Abstract
The diffusion process has many applications in food processing operations, such as extraction; preservation (curing, salting, and smoking); drying; and color transfer. Our main objective was to measure the diffusion coefficient, Ds, of salt into vegetable tissue (celery, mushroom, and water chestnut) as a function of temperature. For this purpose, a sample of tissue was used to separate donor and receiver compartments, each with solutions of different salt concentrations. Values of Ds were determined by periodic monitoring. The results show that the equilibrium distribution coefficient, K, and Ds of all materials were significantly affected by temperature (p < 0.05). The temperature dependence of Ds for all three materials was described by the Arrhenius equation, with water chestnut showing the lowest activation energy.
INTRODUCTION
Diffusion can either involve leaching, where the solute migrates from the solid to the liquid, or infusion, where the solute migrates from the liquid into the solid. The diffusion process controls the rate at which the solute is either absorbed or released. Several studies have addressed the salt diffusion process in pork tissue,[Citation1–3 beef muscle,[Citation2] green olives,[Citation4] potato,[Citation5] white potato,[Citation6,Citation7] Atlantic salmon,[Citation8] chicken breast,[Citation9] and water chestnut.[Citation10]
Most salt diffusion studies have been conducted below 50°C. Only two salt diffusion studies involved higher temperatures: that by Liu[Citation5] for potato from 50–120°C, and by Sarang and Sastry[Citation10] for water chestnut from 25–80°C. Limited salt diffusivity data are available for food materials. Accordingly, our objective here was to measure Ds of salt in celery, mushroom, and water chestnut over a range of temperatures, from 25–80°C. This information is useful in marination and blanching processes as a pre-processing step prior to cooking or electrothermal processing.[Citation11,Citation12]
MATERIALS AND METHODS
The equilibrium distribution coefficient, K, and the diffusion coefficients, Ds , of salt into vegetable tissue were determined at three temperatures (25, 50, and 80°C). The vegetable tissues were Chinese water chestnut (Trapa natans), pascal celery (Apium graveolens var. dulce), and white button mushroom (Agaricus bisporus). The celery and mushroom were purchased fresh from the grocery store. The cross section of a Pascal celery stalk was used for the experiments. The stem of white button mushroom was removed and the cap was used for the experiments. Because canned water chestnut still has the same cell structure as fresh water chestnut,[Citation13] cans of sliced water chestnut were used. A detailed methodology follows.
Equilibrium Distribution Coefficient (K)
The samples were prepared using a slicer and a set of cork borers. Sample dimensions are listed in . A single slice of sample was placed in a beaker containing 100 ml of 5% salt solution. The temperature of each experiment was controlled within ±2°C of the pre-assigned temperature of 25, 50, or 80°C by placing the beakers inside a water bath. The beakers were sealed with aluminum foil to prevent vaporization of the solvent. At fixed time intervals, one beaker was picked randomly and removed from the water bath. The sample was removed and crushed with distilled water. The sodium chloride concentration of the solution and the sample was determined separately by Mohr titration.[Citation14,Citation15] All experiments were done in triplicate. In summary, the experiment consisted of one independent variable (temperature) tested at three levels, with three replicates. The constant factor was salt concentration.
Table 1 Dimension of samples used to determine equilibrium distribution coefficient (K) and diffusion coefficient (D s )
Equilibrium between the sample and the salt solution was assumed when no further change of the sodium chloride concentration was observed. The equilibrium distribution coefficient was determined from the following equation:
The equilibrium distribution coefficient (also referred to as a partition coefficient) is the ratio of concentrations of a solute between two different phases in equilibrium with each other.
Diffusion Coefficient (Ds )
One experimental variable was studied (temperature) at three levels: 25, 50, and 80°C. Salt concentration in the donor compartment was maintained constant at a single level 5%, while that in the receiver compartment began at 0.001% but of necessity rose through the experiment. Three replicates were conducted.
Cylindrical samples were prepared using a slicer and a set of cork borers. The diameter of the samples was chosen based on (1) the size of the sample holder and (2) the largest diameter of the sample that was possible for celery and mushroom. The dimensions of these samples are shown in . Samples were prepared from raw celery and canned water chestnut. However, mushroom samples needed pretreatment as described below.
Precut disk-shaped mushroom samples were blanched prior to the experiment at 100°C for 3 min with distilled water. This time was set from preliminary experiments, in which it was found that mushrooms did not shrink further after 3 min. After blanching, a cork borer was used to obtain the desired sample size. The final size of the blanched mushroom for the experiment was similar to that of celery.
Experimental device
The diffusivity cell, as shown in was made of Acrylic Plexiglas®, and consisted of donor and receiver compartments, with a capacity of 1250 ml each. These compartments were separated by a wall where the sample was held by a sample holder (, showing sample dimensions for celery and mushroom; for water chestnut, dimensions were slightly modified). To avoid evaporation, the cell was closed with a lid made of the same materials as the compartment. A mechanical stirrer, which consisted of a polypropylene stirring rod (Fisher Scientifics, Hayward, CA, USA) connected to a motor (Talboys Instruments Corp., Emerson, NJ, USA), was used to regularly stir the solution in each compartment. A thermocouple (Cleveland Electric Laboratories, Twinsburg, OH, USA) was inserted via an opening in the lid on the donor compartment to measure the temperature of the experiments. A water bath (Fisher Scientifics) was used to control the temperature of the experiments.
Experimental procedure
Two concentrations of salt solutions, 5% NaCl for the donor compartment and 0.001% NaCl for the receiver compartment, were preheated to the pre-assigned temperature. The 5% level was chosen based on a previous study of salt diffusion into water chestnut,[Citation10] which showed that the apparent diffusion coefficient was not significantly affected by salt concentration in the range of 5 to 10%. Each compartment was filled with 950 ml of the salt solution. The cell was checked for leakage prior to the experiment by filling the cell with distilled deionized water. The temperature of the solutions was controlled within ±2°C of the pre-assigned temperature by placing the diffusivity cell inside a water bath. The solutions were continuously stirred using a mechanical stirrer. Since the solutions in both compartments were well agitated, the concentration in each was assumed to be spatially uniform at any given time. At fixed time intervals, a 5-ml sample of the solution was withdrawn from the receiver compartment and was analyzed for its salt concentration. The sodium chloride concentration accumulated in the receiver compartment was determined by Mohr titration.[Citation14,Citation15] Since 5 ml of the solution was withdrawn from the receiver compartment at each sampling time, the receiver volume changes during the experiment. The maximum volume change for the length of the experiment (about 5%) was assumed to not be significant.
Mathematical model for diffusion
The sample was assumed to be of fixed thickness. The diffusion across the sample, which causes the concentration in the receiver compartment to change with time, can be described using Fick's second law of diffusion (EquationEq. 2; Crank[Citation16]). The sample was assumed to act as a barrier separating the donor and receiver compartments ().
If diffusion coefficient is assumed to be reasonably spatially (x-direction) constant over the experimental duration, EquationEq. (2) may be modified to:
The initial condition is
The boundary condition at the donor compartment is
Since the salt concentration in the receiver compartment increases throughout the experimental duration, the salt concentration therein was calculated using the following equation:
The governing equation was discretized through the finite difference method. The diffusion terms were discretized by second-order central differences. The temporal discretization (time derivative) was done with the second-order implicit Crank-Nicholson method. Convergence was assumed at each time step when the relative change in the solution at all spatial nodes from successive iterations was less than 10−5. The domain was based on a grid of 41 nodes, which was found sufficient to ensure a grid-independent solution (further refinement led to a change of less than 0.5%). All calculations were performed using MATLAB (The Mathworks, Inc., Natick, MA, USA). The least squares method (EquationEq. 7) was used to estimate the diffusion coefficient (Ds ) by minimizing the difference between the measured and the predicted salt concentration in the receiver compartment.
Determination of Temperature Effect
The effect of temperature on the diffusion coefficient was modeled as showing Arrhenius dependence (EquationEq. 8). The diffusion coefficient data at each temperature were plotted and fitted into a linearized form of the equation. The activation energy Ea was calculated from the slope of the line. The diffusion coefficient constant was determined from the line intercept.
Statistical Analysis
Data were analyzed using comparison of means (analysis of variance/ANOVA). An additional Tukey-Kramer HSD (honestly significant difference) test was done to evaluate further when the result of ANOVA test indicated that there was a significant difference among means. All statistical evaluations were done using JMP 6 (SAS, Inc., Cary, NC, USA).
RESULTS AND DISCUSSION
The equilibrium distribution coefficient (K) of salt in celery, mushroom, and water chestnut was significantly affected by temperature (p < 0.05) (). The K value of celery and mushroom decreased as the temperature increased. However, there was no discernible trend for the K value of water chestnut. As shown in , our K values at 25 and 80°C were similar to K values from the study by Sarang and Sastry.[Citation10] However, Sarang and Sastry did not observe any significant effect of temperature on the K values of water chestnut. Sarang and Sastry also used canned sliced water chestnut in their experiment. It is noted that although the authors' numbers show statistically significant differences, the differences are not of practical significance. Indeed, the differences observed in this study are due to lower sample-to-sample structural variability in the present set of experiments compared to those of Sarang and Sastry. While some residual salt would be expected in previously canned samples, the quantity of salt in the sample was small compared to the total diffusing quantity over the experimental duration.
Table 2 Equilibrium distribution coefficient (K) and diffusion coefficient (D s ) of sodium chloride in celery, mushroom, and water chestnut tissue at three temperatures
Table 3 Comparison of K and D s values between our study and the study by Sarang and Sastry.Citation[10]
The increase of salt concentration over time in the receiver compartment at various temperatures is presented for celery (), mushroom (), and water chestnut (). The effect of temperature increase in water chestnut is not as obvious as in celery and mushroom, with only a gradual increase with temperature. Data in confirm that the salt diffusion coefficient (Ds ) of all materials increases significantly as the temperature increases (p < 0.05). As expected from the salt concentration curves, the increase of Ds in celery and mushroom is much higher than water chestnut. Unlike celery and mushroom, water chestnut is more resistant to thermal softening; its structure has been reported to be sturdy and not easily degraded by heat.[Citation10,Citation13] The same phenomenon was observed in this study. Chinese water chestnut is thermally stable because of the cell wall polymers, which helps in cell to cell adhesion.[Citation13]
Figure 4 Changes in salt concentration of celery in the receiver over time. Error bars for data at 25°C are not visible on this scale.
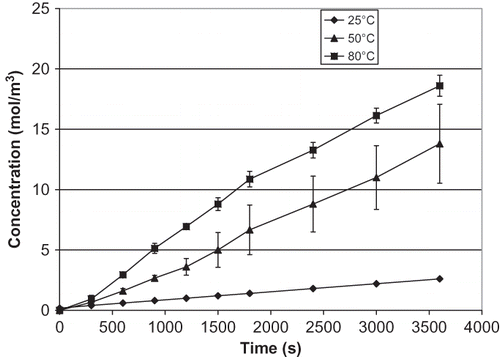
The trend of increasing Ds with increasing temperature has also been observed by Liu,[Citation5] who reported that diffusion coefficients data of salt in potato increased from 17.9 at 70°C to 44.5 × 10−10 m2/s at 120°C. Sarang and Sastry[Citation10] reported increasing Ds of salt into water chestnut with increasing temperature. Our values are somewhat lower, as shown in . Other than sample to sample variability, the difference might be due in part to the Mohr titration methodology used in both studies, where the titration end point is subject to operator judgment.
Table 4 Activation energy (E a ) and reference temperature constant (D o ) for salt diffusion in celery, mushroom, and water chestnut
The Ds for mushroom showed the greatest standard deviation of all samples. This may be due to variations within parts of the mushroom cap used for the experiment. No literature Ds data are available for salt in mushroom, to permit comparison. The Ds value may vary from one material to the other because of variations in cell characteristics. Water chestnut cells are more tightly packed than celery.[Citation13] This might cause greater resistance to the diffusion process, resulting in lower Ds values of water chestnut.
The increase of Ds with temperature is caused in part by structural changes of the tissue. As the vegetable tissue is heated, structural changes will increase the ionic mobility.[Citation17–19 Structural changes of the tissue may be caused either by cell wall or cell membrane breakdown. The cell wall breaks down in the 60–75°C temperature range,[Citation20] meanwhile cell membrane destruction happens in the 50–70°C temperature range.[Citation21] The main known function of the cell wall is to provide structural support, while the main function of the cell membrane is to regulate the movement of water and solutes between the cells. The intact cell wall and the cell membrane are the major resistance for the diffusion process.[Citation17,Citation22] With the destruction of either cell wall or cell membrane, there will be less resistance to the diffusion process.[Citation23] As the cell structure changes, the cell is decompartmented and the solutes diffuse freely to all parts of the tissue and the open intercellular spaces.[Citation17,Citation24] Without the presence of its structure, there is no restriction for the solute to pass through the tissue. This will allow for a faster diffusion process, which is reflected in the increase of the Ds values.
To further investigate the trend for increasing Ds with temperature, we took microscopic images (light microscopy) of raw and treated samples of celery and water chestnut at 80°C. We chose this condition because for all materials, the Ds at 25°C was significantly different from 80°C (). Microscopic images of raw and blanched mushrooms did not exhibit a clear cell structure, making examination difficult, thus no images could be presented.
Microscopic images of untreated (canned water chestnut) and treated (3 h at 80°C) water chestnut are shown in . The image of raw water chestnut () shows the closely packed, thin-walled parenchyma cells, which contain starch.[Citation13] The cells in water chestnut are not easily separated from one another, even by heat treatment from the canning process.[Citation13] The image of the treated water chestnut at 80°C () indicated a possibility of some changes in cell structure. The cells had slightly different characteristics compared to the raw sample, appearing to be less defined, and a fuzzy, less defined appearance of cell contents. This could be an indication of cell membrane breakdown, suggesting how the Ds values may have increased with temperature.
Figure 7 Microscopic images of water chestnut: (a) untreated sample and (b) treated sample heated at 80°C. (Color figure available online.)
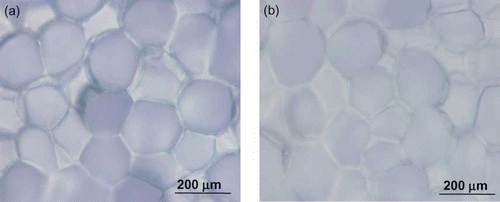
Microscopic images of raw and treated (80°C for 1 h) celery are shown in . The cell structure of raw celery () is clearly defined. The 80°C treated celery cells () did not have a clear structure and exhibited considerable overlap between adjacent cells. A study by Aguilera and Stanley[Citation17] on the microscopic structure of raw and cooked potatoes also showed similar findings: potato cells appeared to have less structure after cooking. The microscopic image difference between the raw and treated sample in celery was more obvious than the difference in water chestnut samples, and indicate that the heating treatment might affect the cell structure, enhancing diffusivity.
Figure 8 Microscopic images of celery: (a) raw sample and (b) sample heated at 80°C. (Color figure available online.)
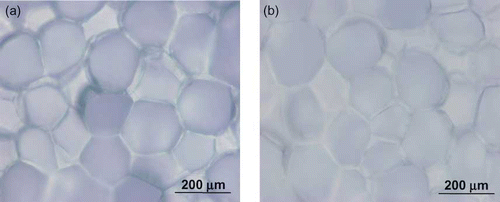
Diffusivity-temperature data were fitted to the Arrhenius equation, shown in the plot of . The results are tabulated in . As expected, water chestnut showed the lowest activation energy of the three tissues, showing relatively low temperature sensitivity. The authors' values for water chestnut (22 kJ/mol) appeared higher than those of Sarang and Sastry[Citation10] (12.7 kJ/mol), also plotted in . However, a statistical test (plotting the differences between data sets versus temperature, regression of the resulting data, and the test for the null hypothesis that the slope was zero) showed that the slopes of this work and those of Sarang and Sastry were not significantly different. The authors recalculated the activation energy of the combined data set of this study and those of Sarang and Sastry. These results are also shown tabulated in . This updated value would be expected to better reflect sample to sample variation. The values of coefficient determination, r 2 of celery (0.8381) and mushroom (0.8949) were lower than for water chestnut (0.9867). It is noted that both celery and mushrooms were obtained fresh, while water chestnuts were canned. However, as noted previously, the salt content of a sample is small compared to the overall diffusion over the experimental duration.
CONCLUSIONS
The equilibrium salt distribution coefficient (K) and salt diffusion coefficient (Ds ) in celery, mushroom, and water chestnut were significantly affected by temperature. K in celery and mushroom decreased as the temperature increased. There was no specific trend observed for water chestnut. Values of Ds for celery, mushroom, and water chestnut increased as the temperature increased. As the temperature increased, the increase of Ds in celery and mushroom was larger compared to the increase of Ds in water chestnut. Microscopic images of the raw and treated celery and water chestnut indicated that the heat treatment caused slight changes in the cell structure of the samples. This showed that the increase of temperature over time might compromise the cell structure by degrading the compartments within the cell, which would enable the solute to diffuse through the sample faster. The temperature dependence of Ds for all materials was described by the Arrhenius equation, with water chestnut showing the lowest activation energy of the three tissues.
NOMENCLATURE
A | = |
Cross sectional area of sample (m2) |
C | = |
Concentration |
Do | = |
Reference value of diffusion coefficient (m2/s) |
Ds | = |
Diffusion coefficient of salt through tissue (m2/s) |
Ea | = |
Activation energy (J/kg) |
K | = |
Equilibrium distribution coefficient |
l | = |
Thickness of sample (m) |
M | = |
Predicted salt concentration in the receiver compartment at fixed time interval (mol m−3) |
R | = |
Universal gas constant J/kg °K |
S | = |
Sum of squares of errors |
t | = |
Time (s) |
T | = |
Temperature (°K) |
Vr | = |
Volume of receiver compartment (m3) |
x | = |
Coordinate direction |
= |
Subscripts/Superscripts | |
d | = |
Donor |
i | = |
Node index |
m | = |
Sample |
∞ | = |
Value at equilibrium |
ACKNOWLEDGMENTS
Support provided by the USDA National Integrated Food Safety Initiative Grant No. 2003-51110-02093, titled “Safety of foods processed using four alternative processing technologies,” is gratefully acknowledged. Salaries and research support was provided in part by the Ohio Agricultural Research and Development Center, through the Regional Research Project NC-1023. References to products and trade names are made with the understanding that no endorsement or discrimination by The Ohio State University is implied.
REFERENCES
- Wood , F.W. 1966 . The diffusion of salt in pork muscle and fat tissue . Journal of the Science of Food and Agriculture , 17 : 138 – 140 .
- Dussap , G. and Gros , J.B. 1980 . “ Diffusion-sorption model for the penetration of salt in pork and beef muscle ” . In Food Process Engineering , Edited by: Linko , P. , Malkki , Y. , Olkku , J. and Laringkari , J. 407 – 411 . London : Applied Science Publishers .
- Graiver , N. , Pinotti , A. , Califano , A. and Zaritzky , N. 2006 . Diffusion of sodium chloride in pork tissue . Journal of Food Engineering , 77 : 910 – 918 .
- Drusas , A. and Vagenas , G.K. 1988 . Diffusion of sodium chloride in green olives . Journal of Food Engineering , 7 : 211 – 222 .
- Liu , H. 1992 . A kinetic study of salt diffusion in potato at high temperature . International Journal of Food Science and Technology , 27 : 443 – 455 .
- Wang , W.-C. and Sastry , S.K. 1993 . Salt diffusion into vegetable tissue as a pretreatment for ohmic heating: Electrical conductivity profiles and vacuum infusion studies . Journal of Food Engineering , 20 : 299 – 309 .
- Wang , W.-C. and Sastry , S.K. 1993 . Salt diffusion into vegetable tissue as a pretreatment for ohmic heating: Determination of parameters and mathematical model verification . Journal of Food Engineering , 20 : 311 – 323 .
- Wang , D. , Tang , J. and Correia , L.R. 2000 . Salt diffusivities and salt diffusion in farmed atlantic salmon muscle as influenced by rigor mortis . Journal of Food Engineering , 43 : 115 – 123 .
- Volpato , G. , Michielin , E.M.Z. , Ferreira , S.R.S. and Petrus , S.R.S. 2007 . Kinetics of the diffusion of sodium chloride in chicken breast . Journal of Food Engineering , 79 : 779 – 785 .
- Sarang , S. and Sastry , S.K. 2007 . Diffusion and equilibrium distribution coefficient of salt within vegetable tissue: Effects of salt concentration and temperature . Journal of Food Engineering , 82 : 377 – 382 .
- Tulsiyan , P. , Sarang , S. and Sastry , S.K. 2008 . Electrical conductivity of multicomponent systems during ohmic heating . International Journal of Food Properties , 11 ( 1 ) : 233 – 241 .
- Bozkurt , H. and Icier , F. 2009 . Rheological characteristics of quince nectar during ohmic heating . International Journal of Food Properties , 12 ( 4 ) : 844 – 859 .
- Parker , M.L. and Waldron , K.W. 1995 . Texture of Chinese water chestnut: Involvement of cell wall phenolics . Journal of the Science of Food and Agriculture , 68 ( 3 ) : 337 – 346 .
- Skoog , D.F. and West , D.M. 1976 . Fundamentals of Analytical Chemistry , 232 – 233 . New York : Holt, Rinehart, and Winston .
- Williams , W.J. 1979 . Handbook of Anion Determination , 297 – 301 . London : Butterworths .
- Crank , J. 1990 . The Mathematics of Diffusion , 2nd , Oxford : Clarendon .
- Aguilera , J.M. and Stanley , D.W. 1990 . Microstructural Principles of Food Processing and Engineering , Essex , , UK : Elsevier Applied Science .
- Wang , W. and Sastry , S.K. 1997 . Changes in electrical conductivity of selected vegetables during multiple thermal treatments . Journal of Food Process Engineering , 20 : 499 – 516 .
- Lebovka , N.I. , Praporscic , I. , Ghnimi , S. and Vorobiev , E. 2005 . Temperature enhanced electroporation under the pulsed electric field treatment of food tissue . Journal of Food Engineering , 69 : 177 – 184 .
- Andersson , A. , Gekas , V. , Lind , I. , Oliveira , F. and Öste , R. 1994 . Effect of preheating on potato texture . Critical Reviews in Food Science and Nutrition , 34 ( 3 ) : 229 – 251 .
- Praporscic , I. , Ghnimi , S. and Vorobiev , E. 2005 . Enhancement of pressing of sugar beet cuts by combined ohmic heating and pulsed electric field treatment . Journal of Food Processing and Preservation , 29 : 378 – 389 .
- Campbell , N.A. and Reece , J.B. 2003 . Biology , 6th , San Francisco : Benjamin Cummings .
- Schwartzberg , H.G. and Chao , R.Y. 1982 . Solute diffusivities in leaching process . Food Technology , 36 : 73 – 86 .
- Doulia, D.; Tzia, K.; Gekas, V. A knowledge base for the apparent mass diffusion coefficient (Deff) of foods. 1999 http://www.nelfood.com (http://www.nelfood.com)