Abstract
Second order phase transitions may occur in foodstuffs during the convective drying process. These transitions involve physicochemical changes, which influence both structural properties and drying behavior. The aim of this study was to examine the effects of drying air temperature and the second order phase transition of garlic on the changes in particle density, apparent density, apparent porosity, effective diffusivity, and cracking produced during drying. Garlic slices were dehydrated at three air temperatures (40, 50, and 60°C). The moisture content (X), inside temperature (Ti), surface temperature (Ts), apparent (ρb) and particle (ρp) densities of garlic slices were measured during drying. Porosity (ϵ) was calculated based on the data collected for ρp and ρb. Glass transition temperatures (Tg) and micrographs were obtained for both raw and dehydrated garlic. A critical point in the intersection of Ti, Ts, and Tg was found; this point was identified as a second order phase transition. Diffusivity and slope changes in ρb, ρp, and ϵ with respect to moisture content were found to be related to this critical point. Experimental data for ρb, ρp, and ϵ was fitted to a nonlinear equation with three exponential terms with respect to moisture content, with an R2 > 0.85. Less dense products were found to be more porous, with more cracking, higher moisture diffusivity, and lower Tg at the end of the drying process.
INTRODUCTION
New, unconventional drying methods, such as infrared-convective, convective-frying, convective-lyophilization, and non-isothermal[Citation1] drying processes have shown promising results for minimizing loss of quality in dried foodstuffs. Nevertheless, the effects of the most widely used drying method, convective drying, on the properties of foodstuffs are still not sufficiently understood. Before exploring new, alternative drying methods, it is necessary to fully understand the effects of the most common drying method on certain structural properties that are key in preserving the quality of dried foodstuffs and in minimizing deterioration both during and after processing.
During the drying process, water movement may occur through multiple mechanisms coupled with mass and heat transfers. The migration of water from the interior (sub-structures, micro-domains, and organelles) to the exposed surface of foodstuffs is accompanied by moisture gradients and stress. These gradients produce changes in the original characteristics of the product, such as shrinkage, collapse, case hardening, porosity development, and both internal and external cracking.[Citation2] Cellular rupture caused by unbalanced stress and moisture gradients can intensify enzymatic and non-enzymatic chemical reactions, forming undesirable or volatile compounds, and causing the loss of valuable components.
As garlic is a biological material highly sensitive to temperature changes, cellular structure is fragmented during the drying process, and vacuolar enzymes are released. These enzymes react with substances contained in the cytoplasm, forming volatile and bioactive compounds.[Citation3–7 Citation Citation Citation Citation7 From a technological perspective, it is very important to keep bioactive substances compartmentalized with a minimum amount of cell cracking during drying. In addition, loss of flavor intensity is exacerbated by a high drying temperature.[Citation8,Citation9] Changes in structural characteristics (particle density, apparent density, and porosity) have often been attributed exclusively to the effects of a change in moisture content.[Citation10,Citation11] Nevertheless, these effects do not sufficiently explain non-lineal density behavior at lower water contents. More recently, Aguilera and Stanley[Citation12] suggested that this behavior may be the result of the effects of micro structural changes, such as density and porosity, on macro structural quality.
Some authors have found that the particle density of garlic has a linear tendency with regard to water content,[Citation10,Citation11] and a relationship has been found between low density and a large quantity of pores formed during drying.[Citation13] Several equations have been proposed to describe the behavior of density during the drying process. Lozano et al.[Citation10] correlated the ρ b , and ρ p of garlic slices with moisture content as follows:
Madamba et al.[Citation11] used the equation below to describe the apparent density behavior of garlic slices with respect to moisture during the drying process:
The above-mentioned equations do not take into account changes in the chemical and biological properties of the material that may modify its density. In thermolabile biological materials, several reactions are set off by the drying process, including chemical reactions, cellular collapse, and first and second order phase transitions.[Citation12] As a result, structural property changes may occur.[Citation14–17 Citation Citation Citation17 During the drying process, the second order phase transition is dependent on the thermal history of the material[Citation18] and manifests itself as a change from a meta-stable, rubbery amorphous state to a glassy amorphous state; this occurs at a temperature denoted as the glass transition temperature (Tg ). However, it has not been clearly established if changes in apparent and particle density result from the transition.
The glass transition temperature (Tg ) of a product can be used as a reference in order to modify processing conditions and halt the drying process at the appropriate point in order to maintain the quality of the foodstuff. Tg increases with an increase in drying temperature, solids content, molecular weight, and removal of water during the drying process.[Citation14–19 Citation Citation Citation Citation19 Additionally, this property has been associated with porosity, as a large quantity of pores has been linked to a decrease in Tg .[Citation15,Citation17]
Molecular diffusion in foodstuffs is linked to molecular mobility. Independent of drying conditions (temperature, T; relative humidity, %HR; air velocity, v), the degree of mobility and the structure of the material influence water diffusivity and the compounds it transports. In many agricultural food products, diffusivity is the main mechanism for moisture transfer during the first part of the drying process; in materials in a glassy state, such as dried products, molecular movement is virtually nonexistent.[Citation20] Moisture diffusivity during drying is limited by the structure of the material and the possible state in which it is found (glassy or rubbery).
Currently, several methods are used to calculate effective diffusivity, including the permeation method, as well as drying and sorption kinetics.[Citation21] Effective diffusivity calculated from experimental data is a function of both the temperature and water content of the material. In order to calculate the deviation between theoretical diffusivity and experimental diffusivity, the slopes method, a simplified method used by Perry and Green,[Citation22] was used. A comparison of the slopes method with an optimization method (numerical simulation) produced by Kataranos et al.[Citation23] conclude that both methods produce similar data in materials where diffusion is predominant. The aim of this study was to analyze the effects of drying air temperature, moisture content, and the second order phase transition on changes in particle density, apparent density, apparent porosity, effective diffusivity, and cracking in garlic slices.
MATERIALS AND METHODS
Sample Preparation
For the sample, garlic (Allium sativum L.) of the purple, vermillion variety was obtained from a single lot provided by a grower in Calera Zacatecas, Mexico. Longitudinal slices with a thickness of 2.2 ± 0.2 mm were cut using an adjustable steel slicer. The samples were placed on four plastic meshes (25 cm × 25 cm), and then placed inside a convective tunnel dryer.[Citation24] Sliced garlic samples were removed from the dryer during the process for structural properties determination.
Tunnel Dryer
A tunnel dryer with temperature and air velocity control,[Citation24] heated by two electrical resistances of 20 Ω (2.4 kW) each, connected in parallel, was used for all tests. Air flow was provided by a centrifugal fan (A-SQ, Armee, Chicago, IL, USA). Air velocity was controlled by a frequency variator (SAMI MINISTAR SNKU-501A, ABB, Wickliff, OH, USA), and air temperature was regulated using a PID controller (Love Controls 16A3030, Dwyer Instruments, Michigan, IN, USA). Relative humidity (HR), air velocity (v), air temperature (Tdrying ), surface temperature (Ts ), inside temperature (Ti ), and weight loss were monitored in real time using a FieldPOINT® (National Instruments, TX, USA) data acquisition system. Data recording was performed once a minute using LabVIEW® software from National Instruments®. Temperatures Ti and Ts were measured with a semisolid implantable thermocouple 40-gauge (0.225 mm diameter), type T (A-08506-70, Cole Parmer, IL, USA). For Ti measurement, the thermocouple was implanted in a garlic slice that had been previously perforated with a needle (BDTM, 21G × 1½ in., regular bevel, O.D. of 0.81 mm). The perforation was carefully performed parallel to the longest axis in the center of the slice. For Ts measurement, the thermocouple was attached to the surface of the garlic slice using the natural adhesiveness of garlic and a circle (0.5 mm diameter) of aluminum tape to increase contact surface area. Three air temperatures (40, 50, and 60°C) were tested; the air velocity for each test was 1.5 m/s, and the flow was parallel to the largest exposed area of the garlic. Each drying trial and measurement was performed in triplicate. An ANOVA of the results was performed with α = 0.05 using NCSS 2003 software (NCSS, UT, USA).
Density and Porosity
Particle density (ρ p ) is defined as the mass contained in a given volume, excluding pores in the material:
Particle density was calculated using the particle volume and mass of the sample. Particle volume was measured with a stereopicnometer (SPY–5DC, Quantachrome, Boynton Beach, FL, USA) with the pressure inside the equipment set at 1.195 ± 0.003 kgf/m2. For mass measurements, samples of 30 ± 2 g were used. The apparent density of foodstuffs is considered to be ratio of the mass over the volume of the sample, including pores, and is defined as:
Apparent density (ρ b ) was measured in 1 ± 0.25 g of non-coated sample, using the volume displacement method with n-heptane[Citation25] and a density kit (YDK 01-OD, Sartorius Goettingen, Germany). Measurements were carried out within ≈10 s to prevent the immersion liquid from being absorbed. This variable was calculated with the following equation:
where ρ l was 680 kg/m3. Apparent porosity was calculated using the data for particle and apparent densities according to the definition of porosity as established by Zogzas et al.[Citation26] where air volume is considered to be the total volume of a material, as expressed by the following equation:
Fitting Experimental Data
The experimental data for particle density, apparent density, and porosity were fitted to a non-linear equation using NCSS 2003 software.
Microstructure
The microstructure of the material was observed from dried samples that had been stored in vacuum-sealed bags. The cellular structure of the samples was observed using a low-pressure vacuum-metric scanning electron microscope (JEOL JSM-6360 LV, Tokyo, Japan) operated at 15 kV in secondary electron mode. The samples were first vacuum-dehydrated and then coated with a 10-nm-thick layer of gold-palladium. No chemical fixation was used.
Effective Diffusivity
Effective diffusivity was calculated using the weight data obtained during drying from the LabVIEW® software. The slopes method (EquationEq. 10) was used. The experimental drying curve (W versus t) was compared with the theoretical drying curve (W versus Fo). The slopes of the experimental curve (dW/dt) exp and the theoretical curve (dW/Fo) are estimated for given non-dimensional moisture contents (W). For W, the weight data obtained from the drying process was used, and the final weight data was Xe . Diffusivity was calculated using the following equation:
where the Fourier number is:
The solution of this equation for a slice with constant diffusivity (D) in terms of an infinite series is given by Crank.[Citation27] In this study, the solution of EquationEq. (10) was employed, using the first term in the series:
Glass Transition Temperature (Tg )
The glass transition temperature was obtained using an MDSC (Q100, TA Instruments, New Castle, USA) and Universal Analysis 2000 4.1D software (TA Instruments). The samples were ground with a mortar and pestle, and 25.56 ± 2.91 mg was hermetically sealed in an aluminum pan. An empty pan was used as reference. The scanning range was from -50 to 100°C. The heating/cooling rate used in this study was 4°C/min with a modulation of ±1.0°C in amplitude. Thermograms of total, reversible, and non-reversible heat flow were analyzed. The glass transition temperature was identified as the midpoint between Tgonset and Tgendset in the reversible heat flow line. The Tg of the dried garlic samples was calculated using the Gordon and Taylor equation:[Citation28]
where kG-T is the Gordon-Taylor coefficient, and was calculated using the Simha-Boyer rule:[Citation29]
In the present study, water density (ρ
w
) and particle density (ρ
p
) were used to calculate ρ
s
using EquationEq. (4). The value of water was −135°C.
was calculated by iteration using experimental data (Tg
) and EquationEqs. (11) and Equation(12).
RESULTS AND DISCUSSION
Experimental data for particle density (ρ p ) and apparent density (ρ b ) with respect to moisture content are shown in and , and particle density, apparent density, and glass transition temperature for garlic samples at 0. 1 X/X0 are shown in . The initial moisture content of garlic (X 0) was 1.83 ± 0.21 kg/kg. Particle density varied linearly from 1214 to 1677 kg/m3 as X/X 0 decreased from 1.0 to 0.18. Subsequent to this value, a change in the linear tendency was observed with X/X 0 tending to 0.0 when ρ p falls from 1700 to 1100 kg/m3, depending on the drying air temperature. Apparent density varied linearly from 1045 to 1345 kg/m3, as X/X 0 decreased from 1.0 to close to 0.1. Subsequently, ρ b had a change in tendency, falling from 1345 to 1150 kg/m3 as X/X 0 decreased from 0.1 to close to 0.0.
Considering that density is a function of volume (EquationEqs. 4 and Equation5), the concepts employed in studies of shrinkage (V/V 0) were used to explain the behavior of density during the drying process. It has been suggested that a decrease in volume is proportional to water loss[Citation10,Citation11] and has a linear behavior with respect to moisture content. The non-linear segment may be explained by the fact that a reduction in volume is not equivalent to water loss due to the formation of a rigid surface that limits moisture transference to surrounding areas, particularly during the final stage of the drying process. If this is the case, then the tendency of density is non-linear with respect to moisture content.Citation[15, Citation26, Citation30, Citation31] However, this non-linear behavior has also been explained as a result of pores closing during the final stage of drying.[Citation10] In that case, the solid volume considered in the calculation of density is greater than the real volume. As a final possibility, Franklin[Citation32] suggests that changes in density are related to chemical composition, which in turn is affected by phase transitions during drying. Therefore, it is of interest to assess the effects of phase transitions on density in order to explore this phenomenon further.
Table 1 Particle density, apparent density, and glass transition temperature for garlic samples at 0.1 X/X 0
Figure 3 shows porosity (ϵ) as a function of X/X 0. The porosity of the raw garlic samples was found to be 0.14–0.16. Water loss caused a decrease in porosity when X/X 0 approached 0.1; this decrease was particularly drastic at drying temperatures of 50 and 60°C. Rahaman et al.[Citation30] explain porosity behavior through the analysis of tendencies found in various materials. According to these tendencies, porosity at 40°C is related to moisture content, whereas at higher temperatures, there is an increase in the formation of pores in the first stage of the drying process, until a critical value is reached, at which point a collapse occurs as a result of water loss. In addition, the effects of case hardening may affect the formation of pores,[Citation26] especially at high temperatures.[Citation30] Water elimination has been found to be accompanied by shrinkage; this results in an increase in apparent density and porosity during drying.[Citation10,Citation15,Citation19,Citation26,Citation31,Citation33–35 Citation Citation Citation35
Some authors[Citation10,Citation11] have proposed equations for the mathematical representation of changes in structural properties (ρ p , ρ b , ϵ); these equations are functions of moisture content. Nevertheless, at the end of the drying process, when the data has a non-linear tendency with respect to moisture content, these equations do not provide a suitable fit. An approach for fitting experimental data to an empirical model is presented in EquationEq. (13), composed of the sum of three exponential terms whose coefficients are shown in . A good fit for experimental data was found (R 2 > 0.85) for this equation:
Table 2 Coefficients corresponding to EquationEq. (13) for particle density, apparent density, and apparent porosity for garlic samples dehydrated at 40, 50, and 60°C
According to Aguilera and Stanley,[Citation12] cellular structure is affected when water in the protoplasm migrates through the cell membrane towards surrounding cell walls, and through the pores of the surface tissue, causing membrane rupture (cracking). The effects of drying on the cellular structure of garlic are shown in . Well-defined cellular structure was found in raw garlic (), whereas a large amount of cracking and loss of tridimensional cellular structure was found in samples dehydrated at high temperatures (). Similar results were reported by Bai et al.[Citation36] for apple rings dehydrated by convection. Sacilik and Unal[Citation37] found that the structural characteristics of Kastamonu garlic slices were affected by the drying air temperature and the thickness of the sample. Damage in the cellular structure of garlic (cellular cracking) may result in the loss of important nutraceutical properties.[3,4,6] The structural behavior shown in micrographs of dried samples is consistent as to porosity () and density () at the end of the drying process. A small amount of cracking in cellular structure with few pores indicates a denser product.[Citation12]
Figure 4 Microstructure of fresh garlic (a), garlic dried at 40°C (b), at 50°C (c), and at 60°C (d).
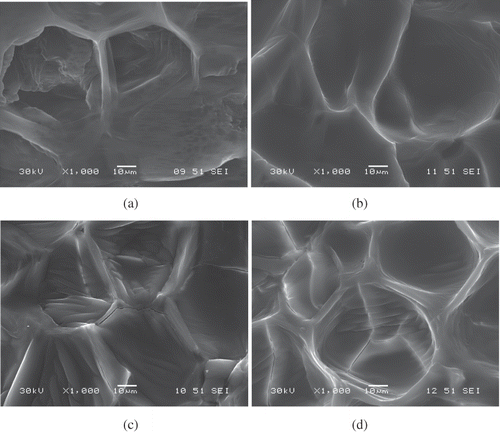
Micro-structural aspects are reflected in transference coefficients. Changes in cellular structure during the drying process affect moisture diffusion (D) in materials.[Citation12] In , a decrease in D can be observed as a function of a decrease in X/X 0 and drying air temperature. Dense products with lower porosity were found to display lower diffusivity (), which agrees with the results found by King.[Citation38] In dense products, moisture transfer from the interior of the material to the surface is lesser than in porous materials. Similar results were obtained by Monteau et al.[Citation39] and Marinos-Kouris and Maroulis.[Citation21]
According to the William-Landel-Ferry theory[Citation40] of glass transition, molecular mobility is limited by the phase which the material is in. Low moisture diffusivity is accompanied by a decrease in the mobility of polymer chains and an increase in Tg .[Citation26] At low moisture content levels, diffusivity is no longer a dominant mechanism, as at that point the material passes into an amorphous glassy state.[Citation20] This behavior was identified in X/X 0 values between 0.13 and 0.07 ()
Figure 6 shows the intersection of glass transition temperature (Tg ) with the inside (Ti ) and surface (Ts ) temperatures for garlic slices. A critical point was found when Ti ≅ Ts ≅ Tg , which indicates a second order phase transition. The identification of this critical point may aid in the determination of the appropriate time to stop the drying process in order to prevent undesirable changes in structural properties. The glassy state was identified at moisture contents of 0.0749, 0.0937, and 0.1172 kg/kg for garlic dehydrated at 60, 50, and 40°C, respectively, when (Tg − Ti ) > 0 and (Tg − Ts ) > 0.
After a phase transition, the molecular mobility of foodstuffs is slower, and the product is more brittle than it was prior to the transition. As a result of this effect, Tg can be used as a reference to evaluate quality degradation occurring at a given point in the drying process. Many studies have found that the drying temperature and the thermal history of the material affect the Tg of foodstuff samples.[Citation14–17 Citation Citation Citation17
Previous studies have suggested the importance of phase transitions that occur during the drying process, and their effects on structural properties.[Citation15–17 Citation Citation17,Citation30,Citation36] Conformational arrangements, molecular arrangements, and the formation of new compounds can be produced both by the thermal effects of drying[Citation3] and by second order phase transitions.[Citation18] In pure materials, such as carbon, Franklin[Citation32] demonstrated that density decreases as the hydrogen present in the carbon sample increases, indicating a change in the chemical characteristics of the material. Despite the lack of experimental evidence of the density-composition relationship in complex foods such as garlic, the presence of a significant number of chemical changes in superficial and internal structures as a result of the drying process has been demonstrated (enzymatic and non-enzymatic reactions, browning, color changes). Thus, as changes in chemical composition produce changes in density, and second order phase transitions affect chemical composition, a change in density can be expected.
A phase transition () related to slope changes in the tendencies of the particle density, apparent density, and porosity of garlic was observed during the drying process (Figs. 1–3). Additionally, high values of Tg were found in the more dense samples (). These results confirm that the chemical composition of the sample was modified by phase transitions occurring during the drying process, and that a new product was formed during drying, with different characteristics from those of the original material.
Figure 6 Glass transition temperature, surface temperature, and inside temperature for garlic slices during drying.
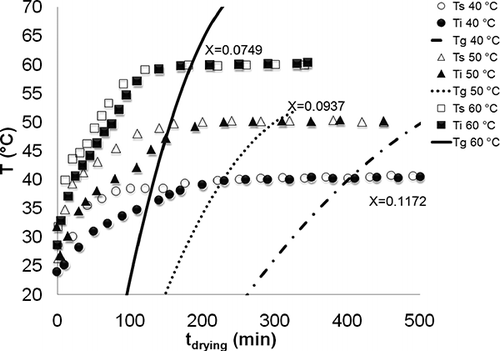
The results of this study also demonstrate a decrease in porosity as Tg increases. At the end of the drying process, (X/X 0 = 0.038), Tg was found to have values of 65.51, 62.00, and 59.76, and porosity was found to be 0.06, 0.09, and 0.14 for drying temperatures of 40, 50, and 60°C, respectively. These results confirm the explanation put forth by Kasapis et al.,[Citation17] and Sablani et al.,[Citation15] who indicated that pore formation irreversibly affects cellular structure during dehydration, and may be related to glass transition temperature.
CONCLUSIONS
ρ b , ρ p , ϵ, and D were found to be functions of drying air temperature, moisture content, and the phase that the material was in. A critical point in the intersection of Ti , Ts , and Tg was found; this point was identified as a second order phase transition. This second order phase transition was associated with a change in the slopes of structural properties (ρ b , ρ p , and ϵ). A drastic tendency change was found in porosity (ϵ) with respect to moisture content, particularly at high temperatures and low moisture contents. A nonlinear, three term exponential equation was proposed to represent changes in ρ b , ρ p , and ϵ as a function of moisture content, with an R 2 > 0.85. Micrograph analysis confirmed that porosity and density at the end of the drying process were related to cracking in cellular structure. Moisture diffusion (D), as calculated, was consistent with the density, porosity, and glass transition temperature observed throughout the drying process. These results indicate that the chemical composition, structure, and cellular arrangement of samples at the end of the drying process were different from those of the original, raw samples.
NOMENCLATURE
D | = |
Diffusivity over time (t) (m2/s) |
HR | = |
Relative humidity (%) |
kG-T | = |
Gordon-Taylor constant |
l | = |
Thickness (m) |
m | = |
Mass (kg) |
ms-a | = |
Mass of the solid in air (kg) |
ms-li | = |
Mass of the solid in an immersion liquid (kg) |
T | = |
Temperature (°C) |
Tdrying | = |
Drying air temperature (°C) |
Tg | = |
Glass transition temperature (°C) |
Ti | = |
Inside temperature of the garlic slice (°C) |
Ts | = |
Surface temperature of the garlic slice (°C) |
t | = |
Time (min) |
V | = |
Volume (m3) |
v | = |
Drying air velocity |
W | = |
Mass fraction (kg/kgtotal) |
X | = |
Moisture content (kg of water/kg dry solid) |
Greek Letters
ϵ | = |
Porosity |
ρ | = |
Density (kg/m3) |
Subscripts
a | = |
Air |
b | = |
Apparent |
dw | = |
Water displacement (solid in immersion liquid) |
e | = |
Equilibrium |
exp | = |
Experimental |
l | = |
Immersion liquid |
p | = |
Particle |
s | = |
Dry solid |
th | = |
Theoretical |
w | = |
Water |
0 | = |
Initial |
Dimensionless Groups of Physical Properties
Fo | = |
Fourier diffusion number |
W | = |
Non-dimensional moisture content |
ACKNOWLEDGMENTS
The authors would like to thank the SIP and the COFAA of the Instituto Politécnico Nacional for the facilities provided to carry out this investigation, and their generous financial support of the project SIP-20110358 and scholarship from CONACyT.
REFERENCES
- Chua , K.J. , Ho , J.C. , Chou , S.K. , Mujumdar , A.S. and Hawlader , M.N.A. 2002 . Analytical study of cyclic temperature drying: Effect on drying kinetics and product quality . Journal of Food Engineering , 51 : 65 – 75 .
- Aguilera , J.M. 2003 . Drying and dried products under the microscope . Food Science and Technology International , 9 ( 3 ) : 137 – 143 .
- Amagase , H. , Petesch , B.L. , Matsuura , H. , Kasuga , S. and Itakura , Y. 2001 . Intake of garlic and its bioactive components . The Journal of Nutrition , 131 : 955S – 962S .
- Lawson , L.D. 1993 . “ Bioactive organosulfur compounds of garlic and garlic products: Role in reducing blood lipids ” . In Human Medicinal Agents from Plants , Edited by: Kinghorn , A.D. and Balandrin , M.F. 306 – 330 . Washington , D.C : American Chemistry Society . InEds
- Lanzotti , V. 2006 . The analysis of onion and garlic . Journal of Chromatography A , 1112 : 3 – 22 .
- Jones , M.G. , Hughes , J. , Tregova , A. , Milne , J. , Tomsett , A.B. and Collin , H.A. 2004 . Biosynthesis of the flavor precursors of onion and garlic . Journal of Experimental Botany , 55 ( 404 ) : 1903 – 1918 .
- Amagase , H. 2006 . Clarifying the real bioactive constituents of garlic . The Journal of Nutrition , 136 : 716S – 725S .
- Pezzutti , A. and Crapiste , G.H. 1997 . Sorptional equilibrium and drying characteristics of garlic . Journal of Food Engineering , 31 : 113 – 123 .
- Sharma , G.P. and Prasad , S. 2001 . Drying of garlic (Allium sativum) cloves by microwave-hot air combination . Journal of Food Engineering , 50 : 99 – 105 .
- Lozano , J.E. , Rostein , E. and Urbicain , M.J. 1983 . Shrinkage, porosity and bulk density of foodstuffs at changing moisture contents . Journal of Food Science , 48 : 1497 – 1553 .
- Madamba , P.S. , Driscoll , R.H. and Buckle , K.A. 1994 . Shrinkage, density and porosity of garlic during drying . Journal of Food Engineering , 23 : 309 – 319 .
- Aguilera , J.M. and Stanley , D.W. 1992 . Microstructural Principles of Food Processing and Engineering , 343 London and New York : Elsevier Applied Science .
- Rahman , M.S. , Al-Amri , O.S. and Al-Bulushi , I.M. 2002 . Pores and physico-chemical characteristics of dried tuna produced by different methods of drying . Journal of Food Engineering , 53 : 301 – 313 .
- Roos , Y.H. 1987 . Effect of moisture on the thermal behavior of strawberries studied using DSC . Journal of Food Science , 52 : 146 – 149 .
- Sablani , S.S. , Rahman , M.S. , Al-Kuseibi , M.K. , Al-Habsi , N.A. , Al-Belushi , R.H. , Al-Marhubi , I. and Al-Amri , I.S. 2007 . Influence of shelf temperature on pore formation in garlic during freeze drying . Journal of Food Engineering , 80 : 68 – 79 .
- Sablani , S.S. , Kasapis , S. and Rahman , M.S. 2007 . Evaluating water activity and glass transition concepts for food stability . Journal of Food Engineering , 78 : 266 – 271 .
- Kasapis , S. , Rahman , M.S. , Al-Marhubi , I.M. and Al-Mahroqi , A. 2007 . Porosity and the effect of structural changes on the mechanical glass transition temperature . Journal Agricultural and Food Chemestry , 55 : 2459 – 2466 .
- Martínez-Navarrete , A. , Chiralt , A. and Fito , P. 1999 . Termodinámica y Cinética de Sistemas: Alimento-Entorno , Mexico : Instituto Politécnico Nacional .
- Attanasio , G. , Cinquanta , L. , Albanese , D. and Matteo , M. 2004 . Effects of drying temperatures on physic-chemical properties of dried and rehydrated chestnuts (Castanea sativa) . Food Chemistry , 88 : 583 – 590 .
- Levine , H. and Slade , L. 1989 . Interpreting the Behavior of Low-Moisture Foods, Water and Food Quality; Hardman, T.M , 71 – 134 . London : Elsevier Applied Science .
- Marinos-Kouris , D. and Maroulis , Z.B. 2006 . “ Transport properties in the drying of solids, Part I ” . In Hand book of Industrial Drying , 3rd , 1280 CRC Press: Atlanta, GA, USA . Edition
- Perry , R.H. and Green , D.W. 2001 . Manual del Ingeniero Químico , 6th , México : McGraw-Hill . Edition
- Kataranos , V.T. , Villalobos , G. and Saravacos , G.D. 1990 . Comparison of two methods of estimation of the effective moisture diffusivity from drying data . Journal of Food Science , 5 ( 1 ) : 219 – 233 .
- Rodríguez-Rámirez , J. , Méndez-Lagunas , L. , Martínez-Álvarez , C. and Diego-Nava , F. 2001 . “ A closed loop tunnel for drying kinetics research ” . In Inter-American Drying Conference , 499 – 504 . Veracruz , , Mexico, July 8–10 : Boca del Río .
- Mohsenin , N.N. 1980 . Physical Properties of Plant and Animal Materials , 742 New York : Gordon and Breach Science Publishers, Inc .
- Zogzas , N.P. , Maroulis , Z.B. and Marinos-Kouris , D. 1994 . Density, shrinkage and porosity of some vegetables during air drying . Drying Technology , 12 ( 7 ) : 1653 – 1666 .
- Crank , J. 1975 . The Mathematics of Diffusion , Second , New York : Oxford University Press . Edition
- Gordon , M. and Taylor , J.S. 1952 . Ideal copolymers and the second order transitions of synthetic rubbers. I. Noncrystalline copolymers . Journal of Applied Chemistry , 2 : 493 – 500 .
- Simha , R. and Boyer , R.F. 1962 . On a general relation involving the glass transition temperature and coefficients of expansion of polymer . Journal of Chemical Physics , 37 : 1003 – 1007 .
- Rahman , M.S. , Hussain , M.A. and Ng , C.W. 2002 . Prediction of pores formation (porosity) of food during drying: Generic models by the use of hybrid neural network . Journal of Food Engineering , 51 : 239 – 248 .
- Krokida , M.K. and Philippopoulos , C. 2005 . Rehydration of dehydrated foods . Drying Technology , 23 : 799 – 830 .
- Franklin , R.E. 1948 . A note on the true density, chemical composition, and structure of coals and carbonizes coals . Fuel , 27 : 46 – 49 .
- Wang , N. and Brennan , J.G. 1990 . Changes of structure, density and porosity of potato during dehydration . Journal of Food Engineering , 24 : 61 – 76 .
- Talla , A. , Puiggali , J.R. , Jomaa , W. and Janno , Y. 2004 . Shrinkage and density evolution during drying of tropical fruits: Application to banana . Journal of Food Engineering , 64 : 103 – 109 .
- Gabas , A.L. , Marra-Júnior , W.D. , Telis-Romero , J. and Telis , V.R.N. 2005 . Changes of density, thermal conductivity, thermal diffusivity, and specific heat of plums during drying . International Journal of Food Properties , 8 : 233 – 242 .
- Bai , Y. , Rahman , S. , Perera , C.O. , Smith , S. and Melton , L.D. 2002 . Structural changes in apple rings during convection air-drying with controlled temperature and humidity . Journal of Agricultural and Food Chemestry , 50 : 3179 – 3185 .
- Sacilik , K. and Unal , G. 2005 . Dehydration characteristics of kastamonu garlic slices . Biosystems Engineering , 92 ( 2 ) : 207 – 215 .
- King , J.C. 1980 . Separation Process , New York : McGraw-Hill .
- Monteau , J. , Hamdami , N. and Bail , A.L. 2004 . Transport properties of a high porosity model food at above and sub-freezing temperatures. Part 2: Evaluation of the effective moisture diffusivity from drying data . Journal of Food Engineering , 62 : 385 – 392 .
- Williams , M.L. , Landel , R.F. and Ferry , J.D. 1955 . The temperature dependence of relaxation mechanisms in amorphous polymers and other glass-forming liquids . Journal of American Chemical Society , 77 : 3701 – 3707 .