Abstract
The effects of pH, dose, and temperature on the superficial charge density of fining agents were determined in a model solution. This study included three gelatines and one egg's albumin, all of which are common fining agents, as well as three wheat glutens (one native and two hydrolyzed). Wheat glutens have been allowed to be used as fining agents only since 2005, and there are no preliminary studies examining them. The results of this study showed that the superficial charge density was mainly pH-dependent (p < 0.01), and that pH showed a greater effect at a higher degree of protein hydrolysis. For Gel 3, albumin and the wheat glutens, a larger protein concentration, decreased the superficial charge density. Gel 2, which was soluble in hot water, had the highest superficial charge density (>0.831 meq g−1), which indicated that it would be more reactive than the other fining agents. Between pH 3.4 and 4.0, Gluten 1 and Gel 3 were more highly charged (superficial charge density >0.3 meq g−1) than Gel 1 or the hydrolysed glutens. These data are useful for wine producers.
INTRODUCTION
The process of fining, or using proteins to clarify wine, seeks to reduce wine turbidity and guarantee wine stability over time. Theories about the mechanism of fining are based on charge phenomena. Rüdiger and Mayr[Citation1] explained wine fining as a process of charge and discharge between colloidal particles. At the same time, Ribéreau-Gayon[Citation2] observed that flocculation was the result of tannin-protein interactions. Tannins transform gelatine, a hydrophilic colloid with a positive charge, into a hydrophobic colloid with a negative charge. The composition of the colloid, which is precipitated by the presence of metallic cations, is affected by factors, such as pH and temperature. Proteins, therefore, play an important role when they interact with tannins and reduce the colloidal state of wine, but they also show complex behaviour in wine.
Salgues and Razungles[Citation3] examined the role of chemical bond forces and reactions of attraction, repulsion, hydration, and dehydration in the fining process. They also considered the reversibility or non-reversibility of fining stages. Research based on superficial charge density[Citation4,Citation5] showed that fining is not a simple discharge between tannins and proteins, as determined by Rüdiger and Mayr.[1] Gelatine behaves as a positive polyelectrolyte and its interactions with tannins are mainly due to hydrogen bonds and hydrophobic forces. Previously, Oh et al.,[Citation6] Hagerman and Butler,[Citation7] and McManus et al.[Citation8] observed that hydrophobic forces are quite important in tannin-protein interactions. Moreover, this chemical force has a cooperative effect on polyphenol–protein interactions.[Citation9,Citation10] Lagune[4] observed that wine components bind to surface proteins at different linkage sites until a mono-coat is formed. According to studies of the physico-chemical behaviour of polyphenols and proteins,[9] equilibriums between the different species are established as a function of time and the nature of the substances involved (the fining agents, polyphenols, etc.). Once the interaction occurs, insoluble particles are formed. Charlton et al.[Citation11] showed that the distribution of particle size depends on the concentration of polyphenols and the conditions of the medium (such as pH and temperature). The pH-dependence suggests that surface charge plays an important role in determining the size of particles.
The charge of a substance in a medium is expressed by the superficial charge density (SCD). The SCD of gelatines is affected by the hydrolysis process.[Citation4,Citation12,Citation13] Lagune[Citation4] suggested that gelatines with a high net charge will precipitate more wine tannins. Several studies have found that fining agents with a high SCD are correlated with high turbidity reduction during the clarification process.[Citation12–15] These studies were carried out in gelatines and other animal proteins, but there are no studies for new fining agents. The aim of this study was to determine the effect of pH, dose, and temperature conditions on variations in superficial charge density. Obtaining optimal values for the SCD of fining agents can help wineries gain more control over the clarification process. This work included various clarifying agents, like gelatines and albumin, that are normally used in wineries, as well as wheat glutens, which have been allowed to be used as fining agents since 2005.[Citation16] The response surface plot constructed from equations obtained by multiple regression analysis allows us to determine the optimal region for the use of each substance.
MATERIAL AND METHODS
Fining Agents
Seven proteins () were analyzed: an atomized gelatine (Gel 1) soluble in cold water (ViniGEL AT, Agrovin, Navarret, Spain), a powdered gelatine (Gel 2) soluble in hot water (ViniGEL GR, Agrovin, Navarret, Spain), a liquid gelatine (Gel 3) (Colle H, Az3oeno, Navarret, Spain), a pasteurized liquid ovoalbumin (albumin) (Adagio, Az3oeno, Navarret, Spain), a native wheat gluten (Gluten 1) supplied by Huici-Leidan (Navarret, Spain), a wheat gluten obtained from protein heat hydrolysis (Gluten 2) (devital wheat gluten, Roquette-Laisa, Navarret, Spain), and an enzymatically hydrolyzed wheat gluten (Gluten 3) (GBS-P51, Chamtor, Bazancourt, France).
Table 1 Characteristics of fining agents
Model Wine Solution
To measure the isoelectric point and superficial charge density, a model wine solution was prepared by diluting 5 g of tartaric acid (Sigma, St. Louis, MO, USA) and 130 ml of absolute ethanol (Merck, Damstadt, Germany) in up to 1 litre of deionised water. In case of superficial charge density, the pH of the model wine solution was adjusted with solutions of 1 mol l−1 HCl/NaOH. The solution was prepared just before the tests.
Determination of Isoelectric Point (Ip)
The protein isoelectric points were determined by titration using a particle charge detector (Mütek PCD 03 pH, BTG, Herrsching, Germany). Proteins were dissolved in a model wine solution at 20 g hl−1 and 15 ml of solution were used to perform the measurement. Titration was carried out with a 0.1 mol l−1 HCl/NaOH solution (depending on the solution's cationic or anionic character) until the streaming potential reached 0 mV. All measurements were made in triplicate at a temperature of 20°C. Data were analysed by one-way analysis of variance (ANOVA). If the ANOVA determined statistical differences, a Tukey's significant multiple test (α = 0.01) was performed.
Determination of Superficial Charge Density (SCD)
Superficial charge density (meq g−1) was measured using the PCD 03 pH particle charge detector (BTG, Herrsching, Germany) according to the Lagune method.[4] A volume of 20 ml was titrated by an automatic compact titrator (Crison, Alella, Spain) using the solutions polydiallyldimethyl ammonium chloride (polyDADMAC) and potassium polyvinyl sulfonic acid (PESNa). All measurements were made in triplicate.
Experimental Design and Statistical Analysis
A complete block statistical design with three independent variables (pH, dose, and temperature) was applied. Six levels of pH were measured, ranging from 2.5 to 4.0 in 0.3 unit intervals. The protein concentration range was the customary one used in the fining process with gelatine and egg's albumin; the three levels examined were 8, 12, and 20 g hl−1. Temperature conditions were analyzed at five levels (5, 10, 15, 20, and 25°C). In the case of Gel 1, the complete research design was carried out. For Gluten 1, the effect of pH and temperature was measured at protein concentrations of 8 and 12 g hl−1. For Gel 2, Gel 3, albumin, and the hydrolyzed wheat glutens, the statistical design focused on the influence of pH and dose at 20°C. All of the measurements were done in triplicate.
Multiple regression analyses were used to determine the influence of pH, dose, and temperature on SCD. The analysis was performed with the aid of the Statgraphics® Statistical software package (Statgraphics Plus 5.1, Statistical Graphic Corp., Cincinnati, OH, USA). A stepwise regression was used to choose the best fitting model. Before the test, the residual standard deviation was used to delete outlier values. To obtain an adequate mathematical equation for superficial charge density, the maximum linear and quadratic effects were considered. EquationEquation 1 expresses all the possible terms:
Y is the response (i.e., the SCD of the analysed protein). β0, β i , and β ii are regression coefficients for intercept, linear, and quadratic terms, respectively. xi and xj are uncoded independent variables (pH, dose, and temperature). The coefficient of determination (R 2) indicates the percentage of variation or the optimization parameter that is explained by the model, and the adjusted R2 indicates its predictive ability. Stepwise multiple regression analysis selects predictor variables when their inclusion in the model exceeds the entrance threshold of an F-test (Fin ). This procedure takes into account the inclusion of a new predictor variable that modifies the significance of the predictors that are already present in the model. Therefore, a rejection threshold Fout is used to determine whether one of the previous predictors should be removed from the model.
RESULTS AND DISCUSSION
Isoelectric Point
shows the isoelectric points of the seven proteins that were analyzed in a model wine solution. A model wine solution was used because this characteristic of proteins is medium-dependent.[Citation17] All of the proteins that were tested presented an Ip above the pH range that was studied (pH 2.5–4.0). In the pH range of the model wine solutions (pH 2.5– 4.0), the gelatines, albumin, and glutens presented an always-positive charge.
Table 2 Isoelectric points of the proteins analyzed
Gel 1 presented an Ip that was closer to pH 4.0, which corresponds to the Ip range of gelatines obtained from an alkaline manufacturing process.[Citation18] Although Gel 2 presented an Ip above pH 6.0, this protein is an enzymatic hydrolyzed and was obtained from an alkaline process.[Citation19] Gel 3 presented an Ip that was within the Ip range of gelatines that are obtained from an acid process and that are soluble in hot water.[18]
Albumin presented an isoelectric point (4.7 ± 0.1) that corresponds to the Ip of pure egg albumin.[17] Statistical analysis showed that its isoelectrical point is ranged in the same values as gelatine Gel 1. The wheat glutens had isoelectric point values that ranged from pH 5.8 to 6.4. Although Gluten 2 and Gluten 3 were hydrolyzed, their isoelectric points were close to that of Gluten 1, which was native.
Superficial Charge Density (SCD) and Regression Analysis
Gelatines
Gel 1
In the pH range that was studied, Gel 1 presented a superficial charge density of 0.025 to 0.402 meq g−1; these values correspond to the usual values for cold-water-soluble gelatines that were obtained by enzymatic hydrolysis.[4, Citation12,Citation14,Citation15,Citation20,Citation21] After 270 trials were run according to the experimental design and the superficial charge density of Gel 1 was determined, a stepwise multiple regression analysis was applied to the results. The equation obtained for the superficial charge density of Gel 1 () explained 93.9% (R 2) of the variability. shows the response surface plots which were obtained from the model equation and which represent the SCD as a function of the studied variables.
Table 3 Fitted equation for the different proteins obtained by stepwise multiple regression
Figure 1 Response surface plot for superficial charge density (meq g−1) of Gel 1 as function of pH, temperature (°C), and dose (g hl−1): (a) 20°C; (b) dose 12 g hl−1; (c) pH 3.4.
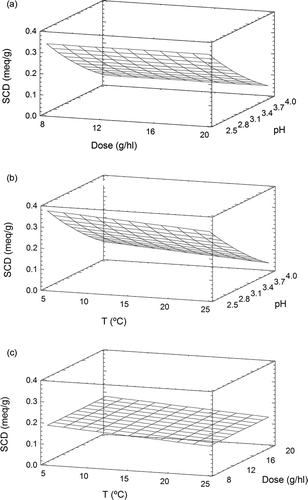
Gel 1 presented a maximum SCD value (0.374 meq g−1) at pH 2.5 and 5°C and presented a minimum SCD value at the same temperature and pH 4.0. Dose did not influence the superficial charge density. The SCD of Gel 1 decreased as it approached the Ip, since protein net charges are weak and protein molecules produce minimum interactions with water.[17] In the pH range that was studied, the loss of superficial charge density of Gel 1 varied from 0.242 to 0.308 meq g−1. However, the superficial charge density of Gel 1 decreased slightly as the temperature increased from 5 to 25°C. This observation corresponds to the findings of Ferrarini et al.[12]
Gel 2
Gel 2 presented SCD values that ranged from 0.831 to 1.272 meq g−1; these values correspond to the usual values for hot-water-soluble gelatines.[4,12,18,20,21] The results from 54 trials determining the superficial charge density of Gel 2 (at 20°C) were analysed. shows the developed model with the fitted equation at 56.2% goodness of fit.
The response surface plot () indicated that the SCD (1.085 meq g−1) was highest at pH 3.0 and fell at lower pH values. The charge loss of Gel 2 between pH 3.1 and 4.0 varied from 0.181 to 0.211 meq g−1. Although the polynomial equation that was obtained indicates that the SCD of Gel 2 is influenced by pH, this variable was not explanatory enough and additional factors also affect the variability of the superficial charge density in this pH range.
Gel 3
Gel 3 presented SCD values that ranged from 0.314 to 0.907 meq g−1; these values correspond to the usual values for gelatines manufactured by an enzymatic process.[4,18,20] Gel 3 is less hydrolyzed than the other gelatines and, therefore, had SCD values that were higher than those of Gel 1. The stepwise regression adjusted the fitted model that is indicated in and presented an R 2 of 0.913.
The response surface plot of superficial charge density for Gel 3 is shown in The maximum SCD value (0.765 meq g−1) occurred at pH 2.5 and dose 8 g hl−1. For Gel 3, the loss of superficial charge density as pH decreased (from 0.304 to 0.412 meq g−1) was less pronounced than in Gel 1 but higher than in Gel 2. At the same time, the superficial charge density of Gel 3 varied very little as the protein concentration increased.
The results from the three gelatines indicate that the manufacturing process significantly influenced the superficial charge density and its variation with respect to pH; this observation corresponds to the findings of other researchers.[4,13,20] The pH influenced the superficial charge density because it is dependent on the isoelectric point. Lagune[4] also observed that while the SCD of less-hydrolyzed gelatines decreased by 12% when the pH ranged from 2.2 to 3.8, the SCD values of more-hydrolyzed gelatines decreased by 30%. In this study, the SCDs of Gel 1 and Gel 3, both hydrolyzed gelatines, decreased from 85 to 46% when the pH ranged from 2.5 to 4.0. The SCD of Gel 2, which had an isoelectric point that was close to that of Gel 3 (Gel 2: pH 6.6 ± 0.2 and Gel 3: pH 6.20 ± 0.07), only decreased by 22%. Therefore, the distribution of molecular weights of gelatines seemed to influence their superficial charge density and their ability to lose a charge when close to their isoelectrical points. It was observed that lower molecular masses had the gelatines higher the variation of the charge density was. The SCD was slightly influenced by both protein concentration (as observed by Lagune[4]) and temperature (as observed by Ferrarini et al.[12]).
Gelatines that are soluble in cold water are pH-dependent; therefore, their charge varied in the pH range that was studied. Gel 3 presented an SCD value of 0.326 meq g−1 at pH 4.0, which was equivalent to Gel 1's charge (0.374 meq g−1) at pH 2.5. Meanwhile, the SCD of Gel 2 only varied by 0.05 meq in the pH range that was studied.
Albumin
The SCD value of albumin ranged from 0.118 to 0.268 meq g−1, as observed by Lagune-Ammirati and Glories[22] and Cosme et al.[15] shows the final model that was developed by a stepwise multiple regression; the fitted model had a coefficient of determination (R 2) of 92.3%. shows the response surface plot. The superficial charge density of this protein was also influenced by pH. Albumin had a maximum SCD value (0.231 meq g−1) at pH 2.5 and dose 8 g hl−1. This pH corresponds to the values obtained from gelatines that are soluble in cold water (Gel 1 and Gel 3). The superficial charge density of albumin varied slightly when the pH varied from 2.5 to 2.8. Otherwise, in a model solution with a pH ranging from 2.8 to 4.0, the superficial charge density decreased considerably in accordance with the findings of Lamadon et al.[20] and Lagune-Ammirati and Glories.[Citation22,Citation23] As the pH approached 4.0, the superficial charge density of albumin fell by 38%, from 0.095 to 0.063 meq g−1. This reduction in charge could be due to the pasteurization of hydrolyzed albumin. Albumin was also influenced by the protein concentration and, as observed in Gel 3, the maximum SCD value was at dose 8 g hl−1.
Figure 3 Response surface plot for superficial charge density (meq g−1) of albumin as function of pH and dose (g hl−1).
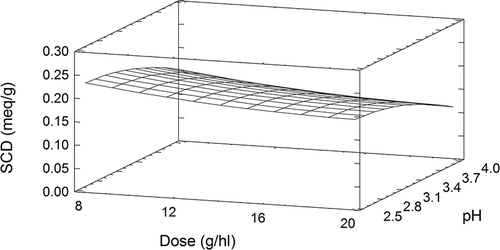
The SCD of ovoalbumin was similar to that of the enzymatically hydrolyzed Gel 1. Gelatines are commonly used in the fining of young red wines, while egg albumin, which tones down wines that are rich in tannins, is traditionally used to clarify aged red wines.[2] Fining assays showed that albumins from fresh eggs did not modify the total polyphenolic index and only reduced slightly reduced the tannin content.[22,23] These observations and the albumin's SCD values show the important relationship between a protein's SCD and its ability to reduce tannins.
Wheat glutens
Gluten 1
In the range of conditions studied, Gluten 1 presented a superficial charge density of 0.171 to 0.548 meq g−1; these values are close to those of Gel 1. The stepwise regression procedure selected the model shown in . The response surface plot of the fitted equation is shown in The graphic shows a maximum SCD value (0.477 meq g−1) at dose 9 g hl−1, pH 4.0, and 25°C. The influence of pH on superficial charge density was lower than it was for gelatines or ovoalbumin. An increase in protein concentration caused the SCD to decrease, as was observed for Gel 3 and albumin. The SCD may be reduced when the protein molecules are closed. On the other hand, an increase in temperature generated an increase in superficial charge density. In the case of Gluten 1, while the superficial charge density of the protein was influenced by all three of the variables studied (pH, dose, and temperature), these variables only explained 56.5% of the variation in SCD.
Gluten 2
The SCD of Gluten 2 ranged from 0.152 to 0.267 meq g−1; these values are similar to the range presented by the enzymatically hydrolyzed Gel 1. The final model represents the SCD of Gluten 2 as the second-degree equation indicated in . This mathematical expression explained 63.5% of the variation in superficial charge density. The response surface plot is shown in There was a maximum SCD (0.234 meq g−1) at pH 2.5 and dose 8 g hl−1, and the SCD decreased slightly as pH increased (from 0.07–0.10 meq g−1). As with other proteins (Gel 3, albumin, and Gluten 1), the augmentation of the protein concentration only slightly reduced the superficial charge density. In the conditions studied, Gluten 2 had lower and more variable SCD than Gluten 1. This fact can be explained by the hydrolysis process that yields polypeptides with lower molecular weights. This fact has been reported in previous studies carried out with gelatine.[13,15,20]
Gluten 3
In the pH range studied, Gluten 3 had SCD values of 0.137 to 0.305 meq g−1. These values were similar to those of albumin and the enzymatically hydrolyzed gelatines. The stepwise procedure showed that pH and dose contributed to 92.9% of the variation in charge; the resulting expression is shown in . The response surface plot of this expression is represented in The superficial charge of Gluten 3 was more influenced by pH than the SCD of the other wheat glutens. There was a maximum SCD value (0.277 meq g−1) at pH 2.5 and dose 8 g hl−1, as observed for Gluten 2. The loss of charge within the pH range that was studied was higher for Gluten 3 than for Gluten 2 (variance of 0.08–0.14 meq g−1). The plot shows that an increase in protein concentration decreased the SCD values.
The results showed that the charge values for wheat glutens were in the range of the SCD values for albumin and for gelatines that are soluble in cold water (Gel 1 and Gel 3). Among the glutens, devital glutens had a lower superficial charge density than vital glutens, which could be an effect of the hydrolysis process. The variation in charge with the increasing pH level was greater in the gluten that was obtained from enzymatic hydrolysis (Gluten 3, 42%) than in the gluten that was obtained from hot process hydrolysis (Gluten 2, 30%). Meanwhile, the SCD of Gluten 1 varied by no more than 10%. This finding suggests that pH had a greater influence as the degree of hydrolysis increased.
In contrast to Gel 1 and Gel 2, for Gel 3, albumin, and the wheat glutens, an increase in substance concentration reduced the superficial charge density. Substance concentration was particularly important for Gluten 1, since the augmentation of the dose resulted in a charge range of 0.4 to 0.2 meq g−1. This change could be due to the size and conformation of the protein. Temperature had a slight effect on the SCD of Gel 1, but had a marked and contrary effect on the SCD of Gluten 1. An increase in temperature from 5 to 25°C caused a 0.1 meq g−1 increase in the charge of Gluten 1.
shows that Gel 2 was the most charged (>0.837 meq g−1) fining agent in the range studied. Research relating superficial charge density with fining aspects have observed that highly charged gelatines produce high wine limpidity[13,15] and precipitate more wine components,[12,14] particularly tannins.[4] Thus, Gel 2 would be indicated for use in wines with high tannin content. Among the remaining fining agents, Gel 1 presented a higher charge (SCD between 0.25 and 0.50 meq g−1) in solutions from pH 2.5 to 3.2 than Gel 3, albumin, or the wheat glutens. From pH 3.4 to 4.0, the SCD of Gel 1 decreased and Gluten 1 and Gel 3 presented SCD values (>0.3 meq g−1) that were higher than those of Gel 1. Therefore, Gluten 1 and Gel 3 would be more reactive in wine with this pH range.
Table 4 Minimum and maximum values of SCD (meq g−1) of the fining agents in the range studied
Previous works[Citation24] showed that Gluten 3 generated a similar volume of lees as Gel 1 in young red wines (pH 3.7). Both are hydrolyzed proteins with a similar superficial charge density and distribution of molecular weight, so it could be expected that the SCD values would be similar. It was also observed that Gluten 1 (the native wheat gluten) generated less lees volume (by 50%) than Gel 1 or Gluten 3 with a comparable reduction of turbidity. However, in those conditions (pH 3.7), Gluten 1 presented an SCD value that was 0.20 meq g−1 more than that of either Gel 1 or Gluten 3, so a high reduction of turbidity would be expected. These observations indicate that other physico-chemical protein characteristics, such as size and flexibility of the protein substance, determine its interaction with tannins and its effectiveness in clarification.[Citation15,Citation25–28]
CONCLUSIONS
The SCD of the proteins was highly influenced by pH. The percentage of variation was higher for gelatines (lineal proteins) than for glutens and albumin (globular proteins). It was also observed that lower the molecular weights was higher the variation of SCD.
The influence of dose and temperature on SCD was slight in the range studied. For all the proteins studied, except for Gel 1 and Gel 2, SCD decreased when temperature or dose increased. Gluten 1 (native protein) was the more influenced protein by these two factors. Gel 2 and Gluten 1 were the proteins that showed the highest SCD so these proteins have been expected to be the more reactive with polyphenols.
REFERENCES
- Rüdiger , M. and Mayr , E. 1929 . Die Weinschonüng. Kolloid-Zeitschrift , 47 : 141 – 155 .
- Ribéreau-Gayon , P. , Glories , Y. , Maujean , A. and Dubourdieu , D. 2002 . “ Tratado de enología ” . In Tomo II. Química del vino. Estabilización y tratamientos , 584 Madrid , , Spain : Mundi Prensa .
- Salgues , M. and Razungles , A. 1983 . Le collage des vins. Vititech . Dec–Jan , : 32 – 35 .
- Lagune , L. 1994 . “ Thésis ” . In Etude des gelatines oenologiques et des mecanismes du collage dans les vins rouges , Universite de Bordeux II, Bordeux , , France : Ecole doctorale des sciences biologiques et medicales .
- Lagune-Ammirati , L. and Glories , Y. 1996 . Les nouvelles données concernant le collage des vins rouges avec les gélatines œnologiques . Revue des Oenologues. , 80 : 18 – 20 .
- Oh , H.I. , Hoff , J.E. , Armstrong , G.S. and Haff , L.A. 1980 . Hydrophobic interaction in tannin-protein complexes . Journal of Agricultural and Food Chemistry , 28 ( 2 ) : 394 – 398 .
- Hagerman , A.E. and Butler , L.G. 1980 . Condensed tannin purification and characterization of tannin-associated proteins . Journal of Agricultural and Food Chemistry , 28 : 947 – 952 .
- McManus , J.P. , Davis , K.G. , Beart , J.E. , Gaffney , S.H. , Lilley , T.H. and Haslam , E. 1985 . Polyphenol interactions. Part 1. Introduction: Some observations on the reversible complexation of polyphenols with proteins and polysaccharides . Journal of the Chemical Society Perkin Transactions , 2 : 1429 – 1438 .
- Haslam , E. and Lilley , T.H. 1988 . Natural astringency in foodstuffs: A molecular interpretation . Critical Reviews in Food Science and Nutrition , 27 ( 1 ) : 1 – 40 .
- Luck , G. , Liao , H. , Murray , N.J. , Grimmer , H.R. , Warminski , E.E. , Williamson , M.P. , Lilley , T.H. and Haslam , E. 1994 . Polyphenols, astringency and proline-rich proteins. Phytochemistry , 37 ( 2 ) : 357 – 371 .
- Charlton , A.J. , Baxter , N.J. , Lilley , T.H. , Haslam , E. , McDonald , C.J. and Williamson , M. P. 1996 . Tannin interactions with a full-length human salivary proline-rich protein display a stronger affinity than with single proline-rich repeats . Journal of Agricultural and Food Chemistry , 50 ( 6 ) : 289 – 292 .
- Ferrarini , R. , Celotti , E. and Zironi , R. 1996 . Importance des charges électriques superficielles des adjuvants œnologiques, des particules et des colloïdes présents dans les moûts et les vins . Revue Française d‘Oenologie , 158 : 5 – 10 .
- Poinsaut , P. and Scotti , B. 1998 . La chiarificazione dei vini con gelatina: Correlazione tra scienza e tradizione . L'Enotecnico , 34 ( 1/2 ) : 89 – 96 .
- Versari , A. , Barbanti , D. , Potentini , G. , Parpinello , G.P. and Galassi , S. 1999 . Preliminary study on the interaction of gelatin-red wine components . Italian Journal of Food Science , 11 ( 3 ) : 231 – 239 .
- Cosme , F. , Ricardo-Da-Silva , J.M. and Laureano , O. 2007 . Protein fining agents: Characterization and red wine fining assays . Italian Journal of Food Science , 19 ( 1 ) : 39 – 56 .
- European Council Regulation (EC) . 2005 . No. 2165/2005 of 20 December 2005 amending Regulation (EC) No. 1493/1999 on the common organisation of the market in wine . Official Journal of the European Union , 28 of December
- Cheftel , J.C. , Cuq , J.L. and Lorient , D. 1989 . Proteínas Alimentarias. Bioquímica. Propiedades Funcionales. Valor Nutritivo. Modificaciones Químicas , 364 Zaragoza , , Spain : Acribia Press .
- Paetzold , M. and Glories , Y. 1991 . Étude de gélatines utilisées en oenologie par mesure de leur charge macromoléculaire . Journal International des Sciences de la Vigne et du Vin , 24 ( 2 ) : 79 – 96 .
- Schrieber , R. and Gareis , H. 2007 . Gelatine Handbook. Theory and Industrial Practice , 334 Weinheim , , Germany : Wiley-VCH Verlag GmbH & Co. KGaA, Press .
- Lamadon , F. , Bastide , H. , Lecourt , X. and Brand , E. 1997 . Acquisitions récentes sur le collage des boissons, enjeux et perspectives, aspects pratiques . Revue Française d'Oenologie , 165 : 33 – 43 .
- Versari , A. , Barbanti , D. , Potentini , G. , Mannazzu , I. , Salvucci , A. and Galassi , S. 1998 . Physico-chemical characteristics of some oenological gelatins and their action on selected red wine components . Journal of the Science of Food and Agriculture , 78 ( 2 ) : 245 – 250 .
- Lagune-Ammirati , L. and Glories , Y. 2001 . Produits de clarification, une alternative rapidement exploitable: Les dérivés de l‘albumine d’œuf . Revue Française d'Oenologie , 191 : 25 – 33 .
- Lagune-Ammirati , L. and Glories , Y. 2002 . Les dérivés de l´albumine d´oeuf: Les essais en cave . Revue Française d'Oenologie , 194 : 18 – 24 .
- Durán , D. 2004 . “ Thesis ” . In Estudio de las proteínas de origen no animal como clarificantes enológicos en vinos tintos de Navarra , Universidad Pública de Navarra, Pamplona , , Spain : Departamento Química Aplicada .
- Ricardo-Da-Silva , J.M. , Cheynier , V. , Souquet , J.M. , Moutounet , M. , Cabanis , J.C. and Bourzeix , M. 1991 . Interaction of grape seed procyanidins with various proteins in relation to wine fining . Journal of the Science of Food and Agriculture , 57 : 111 – 125 .
- Sarni-Manchado , P. , Cheynier , V. and Moutounet , M. 1999 . Interactions of grape seed tannins with salivary proteins . Journal of Agricultural and Food Chemistry , 47 ( 1 ) : 42 – 47 .
- Braga , A. , Cosme , F. , Ricardo-da-Silva , J.M. and Laureano , O. 2007 . Gelatine, casein and potassium caseinate as distinct wine fining agents: Different effects on colour, phenolic compounds and sensory characteristics . Journal International des Sciences de la Vigne et du Vin , 41 ( 4 ) : 203 – 214 .
- Cosme , F. , Ricardo-Da-Silva , J.M. and Laureano , O. 2009 . Effect of various proteins on different molecular weight proanthocyanidin fractions of red wine during wine fining . American Journal of Enology and Viticulture , 60 ( 1 ) : 74 – 81 .