Abstract
The effect of addition of six fatty acids (stearic, palmitic, myristic, oleic, palmitoleic, and myristoleic acid) on the gelatinization, glass transition, and retrogradation properties of corn starch as well as their complexing abilities with amylose were determined. Differential scanning calorimeter studies reflected that addition of fatty acids caused a 73–89% decrease in the gelatinization enthalpy compared to that of the native starch. Besides amylose-lipid formation, exotherm was determined at the same temperature range with the gelatinization endotherm. As a result, it was suggested that fatty acids complexed with amylose during gelatinization. Fatty acid addition significantly increased the glass transition temperature of starch gel. This was attributed to two reasons: the first is due to the physical cross-linking action of amylose–lipid complexes in starch-water system; the second may be due to the effect of uncomplexed fatty acids on water distribution in the gel structure as a result of their amphiphilic character. Thermal properties of amylose-lipid complexes were compared in order to determine the effect of fatty acid properties. It was found that the shorter chain length and unsaturation favored the complex formation but the complexes formed by longer and saturated fatty acids were more heat stable. Addition of fatty acids resulted in 73–90% and 47–71% reduction in the retrogradation enthalpy compared to native starch gels at 5°C and 21°C, respectively. The reduction in the retrogradation enthalpy was inversely related to the amylose-lipid complexing abilities of the fatty acids and it might be explained by the hindrance effect of uncomplexed fatty acids to the water distribution in the starch gel matrix.
INTRODUCTION
Starch contains two main natural polymers that consist of 1,4-a-D-glucose units: amylose and amylopectin. Amylose is an essentially linear polymer where amylopectin is highly branched through a-D-1,6 glycosidic linkages. Amylose chains display a natural twist in a helical conformation with six glucose units per turn and have the unique feature to form helical inclusion complexes with several organic and inorganic complexing agents,[Citation1] and the internal cavity of the helix is considered to be a hydrophobic tube.[Citation2] The best-known inclusion complexes are amylose–lipid complexes, naturally present in starch and/or formed during gelatinization with endogenous or added lipids.[Citation3] Their importance is reflected in numerous food applications, because they can affect the functional,[Citation4] rheological,[Citation5] and nutritional[Citation6] properties of the foods. Besides, other fields of science, such as nanotechnology, helical wrapping of carbon nanotubes,[Citation7] and biotechnology,[Citation8] study the amylose-complexing abilities.
The chain length and degree of unsaturation of lipids have an impact on the thermal properties of amylose-lipid complex.[Citation9] Many authors have described dissociation temperatures of amylose-lipid complexes to increase with the length of the aliphatic chain of the lipid.[Citation10–18 Citation Citation Citation Citation Citation Citation Citation Citation−18 They attributed this to the lower hydrophilicity of longer lipid chains, and the resulting stronger hydrophobic interactions with the interior of the helix, requiring higher temperatures to break these bonds.[Citation19] The degree of unsaturation of the aliphatic lipid chain also has an important effect on the thermal properties of the amylose-lipid complexes. The higher the degree of unsaturation is, the lower the thermal stability of the resulting complex is.[Citation4, Citation10, Citation11, Citation19–23 Citation Citation Citation Citation−23 However, there is a disagreement on the effect of chain length of lipid on the complexing ability.[Citation9] Some researchers suggested that a lipid chain length with 14 carbon atoms is best for complex formation,[Citation20, Citation24, Citation25] while others have reported 16 or 18 carbon atoms to be the preferred lipid chain length.[Citation20,Citation21]
Retrogradation consists of two separable processes involving amylose and amylopectin. These are the fast gelation of amylose solubilized during gelatinization, and slow recrystallization of amylopectin within the gelatinized granules.[Citation26] Complex formation restricts the solubility and mobility of amylose. This prevents amylose double helix formation and crystallization.[Citation27–29 Citation Citation−29 Amylose double helices might function as nuclei for retrogradation by co-crystallizing with amylopectin.[Citation27] Therefore, lipid addition may have an impact this process in two ways. First, amylose-lipid complexation can prevent the co-crystallization with amylopectin. Second, lipids can also complex with the outer branches of amylopectin and, as such, inhibit retrogradation in a more direct way.[Citation27, Citation30, Citation31]
It has been well documented that lipids complex with starch.[Citation32–35 Citation Citation Citation−35 However, there are questions that remain unanswered concerning the effect of fatty acids on starch properties. For instance; the influence of fatty acid properties on the amylose complexing ability is still questioned in the literature. Moreover, saturated and unsaturated fatty acids may affect the retrogradation in different ways at different storage temperatures, which is the subject of another unanswered question in the literature. Besides, negligible research exists in the literature that questions the effects of fatty acids on the glass transition temperature of starch gel. The main object of this study was to shed a light on those indefinite points and provide a useful insight into the bonding between fatty acids and starch.
MATERIALS AND METHODS
Materials
Native corn starch and the fatty acids (stearic, C18:0; palmitic, C16:0; myristic, C14:0; oleic, C18:1; palmitoleic, C16:1; and myristoleic acid, C14:1) were purchased from Sigma Chemical Co. (St. Louis, MO, USA). Hexane was purchased from Merck Chemicals (Darmstadt, Germany). Moisture content of native corn starch was determined using a standard method[Citation36] and was found to be 11.2 ± 0.1 g water per 100 g starch.
Preparation of Starch-Fatty Acid Mixtures
In order to obtain a homogenous starch-fatty acid mixture, fatty acid was first dissolved in hexane and then this mixture was added to the starch. This triple mixture was stirred at 100 rpm overnight at room temperature and most of the hexane was removed naturally. Then, the remaining hexane was removed using an inert (nitrogen) gas stream. After that, the sample was milled mildly and stored in glass containers until taken to further analysis. The sample contained 3 g fatty acid/100 g dry starch.
Gelatinization and Glass Transition Experiments
A differential scanning calorimetry (DSC) technique was used to follow gelatinization and glass transition of starch-fatty acid mixtures. Experiments were carried out using Q20 model DSC equipment (TA Instruments, New Castle, DE, USA). The calorimeter was calibrated with indium (melting point = 156.6°C, ΔH = 28.5 J/g). The DSC runs were operated under nitrogen gas atmosphere (30 mL/min) and an empty pan was used as the reference. The scanning rate was 10°C/min for all experiments.
The starch-fatty acid mixture (3–4 mg) was weighed into an aluminum pan and distilled water was subsequently added to make the ratio of water to dry starch of 3:1 (w/w). The aluminum hermetic pan and pan lids were used in the DSC experiments, which had a capacity of 10 mg (Part No: 900793.901, 900794.901, TA Instruments). Afterwards, the pan was hermetically sealed and allowed to stabilize at room temperature overnight. The pan was heated (1st scan) from room temperature (21 ± 3°C) to 120°C, then cooled to −30°C (2nd scan) and immediately reheated to 120°C (3rd scan) under the nitrogen gas stream (30 mL/min). The DSC thermograms were evaluated to define the onset, peak, and completion temperatures and the enthalpy values associated with the endothermic and exothermic transitions. All transition enthalpies were expressed in joules per gram of dry starch (J/g ds). The second order transition, preceding the initial point of ice melting, corresponds to the apparent glass transition of solid–unfreezable water mixture as suggested by Rahman[Citation37] and Rahman et al.[Citation38] In order to determine the apparent glass transition temperatures, the first derivatives of the … 3rd scans were used. Glass transition was analyzed for the onset, mid, and end points, and the midpoint temperatures were reported as apparent glass transition temperatures (Tg″′) of the starch gel samples.
Retrogradation Experiments
Each sample pan to be used in the retrogradation experiment was prepared according to the procedure mentioned in the previous section. After the heating (1st scan) from room temperature (21 ± 3°C) to 120°C and the cooling (2nd scan) procedure, sample pans were stored at room temperature (21 ± 3°C) and in a refrigerator (5 ± 2°C) for 30 days. At certain time intervals (5, 10, 15, 20, 25, and 30 days of storage), one sample pan was removed from the batch and reheated for retrogradation analysis (3rd scan). For each storage time and temperature, three independent replicates were used. The endothermic peak obtained in the 3rd scan was analyzed quantitatively. The enthalpy associated with retrogradation (ΔHr ) was measured and expressed in J/g ds.
Statistical Analysis
Experimental data were subjected to one-way analysis of variance (ANOVA), using SPSS version 11.5 (SPSS Inc., Armonk, NY, USA). Treatment means were tested separately for least significant difference (LSD) test.
RESULTS AND DISCUSSION
General Evaluation of DSC Thermograms
DSC is a valuable tool for obtaining information about amylose-lipid complex formation and the influence of fatty acids on starch properties. When starch-water mixture is scanned in the presence of fatty acids with heating/cooling/reheating cycles, several peaks are observed due to the lipid transitions, amylose-lipid transitions, starch gelatinization, and phase transitions of water. The transitions observed between 40–110°C were presented for myristoleic acid-corn starch mixture in . During the 1st scan, the sample was heated up to 120°C and starch was gelatinized as shown by an endothermic peak (). It is generally agreed by several authors that the amylose-lipid complex is formed during gelatinization with the endogenous and/or added lipids. In these studies, this is evidenced by the decrease in the gelatinization enthalpy.[Citation32–34 Citation Citation−34 In our study, the gelatinization enthalpies (H), for starch-fatty acid mixtures were determined to be 73–89% smaller than that of the control sample (). Similar values have been reported in the literature.[Citation39] Siswoyo and Morita[Citation39] reported that the gelatinization enthalpies of starch-glycerophosphatidyl-coline mixtures were 32–85% smaller than that of the defatted starch sample. The observed decrease in the gelatinization enthalpy values by the fatty acid addition can be explained as the result of the endothermic gelatinization and exothermic complex formation reactions taking place at the same time.[Citation34, Citation39, Citation40] After gelatinization as the temperature was raised, the complex formed during gelatinization would be dissociated consequently. This dissociation transition was presented as a very small endotherm above 100°C (), and it was not possible to detect its area or temperature properly because of its small size.
Figure 1 DSC thermograms of corn starch scanned with heating/cooling/reheating cycles in the presence of fatty acid (sample; myristoleic acid:corn starch:water = 0.03:1:3). (Color figure available online.)
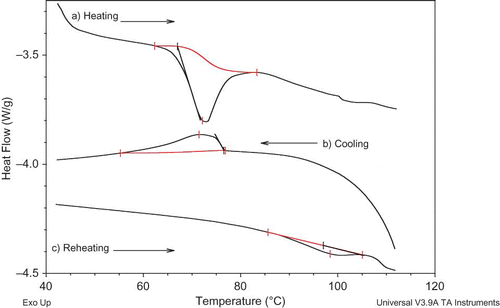
Table 1 Effect of fatty acids on glass transition temperature and gelatinization endotherm parameters of corn starch
During cooling (2nd scan), the amylose-lipid complex, dissociated during the 1st scan, was reformed as shown by an exothermic peak (). It is generally accepted by several researchers that the amylose-lipid complex exhibited a reversible thermal transition on DSC thermograms.[Citation17, Citation32, Citation34] Finally, the 3rd scan (immediate reheating) showed the dissociation endotherm of amylose-lipid complex that was formed during cooling scan (). Here it should be noted that the exothermic peak corresponding to the complex formation was at a lower temperature range than the dissociation endotherm and almost at the same temperature range with the gelatinization endotherm. The coincidence of the temperature ranges of gelatinization endotherm () and amylose-lipid formation exotherm (), indicated that the amylose-lipid complexes could be formed during gelatinization. Similar results were reported with the amylose-monoglyceride complexes.[Citation11] Moreover, the amylose-lipid complex dissociation endotherm area obtained in the reheating cycle () was detected to be considerably larger, compared to the one present in the first heating scan (). This observation is in well accordance with the Tufvesson et al.[Citation17] study in which they mentioned that heating/cooling/reheating cycles could favor the complex formation.
Effect of Fatty Acid Properties on Gelatinization
The effect of addition of various fatty acids on the gelatinization of native corn starch is presented in . Gelatinization enthalpy and temperature values for the samples (C16:0+starch and C18:0+starch) are not detected and presented in , due to the overlapping of gelatinization endotherm of starch and chain melting endotherm of the fatty acids. indicated the effects of fatty acid properties on the apparent gelatinization enthalpy. The apparent enthalpy values decreased significantly as the fatty acids chain length decreased. Moreover, when C14:1+starch sample was compared to its saturated counterpart (C14:0+starch), the enthalpy value decreased to a greater extent (). If the decrease in the gelatinization enthalpy was considered to correspond to the relative amount of complex formation in starch, it could be concluded that the amylose-fatty acid complex formation increases as the chain length of fatty acids decreases and also by introducing unsaturation into the lipid.
With the exception of a small shift in peak temperature to higher values, the onset and completion temperatures of gelatinization endotherm curve were not significantly changed by fatty acid addition (). Different results have been reported in the literature.[Citation34] The emulsifiers, such as monoglycerides, were found to create an increase of 1–3°C in the peak temperature, which is in accordance with our results.[Citation34] On the contrary, Zhou et al.[Citation29] found that the addition of lipids slightly decreased the peak temperature of gelatinization endotherm. But they also reported that the onset and completion temperatures of gelatinization endotherm curve were not significantly changed, which is in accordance with our data.[Citation29] It is generally agreed that the influence of added lipid on the gelatinization temperature range could be changed according to the type of the lipid.[Citation34]
Glass Transition Temperatures
In order to further analyze the effect of fatty acid addition on the gel structure, we measured the apparent glass transition temperatures (Tg′′′) of the starch gels with and without fatty acids (, ). When the sample is cooled, ice formation often causes the solid concentration to increase in unfrozen water. Therefore, the transition preceding apparent (Tm′) (initial point of ice melting) corresponds to the glass transition of solid matrix (mixture of solid and unfreezable water) in the frozen sample. This temperature is named as apparent glass transition temperature (Tg″′).[Citation37,Citation38] The Tg″′ of starch gel was determined as −5.4°C, and between −4.0 and −3.4°C for fatty acid added counterparts (). Fatty acid addition significantly increased the Tg″′ of starch gel. This might be attributed to two reasons: the first one is due to the amylose complexation with the fatty acids and moreover amylose–lipid complexes might act as physical cross-linking agents in a starch-water system.[Citation41] The second one may be due to the effect of free fatty acids on water distribution in the gel structure as a result of their amphiphilic character. It is well known that water has a very good plasticizing effect on completely amorphous and partially crystalline polymers.[Citation42] The starch gel holds moisture as an integral part of solid structure via hydrogen bonding and moreover a greater proportion of amorphous chains in the gelatinized starch interact with water. Besides, additional lipids can form an insoluble layer around the granules, preventing the entry of water[Citation43] and complexation between amylose and these lipids, either at the surface or inside the granule, and also contributes to these phenomena, mostly by preventing leaching of soluble carbohydrates.[Citation25,Citation44] Therefore, the structural differences outcoming by fatty acid-water and/or amylose-lipid complex-water interactions could result in a decrease in available water content of gelatinized starch; consequently, its plasticizing effect was decreased and higher temperatures were required for the glass transition.
Effect of Fatty Acid Properties on Amylose-Lipid Complex
The effect of fatty acid properties on the amylose-lipid complex were evaluated by investigating the cooling and reheating thermograms of starch gel-fatty acid mixtures. The thermograms were given in and the transition temperatures together with enthalpies were presented in . During the cooling cycle, two exotherms were observed with the exception of C14:1+starch mixture (). The higher temperature exotherm (71–83°C) reflected the amylose-lipid complex formation as mentioned before and the lower one (30–65°C) corresponded to the recrystallization of uncomplexed fatty acid (). For the C14:1+starch sample, the recrystallization temperature of myristoleic acid is at a lower temperature than the thermogram's temperature range and was not observed (). In the immediate reheating thermograms (), the dissociation endotherm of amylose-lipid complex was observed at around 100°C. For the saturated fatty acid + starch samples (C14:0+starch, C16:0+starch, and C18:0+starch), the chain melting endotherm of uncomplexed fatty acids were also observed between 55–75°C ().
Figure 3 DSC thermograms of starch gel during (a) cooling and (b) reheating scans in the presence of fatty acids (fatty acid:corn starch:water = 0.03:1:3). (Color figure available online.)
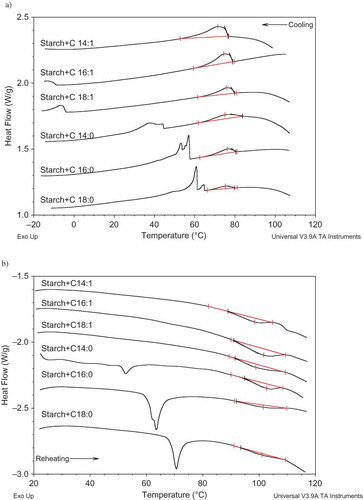
In order to compare the complexing ability of fatty acids with starch, three parameters, namely, transition enthalpies (ΔHc ), transition temperatures, and the width of the complex transition peaks, (To –Tc ), were taken into consideration (). Among these parameters, transition enthalpy can be taken as the measure of the relative amount of complexes in the sample. Transition temperatures are mainly considered as a measure of heat stability. The higher the temperatures are, the more stable the complexes are. Finally the width of the complex transition peaks can be inversely related to the cooperativity and perfection of crystallites.[Citation17]
As it is seen in , the ΔHc was significantly affected by the fatty acid properties. For example, ΔHc value increased by the decrease in the chain length both during cooling and reheating scans. For unsaturated fatty acids, the enthalpy values were higher than their saturated counterparts (). Therefore, it could be concluded that the shorter chain length and unsaturation favored the complex formation. Similar results were found by Tufvesson et al.[Citation17] where they explained this tendency by the solubility of the fatty acids. In fact, two factors, the chain length and saturation, influence the solubility of fatty acids in such a way that the solubility increases as the chain length and saturation decrease. The higher solubility means that more fatty acids are available for complex formation, which in turn results in more amylose-lipid complex formation, as it is also evident from our experimental data.
Table 2 Transition temperatures and enthalpies of amylose-lipid complexes
It should also be noted that lower ΔHc values were observed during reheating than the cooling scan. This could be the result of the formation of complexes with different heat stabilities during the cooling scan. Karkalas et al.[Citation4] determined the thermal stability of amylose-stearic acid complexes and found out that there could be three different types of complexes with the melting temperature ranges of 96–104, 114–121, and 121–125°C. Temperature ranges higher than 115°C were out of the scope of our experimental technique due to the use of standard hermetic pans. As a result, we were not able to observe the dissociation endotherms at higher temperatures. If more than one type of heat stable complexes were formed during cooling, they would dissociate at different temperature ranges higher than 115°C. This could be the reason for lower ΔHc values obtained in reheating, compared to the cooling in our study.
The transition temperatures and the width of the transition peaks were also significantly affected by fatty acid properties (). During the cooling scan, the transition temperatures were increased with the increase in the chain length and saturation. Besides, the formation cooperativity of the complexes seemed to be higher for longer and saturated fatty acids, which was concluded from the width of the complex transition peaks, (To –Tc ). The width of the peaks decreased with the increase in chain length and saturation (). Therefore, it could be concluded that the complexes formed by longer and saturated fatty acids are more heat stable (, ). Similar results were reported in the literature.[Citation17,Citation45] In contrast to the cooling scan, no general trend could be detected for the dissociation transition temperatures and peak width during the reheating scan (, ).
Effect of Fatty Acids on Retrogradation
In order to follow the retrogradation in the presence of fatty acids, the retrogradation of starch gels in the presence of fatty acids at 5 and 21°C were observed for 30 days. Also, starch gel without fatty acids was taken into consideration as the control sample. The retrogradation enthalpy values for the control sample ranged from 0–9.21 J/g dry starch and from 0–5.42 J/g dry starch for storage at 5 and 21°C, respectively. Then a factor indicating the extent of retrogradation in fatty acid containing samples were defined as follows:
Figure 4 DSC thermograms of retrograded starch gels in the presence of fatty acids stored for 30 days at (a) 5°C and (b) 21°C (fatty acid:corn starch:water = 0.03:1:3). (Color figure available online.)
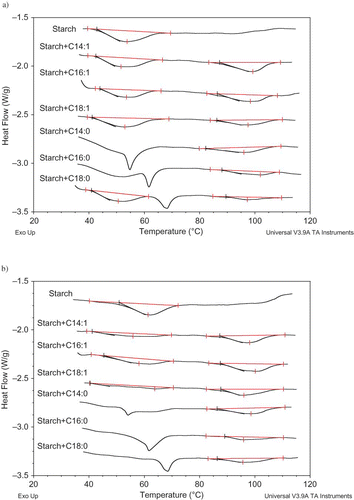
The addition of fatty acids resulted in a significant reduction in the retrogradation enthalpy relative to the value of the control sample. When the comparison was based on the retrogradation enthalpy values at the 30th day represented in , the retrogradation enthalpies were 90% (ΔHC14:1/ΔHcontol), 81% (ΔHC16:1/ΔHcontol), 73% (ΔHC18:1/ΔHcontol), 89% (ΔHC18:0/ΔHcontol) and 56% (ΔHC14:1/ΔHcontol), 71% (ΔHC16:1/ΔHcontol), 47% (ΔHC18:1/ΔHcontol) less than the control sample at 5 and 21°C, respectively. Studies[Citation48–50 Citation Citation−50 indicated that the complexation between amylose and fatty acids would hinder the double helical associations and the formation of a crystalline region during storage. Since the retarding mechanism of lipids on retrogradation is generally related to their ability to form complexes with the amylose fraction,[Citation29,Citation37] it would be expected that the fatty acids with the higher complexing ability should be more effective on retarding the retrogradation. But, in our study, the reduction in the retrogradation enthalpy was inversely related to the amylose-lipid complexing abilities of the fatty acids (). This result raised the questions concerning that formation of amylose-lipid complex was not sufficient to explain the retarding effect of fatty acids. Since lipids retard or delay gelatinization probably by complexing with leached amylose on the surface of the starch granule,[Citation51] it is possible that lipids also retard the retrogradation by a similar mechanism, i.e., by acting as a barrier against water distribution.[Citation50] Hence, it is possible to assume that the presence of uncomplexed fatty acids could contribute this barrier mechanism against water distribution. It was also evidenced by the increase in the glass transition temperature of starch gel in the presence of fatty acids (). Due to the strong dependency of rate of retrogradation on water content,[Citation52] it is not surprising to expect that any blocking effect on water distribution could cause a delay on retrogradation. It should also be taken into account that storage temperature influenced the retarding effect of fatty acids (). At 21°C, fatty acids delayed the retrogradation to a greater extent (). This could be explained by higher molecular mobility at higher temperatures might contribute the retarding effect of fatty acids on retrogradation.
CONCLUSIONS
The following conclusions were derived from this study. Exogenous fatty acids complex with amylose during gelatinization and the shorter chain length and unsaturation favor the complex formation. This tendency was explained by the solubility of the fatty acids. The solubility increases as the chain length and saturation decrease. The higher solubility means more fatty acids are available for complex formation. Fatty acid addition significantly increased the glass transition temperature of starch gel. One possible explanation is the physical cross-linking action of amylose–lipid complexes in a starch-water system. The other one is the barrier effect of uncomplexed fatty acids on available water in the starch gel matrix as a result of their amphiphilic character. Fatty acid addition significantly reduced the retrogradation enthalpy at ambient temperatures. Unexpectedly, the extent of reduction in the retrogradation enthalpy was inversely related to the amylose-lipid complexing abilities of the fatty acids. This result indicated that formation of amylose-lipid complex was not sufficient to explain the retarding effect of fatty acids. This tendency was attributed to the uncomplexed fatty acids present in the starch gel.
ACKNOWLEDGMENTS
The authors wish to thank the Hacettepe University Research Centre Office (Project number: 05 D06 602 001) and TÜBİTAK (Project number: TOGTAG-3181) for providing funds in the form of a research project.
REFERENCES
- Zobel , H.F. 1988 . Molecules to granules—A comprehensive starch review . Starch-Starke , 40 ( 2 ) : 44 – 50 .
- Godet , M.C. 1993 . Molecular modeling of the specific interactions involved in the amylose complexation by fatty-acids . International Journal of Biological Macromolecules , 15 ( 1 ) : 11 – 16 . Train, V.; Delage, M.M.; Buleon, A
- Morrison , W.R. 1993 . Swelling and gelatinization of cereal starches. 4. Some effects of lipid-complexed amylose and free amylose in waxy and normal barley starches . Cereal Chemistry , 70 ( 4 ) : 385 – 391 . Tester, R.F.; Snape, C.E.; Law, R.; Gidley, M.J
- Karkalas , J. 1995 . Some factors determining the thermal-properties of amylose inclusion complexes with fatty-acids . Carbohydrate Research , 268 ( 2 ) : 233 – 247 . Ma, S.; Morrison, W.R.; Pethrick, R.A
- Tang , M.C. and Copeland , L. 2007 . Analysis of complexes between lipids and wheat starch . Carbohydrate Polymers , 67 ( 1 ) : 80 – 85 .
- Kitahara , K. 1997 . Release of bound lipids in cereal starches upon hydrolysis by glucoamylase . Cereal Chemistry , 74 ( 1 ) : 1 – 6 . Tanaka, T.; Suganuma, T.; Nagahama, T
- Lii , C.Y. 2003 . Single-walled carbon nanotube–potato amylose complex . Carbohydrate Polymers , 51 ( 1 ) : 93 – 98 . Stobinski, L.; Tomasik, P.; Liao, C. D
- Sundari , C.S. , Raman , B. and Balasubramanian , D. 1999 . Artificial chaperoning of insulin, human carbonic anhydrase and hen egg lysozyme using linear dextrin chains—A sweet route to the native state of globular proteins . FEBS Letters , 443 ( 2 ) : 215 – 219 .
- Putseys , J.A.L. and Delcour , J.A. 2010 . Amylose-inclusion complexes: Formation, identity and physico-chemical properties . Journal of Cereal Science , 51 : 238 – 247 .
- Raphaelides , S.N. and Papavergou , E.J. 1991 . An investigation of the quantitative relationship of amylose monoglyceride interactions . Food Science and Technology , 24 : 391 – 396 .
- Eliasson , A.C. and Krog , N. 1985 . Physical-properties of amylose monoglyceride complexes . Journal of Cereal Science , 3 ( 3 ) : 239 – 248 .
- Gelders , G.G. 2004 . Amylose-lipid complexation: A new fractionation method . Carbohydrate Polymers , 56 ( 4 ) : 447 – 458 . Vanderstukken, T.C.; Goesaert, H.; Delcour, J.A
- Gelders , G.G. , Goesaert , H. and Delcour , J.A. 2006 . Amylose-lipid complexes as controlled lipid release agents during starch gelatinization and pasting . Journal of Agricultural and Food Chemistry , 54 ( 4 ) : 1493 – 1499 .
- Godet , M.C. , Bizot , H. and Buleon , A. 1995 . Crystallization of amylose-fatty acid complexes prepared with different amylose chain lengths . Carbohydrate Polymers , 27 ( 1 ) : 47 – 52 .
- Godet , M.C. , Bouchet , B. , Colonna , P. , Gallant , D.J. and Buléon , A. 1996 . Crystalline amylose fatty acid complexes: Morphology and crystal thickness . Journal of Food Science , 61 : 1196 – 1201 .
- Stute , R. and Koniecznyjanda , G. 1983 . DSC investigations of starches .2. Investigations on starch-lipid-complexes . Starke , 35 ( 10 ) : 340 – 347 .
- Tufvesson , F. , Wahlgren , M. and Eliasson , A.C. 2003 . Formation of amylose-lipid complexes and effects of temperature treatment. Part 2 . Fatty acids. Starch-Starke , 55 ( 3–4 ) : 138 – 149 .
- Tufvesson , F. , Wahlgren , M. and Eliasson , A.C. 2003 . Formation of amylose-lipid complexes and effects of temperature treatment. Part 1 . Monoglycerides. Starch-Starke , 55 ( 2 ) : 61 – 71 .
- Raphaelides , S. and Karkalas , J. 1988 . Thermal-dissociation of amylose fatty-acid complexes . Carbohydrate Research , 172 : 65 – 82 .
- Krog , N. 1971 . Amylose complexing effect of food grade emulsifiers . Starch-Stärke , 23 : 206 – 209 .
- Lagendijk , J. and Pennings , H.J. 1970 . Relation between complex formation of starch with monoglycerides and firmness of bread . Cereal Science Today , 15 ( 10 ) : 354
- Zabar , S. , Lesmes , U. , Katz , I. , Shimoni , E. and Bianco-Peled , H. 2009 . Studying different dimensions of amylose-long chain fatty acid complexes: Molecular, nano and micro level characteristics . Food Hydrocolloids , 23 : 1918 – 1925 .
- Yamada , T. , Kato , T. , Tamaki , S. , Teranishi , K. and Hisamatsu , M. 1998 . Introduction of fatty acids to starch granules by ultra-high-pressure treatment . Starch-Stärke , 50 : 484 – 486 .
- Bhatnagar , S. and Hanna , M.A. 1994 . Amylose lipid complex-formation during single-screw extrusion of various corn starches . Cereal Chemistry , 71 ( 6 ) : 582 – 587 .
- Hoover , R. and Hadziyev , D. 1981 . Characterization of potato starch and its monoglyceride complexes . Starch-Stärke , 33 : 290 – 300 .
- Srichuwong , S. and Jane , J. 2007 . Physicochemical properties of starch affected by molecular composition and structures: A review . Food Science and Biotechnology , 16 : 663 – 674 .
- Gudmundsson , M. and Eliasson , A.C. 1990 . Retrogradation of amylopectin and the effects of amylose and added surfactants emulsifiers . Carbohydrate Polymers , 13 : 295 – 315 .
- Lebail , P. 2000 . Mobility of lipid in complexes of amylose-fatty acids by deuterium and C-13 solid state NMR . Carbohydrate Polymers , 43 ( 4 ) : 317 – 326 . Buleon, A.; Shiftan, D.; Marchessault, R.H
- Zhou , Z.K. 2007 . Effect of the addition of fatty acids on rice starch properties . Food Research International , 40 ( 2 ) : 209 – 214 . Robards, K.; Helliwell, S.; Blanchard, C
- Huang , J.J. and White , P.J. 1993 . Waxy corn starch: Monoglyceride interaction in a model system . Cereal Chemistry , 70 : 42 – 47 .
- Eliasson , A.C. and Ljunger , G. 1988 . Interactions between amylopectin and lipid additives during retrogradation in a model system . Journal of the Science of Food and Agriculture , 44 : 353 – 361 .
- Eliasson , A.C. 1985 . Starch-lipid interactions studied by differential scanning calorimetry . Thermochimica Acta , 95 ( 2 ) : 369 – 374 .
- Eliasson , A.C. , Finstad , H. and Ljunger , G. 1988 . A study of starch-lipid interactions for some native and modified maize starches . Starch-Starke , 40 ( 3 ) : 95 – 100 .
- Eliasson , A.C. 1994 . Interactions between starch and lipids studied by DSC . Thermochimica Acta , 246 ( 2 ) : 343 – 356 .
- Biliaderis , C.G. 1985 . Thermal-behavior of amylose-lipid complexes . Carbohydrate Polymers , 5 ( 5 ) : 367 – 389 . Page, C.M.; Slade, L.; Sirett, R.R
- AOAC . 1990 . Official Methods of Analysis of the Association of Official Analytical Chemists , Arlington, VA, USA : AOAC .
- Rahman , M.S. 2009 . Food stability beyond water activity and glass transtion: Macro-micro region concept in the state diagram . International Journal of Food Properties , 12 ( 4 ) : 726 – 740 .
- Rahman , M.S. 2010 . Development of state diagram of bovine gelatin by measuring thermal characteristics using differential scanning calorimetry (DSC) and cooling curve method . Thermochimica Acta , 509 ( 1–2 ) : 111 – 119 . Al-Saidi, G.; Guizani, N.; Abdullah, A
- Siswoyo , T.A. and Morita , N. 2003 . Physicochemical studies of defatted wheat starch complexes with mono and diacyl-sn-glycerophosphatidylcholine of varying fatty acid chain lengths . Food Research International , 36 ( 7 ) : 729 – 737 .
- Morrison , W.R. , Tester , R.F. and Gidley , M.J. 1994 . Properties of damaged starch granules. 2. Crystallinity, molecular order and gelatinization of ball-milled starches . Journal of Cereal Science , 19 ( 3 ) : 209 – 217 .
- Zhong , Z.K. and Sun , X.S. 2005 . Thermal characterization and phase behavior of cornstarch studied by differential scanning calorimetry . Journal of Food Engineering , 69 ( 4 ) : 453 – 459 .
- Maurice , T.J. 1985 . “ Polysaccharide–water interaction—Thermal behavior of rice starch ” . In Influence of Water on Food Quality and Stability , Edited by: Simatos , D. and Multon , J.L. 211 Netherlands , Dordrecht : Nijhoff . Slade, L.; Sirret, R.R.; Page, C.M
- van Lonkhuysen , H. and Blankestijn , J. 1976 . Influence of monoglycerides on gelatinization and enzymatic breakdown of wheat and cassava starch . Starch-Stärke , 28 : 227 – 233 .
- Lauro , M. , Poutanen , K. and Forssell , P. 2000 . Effect of partial gelatinization and lipid addition on alpha-amylolysis of barley starch granules . Cereal Chemistry , 77 ( 5 ) : 595 – 601 .
- Putseys , J.A. , Derde , L.J. , Lamberts , L. , Goesaert , H. and Delcour , J.A. 2009 . Production of tailor made short chain amylose-lipid complexes using varying reaction conditions . Carbohydrate Polymers , 78 : 854 – 861 .
- Miles , M.J. 1985 . The roles of amylose and amylopectin in the gelation and retrogradation of starch . Carbohydrate Research , 135 ( 2 ) : 271 – 281 . Morris, V.J.; Orford, P.D.; Ring, S.G
- Tian , Y.Q. 2009 . Effect of beta-cyclodextrin on the long-term retrogradation of rice starch . European Food Research and Technology , 228 ( 5 ) : 743 – 748 . Xu, X.M.; Li, Y.; Jin, Z.Y.; Chen, H.Q.; Wang, H
- Liu , H. 1997 . Amylose-lipid complex formation in acetylated pea starch-lipid systems . Cereal Chemistry , 74 ( 2 ) : 159 – 162 . Arntfield, S.D.; Holley, R.A.; Aime, D.B
- Leloup , V.M. 1992 . Microstructure of amylose gels . Carbohydrate Polymers , 18 ( 3 ) : 189 – 197 . Colonna, P.; Ring, S.G.; Roberts, K.; Wells, B
- Gudmundsson , M. 1994 . Retrogradation of starch and the role of its components . Thermochimica Acta , 246 ( 2 ) : 329 – 341 .
- Dappolonia , B.L. and Morad , M.M. 1981 . Bread staling . Cereal Chemistry , 58 ( 3 ) : 186 – 190 .
- Farhat , I.A. , Blanshard , J.M.V. and Mitchell , J.R. 2000 . The retrogradation of waxy maize starch extrudates: Effects of storage temperature and water content . Biopolymers , 53 ( 5 ) : 411 – 422 .