Abstract
Ovalbumin is the main constituent of egg white protein. Improvements in the functional properties of ovalbumin are of great importance in the food industry. Therefore, in this article, the structural and functional properties of ovalbumin, which were glycated by dry-heating, were investigated. The micrographs showed that glycated ovalbumin was smaller and more homogeneous than native and dry-heated ovalbumin. Although changes in the random coil structure of ovalbumin measured by circular dichroism were mild, increased surface hydrophobicity and decreased tryptophan fluorescence intensity were observed following glycation. Glycation improved the stability against heat induced insolubility, emulsifying properties, water and oil binding capacity of ovalbumin. These results suggested that dry-heating glycation is a useful method for improving the functional properties of ovalbumin. The attention on the structural and functional changes in this work will be contributed to the understanding of the structure-function relationship. These insights would have important implications for the creation of desirable structure and functional properties of food products.
INTRODUCTION
The extent of protein utilized in a food processing system is dependent on improving and tailoring the protein’s functional characteristics to meet the complex needs of manufactured food products.[Citation1,Citation2] Improvements in the functional properties of food proteins could lead to the maximization of their effective use in the food industry.[Citation3] Glycation of protein, by using nontoxic chemical modification through the Maillard reaction with different sugars, has been proved to be a useful method for improving the functional properties of food proteins, such as thermal stability, emulsifying ability, foaming properties, and gelling properties.[Citation4,Citation5]
Ovalbumin (OVA) is the main constituent of egg white protein, and its behavior predominantly affects the functional properties of egg white protein.[Citation6,Citation7] OVA is a globular, acidic protein that comprises a single polypeptide chain of 385 amino acid residues with a molecular weight of 45,000 D, and includes one disulphide bond, four free sulphydryl groups, 0–2 phosphoryl groups, and a carbohydrate chain, which is widely used in the food industry for its ability to foam and to form gels.[Citation8–12]
The increased demand for egg white protein products makes the research on OVA more attractive than ever. This suggests that some incentives exist in improving the functional properties of OVA and, consequently, allowing their efficient utilization in the food industry.[Citation13,Citation14] To contribute to the understanding of the structure-function relationship, we focused our attention on the structural and functional changes. At the same time, the structural changes and the functional properties of glycated OVAs were also characterized.
MATERIALS AND METHODS
The ovalbumin (Grade V minimum 98%, crystallized and lyophilized) was purchased from Sigma (St. Louis, MO, USA). Maltodextrin was purchased from Zhengzhou Natural Chemical Co. Ltd. (Zhengzhou, China). 1-Anilino-8-naphthalene sulfonate (ANS) was purchased from Nacalai Tesque Co., Ltd. (Kyoto, Japan). Solutions were prepared with deionized (DI) water processed with a Milli-Q ultra-high purity water system (Millipore, Bedford, MA, USA). All other chemicals used in this study were of analytical grade.
Preparation of Glycated OVA
Glycation was performed with maltodextrin (DE value 15–20). Ovalbumin was dissolved in DI water with maltodextrin at a ratio 100:9 and the concentration of protein is 50 g/l; the solution pH was adjusted to 8.0 with 1 N NaOH, lyophilized, and incubated at 55°C, RH (relative humidity) 75% for 3 days in an incubator. In comparison with G-OVA, dry-heated N-OVA (DH-OVA) was prepared. The N-OVA were dissolved in deionized water at a concentration of 50 g/l, and the solution pH was adjusted to 8.0 with 1 N NaOH, followed by lyophilization. The lyophilized sample was incubated at 55°C, RH 75% for 3 days in an incubator.
Observation of Microstructure of Protein Samples by Scanning Electron Microscope (SEM)
The microstructure of N-OVA, G-OVA, DH-OVA were observed by an ultra-high resolution SEM (S-4800, Hitachi, Japan) at an accelerating voltage of 5 kV, according to Oliveira et al.[Citation15]
Circular Dichroism (CD) Spectra
The CD spectra of samples were recorded using a JASCO J-810 spectropolarimeter (Tokyo, Japan) according to Li et al.[Citation16] Protein samples were dissolved in 20 mM phosphate buffer (pH 7.4) at a protein concentration of 0.01 g/l and were filtered using 0.02 μm membrane prior to CD measurements. Each CD spectrum was the accumulation of three scans at 200 nm/min with a 1 nm bandwidth and a time constant of 1 s. The cylindrical cell used had a path length of 10 mm and the data was collected from 200 to 260 nm. Estimation of secondary structure composition was performed using the JASCO SSE-338 protein secondary structure estimation program (Japan Spectroscopic Co. Ltd., Tokyo, Japan).
Surface Hydrophobicity (S0)
The surface hydrophobicity of OVAs were determined by measuring the fluorescence intensity (FI) and initial slope (S0) of OVA in the presence of ANS according to the method of Hayakawa and Naka.[Citation17] Protein samples were dissolved in 10 mM phosphate buffer (pH 7.4) to give 1.0% (W/V) OVA concentration and then diluted with the same buffer for a series of five concentrations between 0.1 and 0.5%. One hundred microliters of a 0.3 g/L ANS solution in the same phosphate buffer was added to 2 mL of OVA solution as the fluorescence probe and then incubated at 25°C for 1 h. FI was measured with an LS-55 luminescence spectrophotometer (Perkin-Elmer, Waltham, Massachusetts, USA) at an excitation wavelength of 370 nm and an emission wavelength of 470 nm at 25°C. Emission spectra were corrected for background fluorescence caused by ANS in reactions lacking protein. The S0 was calculated from the FI versus protein concentration plot.
Tryptophan (Trp) Fluorescence Spectra
The protein samples were dissolved in 20 mM phosphate buffer (pH 7.4) at a protein concentration of 0.01 g/l respectively and filtered through a 0.22-μm filter. The sample solutions were scanned at emissions from 300 to 420 nm excited at a wavelength of 280 nm by an LS-55 luminescence spectrophotometer (Perkin-Elmer, Waltham, Massachusetts, USA) at 25°C.
Measurement of Solubility
Protein samples were dissolved at a concentration of 1 g/l in 50 mM Tris-HCl buffer (pH 7.0) and then centrifuged at 1000× g for 20 min. The concentration of protein in the supernatant was determined using the method of Lowry et al.[Citation18]
Measurement of Stability of OVA against Heat-Induced Insolubility
Protein samples were dissolved at a concentration of 1 g/l in 50 mM Tris-HCl buffer (pH 7.0). The sample solutions (1 ml) were placed in small test tubes with aluminium foil stoppers and heated in a water bath at 50–95°C for 10 min. Aggregates were precipitated by centrifugation at 1000× g for 20 min. The soluble protein in the supernatant was measured to estimate the protein concentration of the solution by the method of Lowry et al.[Citation18]
Measurement of Emulsifying Properties
Emulsifying properties of OVAs were measured according to the method of Pearce and Kinsella.[Citation19] One milliliter of soybean oil was added to 3 ml of 1 g/L protein sample in 0.1 mol/L phosphate buffer (pH 7.4). Subsequently, the mixture was homogenized at 10,000 rpm at 25°C for 1 min using an FA25 Model homogenizer (Fluko Equipment Shanghai Co., Ltd, China). From the bottom of a test tube, 100 μl of emulsion was taken out at different times and diluted with 3 ml of 1 g/L sodium dodecyl sulfate (SDS) solution. The absorbance of the diluted emulsion was then determined at 500 nm. The emulsifying activity was determined from the absorbance, which was measured immediately after emulsification. Emulsion stability was estimated by measuring the half-time of the turbidity, which was also measured immediately after emulsion formation.
Measurement of Water and Oil Binding Capacity
The water binding capacity (WBC) and oil binding capacity (OBC) of OVAs were measured according to the modified method of Sosulski.[Citation20] Briefly, 0.5 g protein sample (W0) of N-, DH-, and G-OVA was dispersed in 6 ml of soybean oil or deionized water in a centrifuge tube using a vortex mixer. The sample was allowed to stand at 25°C for 30 min, and the volume was recorded (V1). The dispersion was centrifuged at 3000× g for 20 min. The supernatant was carefully poured into a 10-ml graduated cylinder, and the volume was recorded (V2). WBC or OBC (ml of water or oil per gram of protein) was calculated from the formula: (V1 - V2)/W0.
Statistical Analysis
Statistical analysis was performed using SPSS (IBM, New York, NY, USA) and analysis of variance was performed by ANOVA procedures. Significant differences between mean values are determined by Fisher’s Least Significant Difference at 5% level.
RESULTS AND DISCUSSION
Characteristics of Glycated OVA
The N-OVA were glycated by dry-heating at pH 8.0 and 55°C for 3 days in the presence and absence of maltodextrin. shows the microstructure of N-OVA, DH-OVA, and G-OVA. It can be seen that the surface and edge of DH-OVA is rough, however, G-OVA are smooth and relatively regular. This result indicated that glycation might have some protective effects on protein. Compared to N-OVA, the lump of G-OVA is smaller and more homogeneous, which increases the contact area with water and might result in the improvement of functional properties of proteins, such as foaming and emulsifying properties.[Citation21]
TABLE 1 Secondary structure content, surface hydrophobicity (S0), and solubility of OVAs
FIGURE 2 Some structural and functional characteristics of OVAs. (a) CD spectra of N-, DH-, and G-OVA. Samples were dissolved in 20 mM phosphate buffer (pH 7.4) at a concentration of 0.01 g/L. (b) Tryptophan fluorescence spectra of OVAs. The excitation wavelength was 280 nm and the emission was scanned from 300 to 400 nm. Fluorescence spectra of sample were measured at 0.01 g/L in triplicate. (c) Stability against heat-induced insolubility of N-, DH-, and G-OVA at various temperatures. The protein sample was 1 g/L in 50 mmol/L Tris–HCl buffer (pH 7.0), and heated at various temperatures for 10 min. Data shown are the mean value of the two determinations, with a deviation of less that 1%. (d) Emulsifying properties given as turbidity (A500 nm) of N-, DH-, and G-OVA as a function of standing time after emulsification. The turbidity of the emulsion is plotted as the ordinate and standing time after emulsion formation as the abscissa. Each value is the mean with its SD (n = 3).
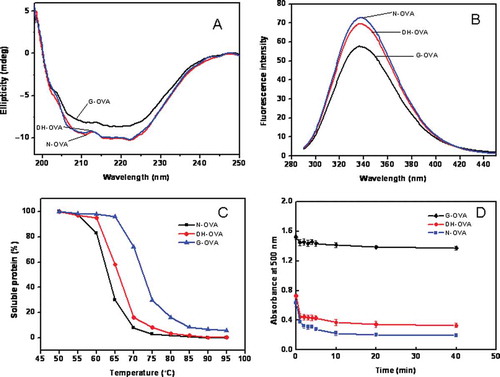
Effect of Glycation on the Structure of OVA
The CD spectroscopy was utilized to determine the impact of glycation by dry-heating in the presence of maltodextrin on the structure of the protein at the secondary folding level. shows the CD spectra of OVAs. In the present article, α-helix content for N-OVA was 30.5% (), which was close to the result reported by Batra et al.[Citation22] There was almost no change in the α-helix content of OVA by dry-heating alone. Slight changes of random coil structure and relatively significant changes of β-sheet occurred both in the absence and in the presence of maltodextrin. The β-sheet content for N-OVA was 28.1%, which was increased to 35.2% in DH-OVA and decreased to 22.6% in G-OVA. However, the overall gross structure was maintained without major change. Results suggested that the random coil structure of OVA was not significantly affected by glycation. This result was also similar to that reported in a previous study,[Citation23] wherein they showed that the random coil structure of deamidated wheat proteins was a small change from 32.8 to 35.9%. All of these results showed that the secondary structure of ovalbumin is barely affected by glycation through dry-heating. Therefore, we can conclude that dry-heating glycation reaction is a mild process since it does not disturb the secondary structure of proteins.
The effect of glycation on the surface hydrophobicity of OVA was evaluated by measuring the FI of OVA using the ANS binding method. The S0 of G-OVA was 12.8, which was 2.5-fold over that of N-OVA (). These results suggest that glycation of OVA by dry-heating in the presence of maltodextrin caused more buried hydrophobic residues to become exposed from the interior of protein rather than that of OVA dry-heated in the absence of maltodextrin. In the present study, ANS was used as a probe for the measurement of surface hydrophobicity of OVA. According to Nakai and Li,[Citation24] ANS binds to aromatic amino acid side chains from aromatic amino acids, such as Phe, Trp, and Tyr, whereas cis-parinaric acid (CPA) binds to aliphatic amino acid side chains from aliphatic amino acids, such as Val, Leu, and Ile. Hydrophobicity measured with ANS showed a significant relationship with protein solubility, while hydrophobicity measured with CPA does not. Thus, the decreased solubility of DH-OVA and G-OVA () was considered a result of increased hydrophobicity caused by dry-heating and glycation.[Citation3]
The Trp fluorescence spectra of OVA were recorded and shown in . Trp fluorescence is a sensitive index of alteration in protein conformation. The Trp fluorescence of OVA was decreased by dry-heating and was further decreased by glycation. These results suggested that conformational changes induced more exposure of tryptophan residues to solvent by glycation and showed that the tertiary structural changes occurring in OVA molecules were enhanced by glycation. Accordingly, a molten (partly unfolded) conformation may be formed by the mild changes in the random coil structure (secondary structure), while more substantial changes in hydrophobicity and Trp FI are included by glycation.
Functional Properties of G-OVA
As shown in , the soluble proteins of N- and DH-OVA decreased more quickly than did those of G-OVA as heating temperatures increased from 70 to 95°C. Results suggested that the heat stability of G-OVA is somewhat better that N- and DH-OVA. The improved heat stability of G-OVA observed in the current study may be due to the conjunction of polysaccharide and protein forming a thermostable structure, which hinders the interaction of protein during denaturation. The steric hinderance effect inhibits the aggregation of denatured protein.[Citation25]
shows the emulsifying properties of N-, DH-, and G-OVA. The value of the ordinate at 0 times denotes the relative emulsifying activity, and the half-life of initial turbidity reflects the stability of the emulsion. The turbidity value (A500 nm) of emulsion at 0 time from N-, DH-, and G-OVA were 0.68, 0.72, and 1.49, respectively, suggesting that although the emulsifying activity of OVA was scarcely affected by dry-heating in the absence of maltodextrin, it was obviously improved by glycation. Meanwhile, the half-life of initial turbidity from G-OVA was much longer than those of N- and DH-OVA, indicating that the emulsifying stability of OVA was essentially unaffected by dry-heating in the absence of maltodextrin. However, both the emulsifying capacity and emulsifying stability of G-OVA were improved, as compared to N- and DH-OVA. Improvement in emulsifying properties could be facilitated by an increase in surface hydrophobicity,[Citation26] and G-OVA may prove especially useful for food products having emulsifying capacity as their most important functional property, such as frankfurters and creams.
As shown in , in the absence of maltodextrin, the OBC of OVA slightly decreased during dry-heating. However, it was then increased by glycation. The WBC of G-OVA was 3.58 ml/g, while the values for N- and DH-OVA were 2.11 and 2.62 ml/g, respectively. This indicates that the WBC of OVA was improved by dry-heating even without maltodextrin, and was further improved by glycation. The improvement in WBC might have been caused by the exposure of additional binding sites and the excellent hydrophilic property of carbohydrate. By dry-heating in the absence of maltodextrin, the OBC of OVA was slightly decreased (2.48 ml/g). However, the OBC of G-OVA was 4.52 ml/g, which was 1.8 times higher than that of N-OVA (2.51 ml/g), suggesting that the OBC of N-OVA was obviously improved by glycation. The improvement in OBC may have been caused by the increased surface hydrophobicity after glycation, which increased the nonpolar side chains and resulted in higher absorption of oil.
TABLE 2 Some functional properties of N-, DH-, and G-OVA
CONCLUSION
This present work showed that OVA could be effectively glycated by dry-heating in the presence of maltodextrin. Molten conformations of OVA may be formed and the secondary structural change was mild during this process. Functional properties, like heat stability, emulsifying properties, oil and water binding capacity of OVA, were all remarkably improved by glycation. The attention on the structural and functional changes in this work will be contributed to the understanding of the structure-function relationship. These insights would have important implications for the creation of desirable structure and functional properties of food products.
FUNDING
This work was supported by the National Natural Science Foundation of China (No. 30871954).
Additional information
Funding
REFERENCES
- Choi, S.M.; Ma, C.Y. Structural characterization of globulin from common buckwheat (Fagopyrum esculentum Moench) using circular dichroism and Raman spectroscopy. Food Chemistry 2007, 102, 150–160.
- Alamprese, C.; Iametti, S.; Rossi, M.; Bergonzi, D. Role of pasteurisation heat treatments on rheological and protein structural characteristics of fresh egg pasta. European Food Research and Technology 2005, 221, 759–767.
- Mulsow, B.B.; Jacob, M.; Henle, T. Studies on the impact of glycation on the denaturation of whey proteins. European Food Research and Technology 2009, 228, 643–649.
- Kato, A.; Ibrahim, H.R.; Takagi, T.; Kobayashi, K. Excellent gelation of egg-white preheated in the dry state is due to the decreasing degree of aggregation. Journal of Agricultural and Food Chemistry 1990, 38, 1868–1872.
- Hellwig, M.; Henle, T. Formyline, a new glycation compound from the reaction of lysine and 3-deoxypentosone. European Food Research and Technology 2010, 230, 903–914.
- Miguel, M.; Manso, M.A.; Lopez, F.R.; Ramos, M. Comparative study of egg white proteins from different species by chromatographic and electrophoretic methods. European Food Research and Technology 2005, 221, 542–546.
- Nisbet, A.D.; Saundry, R.H.; Fothergill, L.A.; Fothergill, J.E. The complete amino-acid-sequence of hen ovalbumin. European Food Research and Technology 1981, 115, 335–345.
- Ahmed, J.; Ramaswamy, H.S.; Alli, I.; Raghavan, G.S.V. Protein denaturation, rheology, and gelation characteristics of radio-frequency heated egg white dispersions. International Journal of Food Properties 2007, 10 (1),145–161.
- Aoki, T.; Hiidome, Y.; Sugimoto, Y.; Ibrahim, H.R.; Kato, Y. Modification of ovalbumin with oligogalacturonic acids through the Maillard reaction. Food Research International 2001, 34, 127–132.
- Hu, H.Y.; Du, H.N. Alpha-to-beta structural transformation of ovalbumin: Heat and pH effects. Journal of Protein Chemistry 2000, 19, 177–183.
- Atlgan, M.R.; Unluturk, S. Rheological properties of liquid egg products (LEPS). International Journal of Food Properties 2008, 11, 296–309.
- Huntington, J.A.; Stein, P.E. Structure and properties of ovalbumin. Journal of Chromatography B 2001, 756, 189–198.
- Enomoto, H.; Nagae, S.; Hayashi, Y.; Li, C.P.; Ibrahim, H.R.; Sugimoto, Y.; Aoki, T. Improvement of functional properties of egg white protein through glycation and phosphorylation by dry-heating. Asian-Australasian Journal of Animal Sciences 2009, 22, 591–597.
- Li, C.P.; Hayashi, Y.; Shinohara, H.; Ibrahim, H.R.; Sugimoto, Y.; Kurawaki, J.; Matsudomi, N.; Aoki, T. Phosphorylation of ovalbumin by dry-heating in the presence of pyrophosphate: Effect on protein structure and some properties. Journal of Agricultural and Food Chemistry 2005, 53, 4962–4967.
- Oliveira, A.L.; Malafaya, P.B.; Costa, S.A.; Sousa, R.A.; Reis, R.L. Micro-computed tomography (mu-CT) as a potential tool to assess the effect of dynamic coating routes on the formation of biomimetic apatite layers on 3D-plotted biodegradable polymeric scaffolds. Journal of Materials Science–Materials in Medicine 2007, 18, 211–223.
- Li, C.P.; Chen, D.; Peng, J.; Enomoto, H.; Hayashi, Y.; Li, C.; Ou, L.; Aoki, T. Improvement of functional properties of whey soy protein phosphorylated by dry-heating in the presence of pyrophosphate. LWT–Food Science and Technology 2010, 43, 919–925.
- Hayakawa, S.; Nakai, S. Contribution of the hydrophobicity, net charge and sulfhydryl groups to thermal properties of ovalbumin. Candian Institute of Food Science and Technology 1985, 18, 290–295.
- Lowry, O.H.; Rosebrough, N.J.; Farr, A.L.; Randall, R.J. Protein measurement with the Folin phenol reagent. Journal of Biological Chemistry 1951, 193, 265–275.
- Pearce, K.N.; Kinsella, J.E. Emulsifying properties of proteins: Evaluation of a turbidimetric technique. Journal of Agricultural and Food Chemistry 1978, 26, 716–723.
- Sosulski, F.W. The centrifuge method for determining flour absorption in hard red spring wheats. Cereal Chemistry 1962, 39, 344–350.
- Zhang, X.C.; Tu, Z.C.; Zheng, W.W.; Li, P.; Chen, G.; Wang, H.; Dou, Y.X. Mechano-chemical effect on arachin by high pressure microfluidization. Acta Chimica Sinica 2009, 67, 2862–2866.
- Batra, P.P.; Roebuck, M.A.; Uetrecht, D. Effect of lysine modification on the secondary structure of ovalbumin. Journal of Protein Chemistry 1990, 9, 37–44.
- Benjamin, T.W.; Li, D.; Don, M.N.; Mary, A.A. The effect of maillard conjugation of deamidated wheat proteins with low molecular weight carbohydrateson the secondary structure of the protein. Food Biophysics 2009, 4, 1–12.
- Nakai, S.; Li, C.E. Hydrophobicity-functionality relationship of food proteins. In: Hydrophobic Interactions in Food Systems; CRC Press: Boca Raton, FL, 1988; 47–48.
- Fujiwara, K.; Oosawa, T.; Saeki, H. Improved thermal stability and emulsifying properties of carp myofibrillar proteins by conjugation with dextran. Journal of Agricultural and Food Chemistry 1998, 46, 1257–1261.
- Kato, A.; Ibrahim, H.R.; Watanabe, H.; Honma, K.; Kobayashi, K. New approach to improve the gelling and surface functional-properties of dryed egg-white by heating in dry state. Journal of Agricultural and Food Chemistry 1989, 37, 433–437.