physicochemical studies on cutin have shown that cutin exhibits an amorphous structure. Work in the literature has not focused on relating the chemistry of the cuticle of the seed coat and permeability, however the loss of lipids in the seed coat has been strongly suggested to be related to loss of hard seededness.[Citation6]
The permeability of the seed coat may be affected by the mechanical properties of the cuticle. A permeable seed coat is mechanically weak and possesses small fractures through which water can pass while the cuticle of an impermeable seed coat is mechanically strong and does not contain any fractures.[Citation3, Citation6] The mechanical properties of a material are influenced by the polymers that are present in the material (i.e., their chemical composition, molecular arrangement, and interaction of the molecules) along with temperature and moisture content.[Citation12] The glass transition temperature (Tg) is a polymer science concept that has been applied to food science research for studying the material properties of biopolymers.[Citation13, Citation14] The Tg is a second order phase transition and the stability and mechanical properties of a material vary depending on whether the material is above or below the Tg.[Citation15, Citation16] With respect to material stability, the phase transition behavior of non-leguminous plant cuticles has been studied in relation to water loss. A second order phase transition was noted to occur at a temperature that coincided with a remarkable increase in the water permeability behavior of the plant cuticles.[Citation11, Citation17] This seems to imply that the Tg of a seed coat might influence water uptake behavior. Furthermore, one of the main changes in the seed coat that occurs in the natural softening process that produces permeable seeds is the development of fractures in the seed coat. In general the development of fractures has been attributed to the alternating temperatures in the areas that some legumes are grown.[Citation6, Citation18] Zeng et al. [Citation6] studied legumes grown in the Mediterranean, where it was noted that growing conditions ranged from 17 to 70°C on the soil surface. It was hypothesized that as the temperature rose and fell the seed coat expanded and contracted, gradually weakening the structure of the seed coat. The repeated expansion and contraction of the seed coat was thought to have created a mechanical force from the cumulative heating energy of high and fluctuating temperatures, which created fractures in the seed coat and aided in creating seeds with good water uptake characteristics (i.e. permeable seeds). Perdon et al. [Citation14] cited the importance of the expansion and contraction of rice kernels and crack formation during drying. They also used the concept of the Tg and its effect on mechanical properties to explain crack formation observed during rice drying.
In this work, there were two main objectives. The first objective was to measure the Tg of the seed coat of different beans and peas and relate Tg with water uptake behavior. The second objective was to utilize Tg as a processing parameter and subject the seeds to processing conditions above and below the Tg with the aim of imposing expansion and contraction in the seed coat to potentially create fractures within the seed coat and alter the physico-chemical properties of the seed coat in order to modify the water uptake behavior.
MATERIALS AND METHODS
Samples
Green (Stratus) and yellow (Mozart) peas were obtained from the Crop Development Center in Saskatoon, Saskatchewan. Pinto (AC Ole and AC Pintoba), Black (CDC Jet), and Navy (Galley and Morden) beans were obtained from the Agriculture and Agrifood Canada Station in Morden, Manitoba. All seed samples were originally stored under ambient conditions. Sub-samples of all of the pea and bean varieties were taken for seed coat removal. The seed coats were removed using a 38.1 mm long needle. The remaining seeds were reserved for processing and hydration rate studies. Seed coat thickness was measured with a digital caliper.
Sub-samples of the seed coat were obtained and were subjected to different conditions. These different conditions included: 1) leaving the moisture content of the seed coat in an unaltered state by storing at ambient RH at 25°C in a polyethylene food storage bag until testing; and 2) altering the moisture content to a common value by placing the seed coats in different relative humidity (RH) environments. The salts used were sodium chloride (NaCl) and potassium chloride (KCl) (Sigma Chemicals, St. Louis, MO), and they produced the following RH environments respectively, 75 and 85% at 25°C. The moisture content of the seed coats was determined by drying duplicate samples for 24 h in an air oven set at 130°C. The moisture content of whole seeds was determined using the same procedure and it is noted that whole seeds were ground before moisture content determination. The whole seeds were ground before moisture content to ensure adequate drying occurred as the seed coat is the principal barrier to water migration.
DSC Experiments
The Tg of the seed coats was measured with differential scanning calorimetry (DSC) (Micro DSC III, Setaram, Caluire, France). Hastelloy C276 sample vessels with a height of 19.5 mm and an inner diameter of 6.4 mm were used. A scanning rate of 1.2°C/min and a temperature range of -20 to 120°C were employed. This slow scanning rate was in agreement with the heating rate used by Lin et al. [Citation15] to study the glass transition behavior of soybean and celery cell walls; however, it is noted that in food science literature it is most common to use a heating rate of 5°C/min for measuring the glass transition of foods.[Citation19] The DSC was calibrated with water and naphthalene (80.6°C). At least 35 mg of seed coat was placed in the bottom of the sample vessel. A mortar and pestle and a sharp blade were used to reduce the particle size of the seed coats where necessary. An empty vessel was used for the reference. The Tg of each thermogram was determined by identifying the transition corresponding to a slope change in the heat capacity of the sample. Tg measurements were performed in duplicate.
Also the gelatinization temperature (Tgelat) of the cotyledons was investigated to determine if the processing regimes used for modifying water uptake had any effect on the cotyledon material of the seeds. Seed coats were removed from the cotyledon manually with a needle. The cotyledon material was ground with a coffee grinder to reduce particle size and sieved to pass an 825μm sieve. Samples of cotyledon flour weighing between 5 and 7 mg were accurately weighed into sample pans and water was added to create a 2:1 proportion of water/flour. Sample pans were sealed and equilibrated for 1 h. DSC thermograms were obtained with a DuPont 990 thermal analyzer and a 910 DSC cell base. Samples were heated from 25 to 140°C at a rate of 10°C/min. Water was used in the reference pan. Analyses were performed in triplicate.
Processing Regimes for Modifying Water Uptake
Only two types of beans were processed with the aim of modifying water uptake behavior. Peas were not processed to modify water uptake behavior as they absorbed water relatively faster than beans. The two bean types tested included: Pinto (AC Ole and AC Pintoba varieties); and Black (CDC Jet). Two different processing regimes were investigated: 1) cyclic heating and cooling and 2) constant/static heating. Samples were processed in both a native state (moisture content unaltered) and a tempered state (moisture content altered). Tempering was performed prior to processing and samples were mixed thoroughly with the predetermined amount of distilled water required to reach the desired tempering level. Tempering levels of 13, 16, and 24% were investigated. Uniformity of water absorption in the seeds was assured by shaking the samples every 30 min for the first hour, and then once every hour for at least 6 h. Total tempering time was 16 h. No unabsorbed water was visible upon completion of the tempering treatment. In the cyclic heating and cooling regime samples were heated to the desired temperature and held for 30 min in an air convection oven (Blue M, Blue Island, IL), and then the samples were removed from the oven and placed in a −20°C freezer for 3 min. The surface temperature of the seeds was measured with a digital thermometer and was noted to be 7 ± 0.6°C. This procedure was performed three times in total. In the constant heating regime the seeds were held at the same constant temperature for 90 (i.e., 3 × 30) min in an air convection oven. The heating temperatures employed were 45, 60, 85, and 160°C. Samples were allowed to cool for 30 min at ambient conditions (25°C, 35% RH) before being sealed in polyethylene food storage bags.
Abrasion Processing
An abrasion treatment was investigated as a method of altering water uptake of the whole seeds through abrading the seed coat surface. A plastic jar (12 cm diameter by 14.5 cm height) was lined with sandpaper (3M, P220). Marbles were also added to the jar to provide weight. Whole seeds were placed in the jar and the jar was placed on a Ball Mill (Norten, Akron, OH) and rotated for 40 min. The water uptake behavior of the seeds was examined.
Hydration Rates
After processing, the beans were subjected to hydration rate measurements using 10 seeds. The initial mass of the seeds was determined and the seeds were placed at the bottom of a 300 ml beaker. Distilled water was allowed to equilibrate to room temperature (24°C) for 16 h prior to testing. 200 ml of distilled water was added to the seeds. Every 30 min, the seeds were removed from the beaker, blotted on a paper towel to remove surface water, and weighed. Hydration rates were measured up to 8 h. As a check to determine whether the processing regime modified the water uptake behavior of the seed coat or the water uptake behavior of the cotyledon, the seed coat was manually removed from the unprocessed and processed seeds and water uptake behavior was examined in the same manner as that noted above. Water uptake was calculated as the difference between the starting mass and mass at the selected time points.
Statistical Analysis
All data were analyzed using analysis of variance (ANOVA) and Tukey mean separation with the Statistical Analysis System (SAS) Program (SAS Institute, Carey, NC). Significance was accepted at p ≤ 0.05.
RESULTS AND DISCUSSION
Relating Tg and Water Uptake Behavior
shows the results for the Tg of the seed coats of selected pulses that have been conditioned in ambient, 75, and 85% RH environments. It has been noted that the glass transition of biological materials is highly variable and experimental measurements performed on biological materials may yield high standard deviations.[Citation20, Citation21] It is acknowledged that the glass transition of a heterogeneous material (as the seed coat used in this research) can be expected to be broad and difficult to measure.[Citation22] In essence, some may argue that it may be difficult to obtain meaningful glass transition measurements from multi-component materials. However, there are numerous reports in the literature stating the technological importance on determining the Tg values of heterogeneous biological materials and relating its measurement to biomaterial functionality and quality.[Citation14, Citation22, Citation23, Citation24] To the authors knowledge there have been no reports in the literature explicitly linking the glass transition behavior of seed coats and water uptake. However, there is a large volume of literature indicating that dormant seeds reside in the glassy state [Citation25, Citation26] as there is much agreement between the glass transition temperatures of seeds as a function of water content and their storage stability as a function of storage temperature and water content.[Citation16]
Table 1 Moisture content, Tg range and Tg midpoint measurements for the seed coats of selected pulses conditioned in various relative humidity (RH) environmentsFootnote∗.
The results in show that the seed coats of the pea (Mozart and Stratus) and navy bean (Morden and Galley) samples have a Tg near room temperature (20–34°C) and the glass transition temperature range covers a relatively narrow 10°C range around the Tg. Representative DSC thermograms are given in and for the seed coats of Mozart and Galley, respectively. The arrows in and denote the Tg ranges observed for those samples. The DSC results for Galley () and Morden (not shown) seem to indicate the presence of two second order transition temperature ranges. Multiple second order transitions have been obtained during the characterization of brown rice thermal properties.[Citation14] These multiple second order transitions were attributed to the high degree of heterogeneity observed in the rice kernels and also due to the different conformations and interactions between the amylose, amylopectin, and other components present in rice. Seed coats are highly heterogeneous containing polysaccharides, lipids, waxes, polyphenolics, lignin, sugars, and proteins.[Citation27, Citation28] It is possible that a seed coat may contain multiple thermal transitions. The beans AC Ole, AC Pintoba (pinto), and CDC Jet (black) exhibit a glass transition temperature relatively higher than room temperature ranging from 37 to 81°C. A representative DSC thermogram for the seed coat of AC Ole is given in . Also shown in are the thermal characteristics of the seed coat of AC Ole seeds that have been subjected to processing, these results will be further discussed in a later section. Upon thermal characterization, AC Pintoba and CDC Jet also presented multiple second order transitions. Considering each multiple transition temperature as distinct, some of the transition temperature ranges cover a broad 30°C span around the thermal transition. Again this type of temperature span around the transition temperature is acceptable for heterogeneous biological materials.[Citation26]
Figure 1 (a) Representative DSC Thermogram for Mozart (MZ, yellow pea, ambient environment). Tg (R1) = Range 1 glass transition; (b) representative DSC Thermogram for Galley (GY, navy bean, ambient environment). Tg (R1) = Range 1 glass transition; Tg (R2) = Range 2 glass transition; and (c) representative DSC Thermogram for unprocessed AC Ole (pinto bean, ambient environment) and processed AC Ole (tempered at16% moisture and subjected to 3 cycle heating (60 °C) and cooling (7 °C) regime). Tg (R1) = Range 1 glass transition.
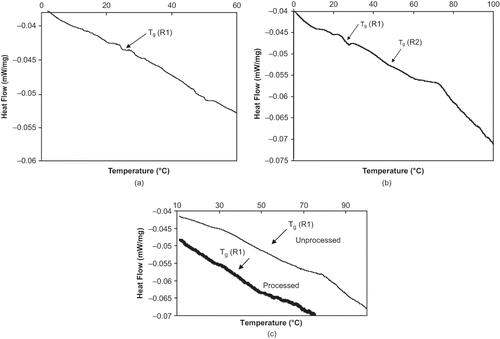
A question that needs to be addressed is that of which second order transition temperature should be associated with the glass transition temperature. Perdon et al.[Citation14] indicated the presence of multiple second order transitions associated with the thermal characterization of rice kernels and stated that the second order transition associated with the glass transition temperature was the one that was correlated (r = −0.75, p ≤ 0.01) with moisture content. From this it might be assumed that the Tg value that should be assigned to each pulse seed coat is associated with the Tg range that exhibited a statistically significant decrease as moisture content of the seed coat was increased (i.e. seeds coat conditioned in relative humidity environments with increasing levels of moisture). There was only one Tg range reported for Stratus and AC Ole; the Tg values determined for these seed coats significantly decreased as moisture content increased. The seed coats of AC Pintoba and CDC Jet showed multiple thermal transitions. The Tg values associated with the Tg of range 2 (AC Pintoba) and the Tg of range 1 (CDC Jet) showed Tg values that significantly decreased upon increase in moisture content. Notably, the Tg values for Mozart and Galley did not show a significant decrease upon increase in moisture content. This may be due to the relatively high variability observed with these samples and the modest correlation (r = – 0.75) cited by Perdon et al.[Citation14] between moisture content and Tg. These facts may work together to prevent the samples from presenting statistically significant differences in Tg as affected by moisture content.
The consequences of different glass transition temperatures on the water uptake behavior of seeds may be inferred from . shows the water uptake behavior of unprocessed/unmodified peas (Mozart and Stratus) and beans (Galley, Morden, AC Ole, AC Pintoba, and CDC Jet). The pulses with relatively fast water uptake (i.e., no lag before substantial water uptake) all have Tg values relatively close to room temperature and a narrow Tg range, while the relatively slowly hydrating samples (i.e. an appreciable lag before water uptake begins) have Tg values greater than room temperature and a broader Tg range. Admittedly, the seed coats from samples with relatively faster hydration are thinner than the seed coats of the samples with slower hydration (see ). Seed coat thickness has been noted in the literature as a factor affecting water uptake. Seeds with thicker seed coats have been shown in the literature to have slower water uptake rates.[Citation7, Citation9] However Sefa-Dedeh and Stanley [Citation9] noted that certain varieties of the cowpeas possessed thicker seed coats than other cowpea varieties yet exhibited faster water uptake than cowpeas with thinner seed coats. Water uptake behavior cannot solely be linked to seed coat thickness.
Seed size has also been linked to water uptake behavior.[Citation2, Citation29, Citation30], Water uptake is determined via mass uptake such that a smaller seed would exhibit a proportionally greater increase in water mass uptake than a larger seed if both were taking up water at the same rate. Therefore, a difference in size could account for different water uptake rates but it does not offer an explanation for different starting times of water uptake (i.e. lag times).[Citation2] The glass transition temperature of the seed coat may offer an explanation for the lag time or different starting times for water uptake. The water uptake behavior of different of lentils, chickpeas, and beans was studied by Seyhan-Gurtas et al. [Citation30] using a diffusion model, which accounted for size, shape, and moisture content of the seeds. They noted that their diffusion model described the water uptake behavior of the lentils and chickpeas very well. However the diffusion model described the water uptake behavior of all but one of the bean varieties poorly, particularly at 15 and 25°C. The poor agreement between the diffusion model and the experimental water uptake values for certain bean varieties at 15 and 25°C was attributed to the lag phase exhibited by these bean varieties before conforming to a typical diffusion pattern.[Citation30] These authors explicitly stated that the lag practically disappeared at 40°C which indicated a temperature sensitivity of the mechanism responsible for the initial lag phase. Arechavaleta-Medina and Snyder [Citation2] also cited the effect of temperature on lessening the lag time observed in the water uptake of soybeans. They indicated that the cuticular layer of the soybean may be a key factor in water uptake behavior as it may be the site of the moisture barrier. They indicated that soaking beans in polar solvents such as methanol and ethanol effectively removed the lag phase before water uptake. These facts implicitly indicate the importance of the physico-chemical properties of the seed coat. Egerton-Warburton [Citation10] indicated that rapidly hydrating/permeable seeds possess seed coats containing cuticular waxes whose structure have been plasticized and possess an altered hydrophobicity. From this it is possible that the Tg of a seed coat might influence water uptake. The phase transition behavior of non-leguminous plant uticles has been studied in relation to water loss and a marked second order transition has been noted to occur at a temperature that coincides with a remarkable increase in the water permeability.[Citation31] Schreiber and Schonherr [Citation31] found that isolated cuticles from various non-leguminous plant species exhibited a second order transition between 40 and 50°C. Matas et al. [Citation32] indicated that isolated cuticles and cutin from young tomato plants demonstrated a Tg around 23°C and with water absorption shifted the Tg to 16.3°C. Matas et al. [Citation32, Citation33] noted that the second order phase transition determines the rheological and mechanical properties of the biopolyester cutin and in turn determines mass transfer between the environment and plant cell. It follows that a similar situation may exist regarding the water uptake behavior of seeds.
Modifying Water Uptake Behavior through Processing
shows the modified water uptake behavior for AC Ole beans processed under different conditions. Although results for both the AC Pintoba and CDC Jet beans are not shown, generally similar results were observed. In all cases, cyclic heating (45, 60, and 85°C) and cooling (7°C) and static heating (45, 60, and 85°C) processing regimes on the untempered beans in their native state did not produce significantly improved water uptake behavior compared against the control seeds. AC Ole and AC Pintoba seeds that were tempered to 16% moisture content and subjected to a 45, 60, and 85°C cyclic heating and cooling treatment exhibited a significant increase in their water uptake behavior compared against the control seeds. CDC Jet seeds that were tempered to 16% and subjected to cyclic heating at 85°C and cooling showed significantly better water uptake than control seeds. However, most significant differences in water uptake behavior of the 16% stopped after 4 h of water uptake as there were no observed significant differences in water uptake at 8 h. The results seem to indicate that both water and heat are required to induce the changes necessary to improve water uptake. Also, there were no significant differences between the water uptake behavior of the 16% tempered AC Ole and the water uptake behavior of the seeds that were heated at 45, 60, and 85°C. The 16% tempered AC Pintoba seeds subjected to 45 and 60°C cyclic heating and cooling showed significantly better water uptake than the 16% tempered seeds subjected to 85°C cyclic heating and cooling. This implies that the least aggressive and energy intensive treatment at 45°C may be employed to elicit improved water uptake behavior. Interestingly the Tg of the seed coat of AC Ole (51.6°C) was higher than the Tg of the seed coat of AC Pintoba (45.2°C). These results seem to indicate that the upper limit of the processing temperature which causes improved water uptake behavior may be influenced by the Tg of the seed coat. AC Ole and CDC Jet seeds in their native state or tempered to 16% when subjected to cyclic heating at 160°C and cooling showed significantly reduced water uptake compared to the control samples at the 8 h time point. It appears that heating at 160°C is detrimental to the seeds ability to imbibe water.
Figure 3 (a) Water uptake behavior of unprocessed whole seeds (AC Ole, pinto bean) processed at 45°C; (b) water uptake behavior of unprocessed whole seeds (AC Ole, pinto bean) processed at 60°C; (c) water uptake behavior of unprocessed whole seeds (AC Ole, pinto bean) processed at 85°C; and (d) water uptake behavior of unprocessed whole seeds (AC Ole, pinto bean) processed at 160°C.
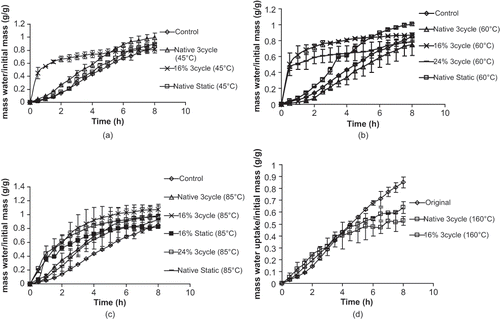
The effect of tempering moisture content on water uptake behavior was also examined. There were no significant differences in the water uptake behavior of the 16% and 24% tempered seeds at common heat treatments. For AC Ole seeds subjected to cyclic heating at 85°C and cooling, the water uptake behavior of the 13% tempered seeds compared against the 16 and 24% tempered seeds was significantly less. These results seem to indicate that the 16% may be the most efficient tempering level to employ when modifying water uptake.
The effect of a cooling step was investigated. Results indicate that the 16% tempered AC Ole and AC Pintoba seeds subjected to cyclic heating and cooling did not show significantly better water uptake compared against the 16% tempered seeds subjected to static heating. The results seem to indicate that the cooling step was not required to cause improved water uptake behavior. This was somewhat unexpected as the presence of small cracks in the seed coat has been attributed to improved water uptake in seeds. The literature has implicated the expansion and contraction experienced by the seed coat during growing conditions as a cause of stress gradients which ultimately creates cracks in the seed coat.[Citation3, Citation6] shows SEM images of the outer surface of the seed coat of a control and processed seed with improved water uptake. There were no cracks observed in the processed seeds compared to the control seeds. There were more pronounced surface spheres observed on seed coat of the processed seeds exhibiting improved hydration. Egerton-Warburton [Citation10] also observed more surface spheres on Emmenanthe penduliflor Benth. (Hydrophyllaceae) seeds that were chemically scarified with smoke treatment compared to dormant seeds. Egerton-Warburton [Citation10] noted that the observed changes in the external cuticle of the seed coat were most likely due to physico-chemical alteration in the conformations of the waxes present, which resulted in an increased surface area of the seed for the exchange of water and solutes, and an alteration in the hydrophobicity of the seed coat.
Figure 4 (a) SEM of outer surface of seed coat (AC Ole-control); and (b) SEM of outer surface of seed coat (AC Ole: 16% 3cycle treatment (60°C) processing treatment.
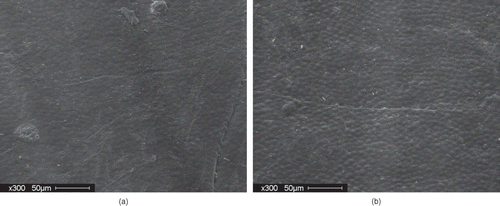
Citing physico-chemical changes in the seed coat as a factor in creating seeds with good water uptake behavior is consistent with the seminal work of Taylor.[Citation18] Taylor [Citation18, Citation34] indicated that two distinct temperature dependent processes are involved in creating seeds with good water uptake behavior and the two stage process includes both physical and chemical changes. The first stage has been attributed to a period where seeds are subjected to temperatures ranging from 30–60°C. The first stage is likely a thermal degradation process in which changes the polymeric structure of lipid materials present in the seed coat occur through a weakening of hydrophobic interactions.[Citation6] The second stage has been attributed to temperature fluctuations ranging from 60 to 15°C or 35 to 10°C[Citation34]. It has been indicated in the literature that cracks are produced in the second stage of the seed softening process.[Citation3, Citation6] Taylor [Citation18] indicated that the rate of change of temperature fluctuations were important in producing seeds with good water uptake behavior. A slow rate of temperature change produced seeds with better water uptake behavior than a fast rate of temperature change. It is likely that the cyclic heating and cooling regime employed in this work was too fast to create the stress gradients within the seeds coat necessary to produce cracks in the seed coats. However, Zeng et al. [Citation6] also implied that lipid hydrolysis was important in Taylor's second stage of creating well hydrating seeds. The cooler temperatures encountered during field growing conditions were linked with increased water availability, which is relevant as water is necessary for the chemical process of lipid hydrolysis. This is likely the reason the tempered seeds showed improved water uptake compared to the native seeds subjected to the same heating and cooling conditions. It should be noted that the processing regimes employed in our experiments were not two-stage in nature; a second stage processing regime was employed. It is conceivable that if the seeds were subjected to a first stage of elevated temperature conditioning followed by either a second stage of heating and cooling or static heating, the seeds processed by the cyclic heating and cooling may have exhibited improved water uptake compared to the seeds subjected to static heating. Nevertheless, it is likely that seeds undergo the fist stage of the process during growing conditions in the field and the food processor is responsible for the second stage. Also, the scope of this study was to investigate a processing regime that is both time and cost effective; Taylor [Citation18] indicated that the duration of the first stage of treatment may last 168 days. Finally, Taylor [Citation18] indicated that subjecting seeds to the second stage of seed softening introduces additional first stage thermal degradation. Therefore, the processing treatments employed in this work are consistent with the work of Taylor [Citation18, Citation34] citing the importance of a two stage softening process and its time and energy efficient.
In order to examine whether physico-chemical changes of the seed coat were induced via processing, the Tg of the seed coat from AC Ole seeds that were tempered at 16% and subjected to cyclic heating at 60°C and cooling was measured. shows a comparison of the thermal characteristics of the seed coats of unprocessed and 16% tempered, 60°C cyclic heated AC Ole seeds. The measured Tg for the seed coat of the 16% tempered 60°C cyclic heated AC Ole seeds was 37.7 ± 1.45°C and the Tg range was 33–47°C. The measured Tg for the seed coat of unprocessed Ole was 51.6 ± 0.2°C and the Tg range was 33–66°C. It appears that the Tg range is less broad for the seed coat from the 16% tempered 60°C cyclic heated seeds and the Tg shifted to a lower temperature. The moisture content of the seed coat of the 16% tempered 60°C cyclic heated AC Ole seeds was 9.4% (± 0.32) and the moisture content of the seed coat of the unprocessed AC Ole seeds was 9.6% (± 0.04) and therefore moisture content was not a factor in the decreased Tg observed in the seed coat of the 16% tempered 60°C cyclic heated AC Ole seeds. From these results it appears that subjecting seed to water and heat alters the physico-chemical properties of the seeds coats.
In order to ensure that the improved water uptake behavior was due to modifying the seed coat and not the cotyledon material of the seeds, the seed coat was removed from processed and control seeds and water uptake was measured. The processed AC Ole () and AC Pintoba (results not shown) seeds with seed coats removed (i.e. cotyledon material) did not show significantly better water uptake than the control cotyledon material. In fact, the control cotyledons showed significantly better water uptake than the 16% tempered 85°C cyclic heated and cooled cotyledon material. shows gelatinization and denaturation temperatures of the cotyledon material of the control and processed seeds. There were no significant differences between the processed seeds and the control seeds. The heat treatments employed in this work did not cause any gelatinization of starch or protein denaturation. These results were somewhat expected as heating in limited water, as the tempering levels used in these experiments were, is unlikely to cause appreciable starch gelatinization.[Citation35] The starch gelatinization temperatures and protein denaturation temperatures obtained in our experimental work are in agreement with the values reported by Reyes-Moreno et al. [Citation8] and Bernal-Lugo et al..[Citation36] Biliaderis et al. [Citation37] reported the starch gelatinization behavior of various starches studied by differential scanning calorimetry. They noted the presence of a second peak following that of the starch gelatinization; they attributed the second peak to be caused by starches with a more crystalline structure, primarily amylose. Roos [Citation16] has since identified the second peak as melting of the amylose lipid complex. Nevertheless, Biliadaris et al. [Citation37] indicated that the second peak was eliminated when a starch system of nearly 80% water was studied with DSC. An auxiliary experiment was performed in this work where a starch system of 80% water was studied with DSC. The second peak remained and it was concluded to be a peak due to protein denaturation.
Table 2 Starch gelatininzation (Tgelat) and protein denaturation (Tdenat) temperatures of the cotyledon material of Ole and Pinto beans subjected to different processing regimesFootnote∗.
also shows results for AC Ole seeds whose seed coats were subjected to abrasion using a jar lined with sandpaper and rotating on a ball mill. The abraded AC Ole seeds showed significantly better water uptake than the cotyledon material, both control and processed. While initially the seed coat may present a barrier to water uptake, once abraded or processed appropriately it may serve to act as a sponge or a physical barrier keeping absorbed water in the seed, presumably residing between the seed coat and the endosperm which is in agreement of work on Emmenanthe penduliflora seeds by Egerton-Warburton.[Citation10]
SEM images of the transverse sections of the seed coat of control and processed seed coats of AC Ole are shown in , respectively. There are three observed layers in the control seed coats: 1) a palisade layer (outer layer), 2) parenchyma cells which connects the palisade layer to the 3) endosperm. In the processed seeds there are only two layers, 1) a palisade layer and 2) a parenchyma layer. It seems that processing may cause a disintegration of the material connecting the parenchyma and endosperm. Egerton-Warburton [Citation10] indicated that for Emmenanthe penduliflora seeds this connecting layer was the internal cuticle. The inner cuticle layer was noted to be comprised of cutin, lipids, polysaccharides, acidic carbohydrates, and polyphenolic compounds. The breakdown of cutin and lipids through the action thermal degradation and heat is consistent with the work of Taylor [Citation18, Citation34] and consistent with the observed change in the Tg of the seed coat of processed seeds ().
Figure 6 (a) SEM of seed coat (transverse section: AC Ole control) p, palisade layer, pa, parenchyma, and en, endosperm material; and (b) SEM of seed coat (transverse section: AC Ole 16% 3cycle, (60 °C) processing treatment) p, palisade layer, and pa, parenchyma.
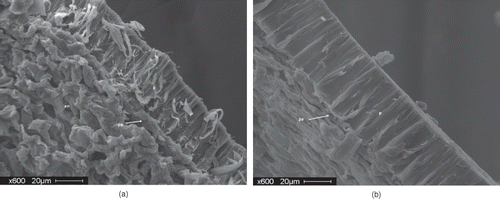
Work has been performed by Zhang et al. [Citation38] on high pressure hydration treatment for soybean processing, which indicated that soybeans that had been exposed to a high pressure hydration treatment showed improved water uptake. They investigated the hilum of the soybean seed and noted that control soybeans exhibited an unbroken network possessing the appearance of a sponge, while processed soybeans showed a broken network. They attributed part of the improved water uptake seen in processed soybean to be due to the presence of a broken network in the hilum. For completeness we investigated both the hilum and micropyle region of control and processed seeds with SEM with the aim of probing differences in their structure. SEM images of the hilum of AC Ole are presented in for the control and processed seeds, respectively. It appears from that the hilum structures of control and processed seeds are comparable. Furthermore, the hilum structures presented in are comparable to those presented by Zhang et al.[Citation38] of the hilum of the unprocessed soybean seeds and they are clearly different from the hilum high pressure processed soybean seeds which show a discernable broken network. The micropyle showed no observable differences either (results not shown). From all of this work, it appears that water and heat act to degrade cutin, wax and lipids present in the seed coat and improve water uptake.
CONCLUSIONS
Water uptake behavior is related to the Tg of pulse seed coat. Samples with good water uptake/no lag exhibited a sharp Tg near ambient temperature spanning 4–10°C, while samples with poor water uptake/lag exhibited a broad Tg above ambient temperature with a range spanning 22–33°C. The glass transition temperature of the seed coat was used as a processing parameter and the results indicate that heat and water are required to induce physico-chemical changes in the seed coat to improve water uptake rather than the presence of cracks due to stress gradients induced by cyclic heating and cooling.
REFERENCES
- Rangaswamy , N.S. and Nandakumar , L. 1985 . Correlative Studies on Seed Coat Structure, Chemical Composition, and Impermeability in the Legume Rhynchosia minima . Bot Gaz , 146 ( 4 ) : 501 – 509 .
- Arechavaleta-Medina , F. and Snyder , H.E. 1981 . Water Imbibition by Normal and Hard Soybeans . J. American Oil Chemical Society , 58 : 976 – 979 .
- Ma , F. , Cholewa , E. , Mohamed , T. , Peterson , C.A. and Gijzen , M. 2004 . Cracks in the Palisade Cuticle of Soybean Seed Coats Correlate with their Permeability to Water. Annals of Botany Vol. 94 , 213 – 228 .
- Thanos , A.J. 1998 . Water Changes in Canned Dry Peas and Bean During Heat Processing. . Int. J. Food Sci and Technol , 33 : 539 – 545 .
- Marbach , I. and Mayer , A.M. 1974 . “ Permeability of Seed Coats to Water as Related to Drying Conditions and Metabolism of Phenolics ” . In Plant Physiol Vol. 54 , 817 – 820 .
- Zeng , L.W. , Cocks , P.S. , Kailis , S.G. and Kuo , J. 2005 . The Role of Fractures and Lipids in the Seed Coat in the Loss of Hardseededness of Six Mediterranean Legume Species . J. Agricultural Science , 143 : 43 – 55 .
- King , R.D. and Ashton , S.J. 1985 . Effect of Seed Coat Thickness and Blanching on the Water Absorption by Soybeans . J. Food Technol , 20 : 505 – 509 .
- Reyes-Moreno , C. , Carabez-Trejo , A. , Paredes-Lopez , O. and Ordorica-Falomir , C. 1994 . “ Physicochemical and Structural Properties of Two Bean Varieties which Differ in Cooking Time and the HTC Characteristic. ” . In Lebensm-Wiss. u-Technol Vol. 27 , 331 – 336 .
- Sefa-Dedeh , S. and Stanley , D.W. 1979 . The Relationship of Microstructure of Cowpeas to Water Absorption and Dehulling Properties . Cereal Chem , 56 ( 4 ) : 379 – 386 .
- Egerton-Warburton , L.M. 1998 . A Smoke Induced Alteration of the Sub-Testa Cuticle in Seed of the Post Fire Recruiter, Emmenanthe penduliflora Benth. (Hydrophyllaceae) . J. Exp. Botany , 49 ( 325 ) : 1317 – 1327 .
- Casado , C.G. and Heredia , A. 2001 . “ Specific Heat Determination of Plant Barrier Lipophillic Components: Biological Implications ” . In Biochemica et Biophysica Acta Vol. 1511 , 291 – 296 .
- Hoseney , R.C. 1994 . “ Glass Transition and its Role in Cereals. ” . In Principles of Cereal Science and Technology , 307 – 320 . St. Paul, MN : American Association of Cereal Chemists .
- Brent , J.L. , Mulvaney , S.J. , Cohen , C. and Bartsch , J.A. 1997 . Thermomechanical Glass Transition of Extruded Cereal Melts . J. Cereal Sci , 26 : 301 – 312 .
- Perdon , A. , Siebenmorgan , T.J. and Mauronmoustakos , A. 2000 . “ Glassy state Transition and Rice Drying: Development of a Brown Rice State Diagram ” . In Cereal Chem Vol. 77 , 708 – 713 .
- Lin , L.S. , Yuen , H.K. and Varner , J.E. 1991 . “ Differential Scanning Calorimetry of Plant Cell Walls ” . In Proc. Natl. Acad. Sci Vol. 88 , 2241 – 2243 .
- Roos , Y.H. 1995 . Phase Transitions in Foods , 360 San Diego : Academic Press .
- Eckl , K. and Gruler , H. 1980 . “ Phase transitions in Plant Cuticles ” . In Planta Vol. 1150 , 102 – 113 .
- Taylor , G.B. 1981 . “ Effect of Constant Temperature Treatments Followed by Fluctuating Temperatures on the Softening of Hard Seeds of Trifolium subterraneum L. Australian ” . In J. Agric. Res Vol. 8 , 547 – 558 .
- Rahman , M.S. 2004 . State Diagram of Date Flesh Using Differential Scanning Calorimetry (DSC) . Int. J. Food Properties , 7 ( 3 ) : 407 – 428 .
- Gibson , L.J. , Easterling , K.E. and Ashby , M.F. 1981 . The Structure and Mechanics of Cork . Proc. R. Soc. Lond , A377 : 99 – 117 .
- Vincent , J.F.V. 1982 . The Mechanical Design of Grass . J. Mater. Sci , 17 : 856 – 860 .
- Irvine , G.M. 1985 . “ The Significance of the Glass Transition of Lignin in Thermomechanical Pulping ” . In Wood Sci. Technol Vol. 19 , 139 – 149 .
- LeMeste , M. , Huang , V.T. , Panama , J. , Anderson , G. and Lentz , R. 1992 . “ Glass Transition of Bread ” . In Cereal Foods World Vol. 37 , 264 – 267 .
- Peleg , M. 1995 . A Note on the Tan δ (T) Peak as a Glass Transition Indicator in Biosolids . Rheol. Acta , 34 : 215 – 220 .
- Williams , R.J. 1994 . “ Methods for Determination of Glass Transitions in Seeds ” . In Annals of Botany Vol. 74 , 525 – 530 .
- Tolstoguzov , V.B. 2000 . The Importance of the Glassy Biopolymer Components in food . Nahrung , 44 ( 2 ) : 76 – 84 .
- Graven , P. , DeKoster , C.G. , Boon , J.J. and Bouman , F. 1997 . Functional Aspects of Mature Seed Coat of the Cannaceae . Pl. Syst. Evol , 205 : 223 – 240 .
- Harris , W.M. 1987 . Comparative Ultrastructure of Developing Seed Coats of “Hard-Seeded” and “Soft-Seeded” Varieties of Soybean, Glycine max (L.) Merr , 148 ( 3 ) : 324 – 331 .
- Hsu , K.H. , Kim , C.J. and Wilson , L.A. 1983 . “ Factors Affecting Water Uptake of Soybeans During Soaking ” . In Cereal Chem Vol. 60 , 208 – 211 .
- Seyhan-Gurtas , F. , Ak , M.M. and Evranuz , E.O. 2001 . Water diffusion coefficients of selected legumes grown in Turkey as affected by temperature and varieity . Turk. J. Agric. For , 25 : 297 – 304 .
- Schreiber , L. and Schonherr , J. 1990 . “ Phase Transitions and Thermal Expansion Coefficients of Plant Cuticles ” . In The Effects of Temperature on Structure and Function. Planta Vol. 182 , 186 – 193 .
- Matas , A.J. , Cuartero , J. and Heredia , A. 2004 . “ Phase Transitions in the Biopolyester Cutin Isolated from Tomato Fruit Cuticles ” . In Thermochimica Acta Vol. 409 , 0165 – 168 .
- Matas , A.J. , Lopez-Casado , G. , Cuartero , J. and Heredia , A. 2005 . Relative Humidity and Temperature Modify the Mechanical Properties of Isolated Tomato Fruit Cuticles . Amer. J. Botany , 92 ( 3 ) : 462 – 468 .
- Taylor , G.B. 1996 . Incidence and Measurement of Autumn Seed Softening within Medicago polymorphia . L. Australian J. Agric. Res , 47 : 575 – 596 .
- Hoseney , R.C. 1994 . “ Starch. In Principles of Cereal Science and Technology. ” . In American Association of Cereal Chemists: St. Paul, MN 29 – 64 .
- Bernal-Lugo , I. , Parra , C. , Portilla , M. , Pena-Valdiva , C.B. and Moreno , E. 1997 . “ Cotyledon Thermal Behavior and Pectic Solubility as Related to Cooking Quality in Common Beans ” . In Plant Foods for Human Nutrition Vol. 50 , 141 – 150 .
- Biliaderis , C.G. , Maurice , T.J. and Vose , J.R. 1980 . Starch Gelatinization Phenomena Studied by Differential Scanning Calorimetry . J. Food Sci , 45 ( 6 ) : 1669 – 1674 . 1680.
- Zhang , H. , Ishida , N. and Isobe , S. 2004 . High-pressure Hydration Treatment for Soybean Processing . Trans. ASAE , 47 ( 4 ) : 1151 – 1158 .