Abstract
In this study, rheological and some physicochemical characteristics of some commercial hydrocolloids were determined. Principal component analysis and viscous synergism index (Iv) were used for the characterization of gums. Specific gravity and pH values of hydrocolloids were in the range of 0.431–1.010 and 5.470–9.830, respectively. Power law model was used to describe the viscous flow behavior of hydrocolloids. In general, hydrocolloids exhibited significant differences in many physicochemical characteristics (p < 0.05). When used individually, xanthan gum showed the highest K (5.131 Pa sn) compared to others. The highest K value was calculated to be 11.570 Pa sn for xanthan and guar gum mixture, which shows a synergistic interaction (Iv = 0.866), while the lowest was for tara and guar gum mixture (0.212 Pa sn), which shows an antagonistic interaction (Iv = 0.459). The n values were in the range of 0.118–0.816 and 0.098–0.619 for sole and mixture hydrocolloids, respectively. Nine physiochemical and rheological variables were reduced to two independent principal components, which accounted for 88.42% of the total variance. Moisture, pH, water holding capacity, oil holding capacity, and ash resulted in the most effective variables for the PC1 while specific gravity, consistency coefficient, and flow behavior index were useful to define the PC2.
Keywords:
1. INTRODUCTION
Hydrocolloids are one of the most important food additives used in many food formulations for various purposes, such as thickening and gelling, stabilizing, and texture modifying, as a result of their ability to alter the rheological characteristics of the formulation in which they are incorporated.[Citation1,Citation2] They are high molecular weight biopolymers with a polysaccharide structure. They strongly interact with water and they act as a thickening agent; thus, they are significantly effective on rheological properties, such as viscosity and gel formation, water holding, prevention of ice recrystallization, and sensory attributes.[Citation1,Citation3−Citation6] There are many types of hydrocolloids used for different purposes in the food industry, such as gum arabic, gum karaya, gum ghatti, and gum tragacanth obtained from the tree gum exudates; guar gum, locust bean gum, tara gum, and tamarind gum obtained from seeds of different plants; xanthan gum, curdlan, dextran, gellan gum, and cellulose obtained from microbial sources; and agar, carrageenan, and alginate obtained from algal sources.[Citation7] Different food products, such as bread, sauces, syrups, ice cream, instant foods, beverages, and ketchups, may be stabilized using various hydrocolloids. Rheological characteristics of hydrocolloids have an important role when they are used in food formulations for their effects on the textural properties. Additionally, rheology of fluid or semi-solid foods should be taken into account for designing and modeling purposes since flow behavior of these foods is very important for the process calculations, including pump sizing, extraction, or filtration.[Citation6,Citation8]
The changes in lifestyle caused a rapid increment in the consumption of ready-made meals, functional foods, and the development of high fiber and low-fat food products. Many food products in which different hydrocolloids are used as fat replacers have been recently developed. For that reason, there is a high demand for hydrocolloids in the food industry. The hydrocolloid market, which has been growing at the rate of 2 ± 3% in recent years, is valued at around $4.4 billion p.a. with a total volume of about 260,000 tonnes.[Citation7]
In food industry, mixture of hydrocolloids is generally added in the same formulation to provide a synergistic effect of the blend. It was reported that product quality can be improved and economic benefit may be gained with the use of mixed hydrocolloids.[Citation6,Citation9] For this reason, many researchers focused on the rheological characteristics of combined hydrocolloids.[Citation6,Citation9−Citation16] Principal component analysis (PCA) is a multivariate statistical method, which is a useful technique in exploratory data analysis and is widely used in food researches. Generally, PCA provides a reduction in the multivariate data by transforming it to new axes or principal components (PCs); therefore, PCA expresses the total variation in the data with only a few PCs. The greatest part of the original data variation is explained by first PC and the next greatest part of data variation is explained by a second PC and so on.[Citation17–Citation19]
The objectives of the present study were to investigate the rheological and physicochemical characteristics of commonly used food hydrocolloids and determine their synergistic effects with guar gum and establish relationship between different physicochemical and rheological characteristics using PCA method and viscous synergism index.
2 MATERIALS AND METHODS
2.1 Materials
Food hydrocolloids used in the present study were locust bean gum (Ceratonia siliqua seed), carboxymethyl cellulose (CMC) (Inallar Food Co., Turkey), xanthan gum (Berk Chemistry, Turkey), kappa-carrageenan (Biyokim Ozesen Food., Turkey), tara gum (Exandal Food Co., USA), guar gum (Kartal Chemistry, Turkey), and alginate (Ashland Inc., USA).
2.2 Methods
2.2.1 Sample preparations
To start, 0.5 g of hydrocolloid sample was weighed and incorporated slowly into 100 ml of distilled water and the solution was stirred on a hot plate (Yellowline, Germany) at 75°C for 10 min for good dissolution of the hydrocolloids. After heat treatment, the solution was cooled down to room temperature and analyzed immediately. For the mixtures, 0.25 g of guar gum and 0.25 g of other gums were weighed and the preparation process was repeated.
2.2.2 Physicochemical analysis
Moisture content of each hydrocolloid sample was determined using a rapid moisture analyzer (Sartorious, MA30, Germany). Ash content was determined according to the approved AACC method.[Citation20] pH values of samples were measured with a pH meter (Hanna, HI 120, Germany) in their solutions prepared with distilled water (10% w/v). Water holding capacity (WHC) of hydrocolloids was determined using a method described by Johnson.[Citation21] For this purpose, 1 g of hydrocolloid was suspended in 10 mL of distilled water, stirred vigorously in a vortex for 2 min, and then centrifuged (Nüve, Turkey) at 4100 rpm for 30 min. After removing the free water, the water absorbed by the samples was expressed as grams of water absorbed per 100 g of hydrocolloid. Similarly, the oil holding capacity (OHC) was determined by dispersing 1 g of hydrocolloid in 10 mL of corn oil and repeating the same procedure such as in WHC. The oil holding capacity was expressed as grams of oil absorbed per 100 g of gum.[Citation21] Water activity (aw) of samples was determined using an automatic aw-meter (Decagon, USA). Specific gravity was measured using a volumetric flask (10 ml) and the weight of a certain volume of hydrocolloid was divided by the weight of the same volume of water at 25°C.[Citation22]
2.2.3 Rheological analysis
Rheological analyses were carried out using a controlled stress/strain rheometer (Thermo-Haake, RheoStress 1, Germany) equipped with a temperature control unit (Haake, Karlsruhe K15 Germany) to characterize steady shear flow behavior of hydrocolloid solutions. A cone-plate configuration (cone diameter 35 mm, angle 4°, gap 0.140 mm) was used for the rheological measurement in the shear rate range of 5–100 s−1 at constant temperature (25°C). Then, a 0.85-ml sample was placed between the cone and plate and steady flow measurement was carried out. A total of 25 data points were recorded at 10-s intervals during shearing. Each rheological measurement was replicated five times with changing the loaded sample from the same solution with two repetitions. The apparent viscosity was recorded as a function of shear rate from the instrument software. Obtained data were fitted to power law (Eq. 1) and Herschel-Bulkley (Eq. 2) models using RheoWin Data Manager (RheoWin Pro V. 2.96, Haake, Karlsruhe, Germany) to calculate rheological parameters as follows:
For the calculation of expected viscosity of binary gum mixtures at equal concentration in a solution, Eqs. (3) and (4)[Citation23] were utilized:
Viscous synergism index (Iv) is calculated using the following expression:[Citation24]
Table 1 Some physicochemical properties of selected gum samples
2.2.4 Statistical analysis
Statistical analysis was conducted using Statistical Analysis Software package MINITAB for Windows Release 13. One-way Analysis of Variance (ANOVA) with the general linear model (GLM) procedure was used to determine the effect of independent variables on the dependent variable and Duncan Multiple Range Test was applied to observe the differences between the mean combination values using MstatC software (α = 0.05).
3 RESULTS AND DISCUSSION
3.1 Physicochemical Properties of Hydrocolloids
Some physicochemical properties of each hydrocolloid were summarized in . In general, significant differences (p < 0.05) were observed among the hydrocolloids with respect to physicochemical properties. The highest specific gravity value (1.019) was determined for alginate while the lowest was in carboxymethly cellulose (CMC) with 0.431. The specific gravity values of samples were found to be lower than unity except for alginate and differences were statistically significant (p < 0.05). The moisture content of the hydrocolloids ranged from 5.260 to 13.340%. As can be seen in , the lowest moisture content was observed in alginate, which has the highest specific gravity value. Similarly, the highest moisture contents were found in CMC gum that has the lowest specific gravity value. As is known, specific gravity is defined as the weight of the material per unit volume. For that reason, increasing of volume decreased the specific gravity. Most of hydrocolloids showed an acidic nature since their pH value was lower than 7. The highest acidity was determined in locust bean gum with a pH value of 5.470, while the lowest was in carregeenan with the pH value of 9.830. Among all samples, the pH of CMC was close to neutral pH. WHC of samples varied in a wide range depending on the type of gum. Alginate showed the highest WHC (40 g water/100 g) while the lowest was observed in guar gum. OHC values of hydrocolloids were lower compared to WHC values as expected. OHC values ranged between 0.600–1.400 g oil/100 g. Water activity of samples was determined to be rather low. All aw values were lower than 0.5. It can be said that these samples are microbiologically safe due to their low aw. The highest aw value (0.393) was determined in locust bean gum. Interesting results were obtained from ash analysis. As seen in , ash content of three samples (alginate, CMC, and carrageenan) was found to be higher than 20%. The lowest ash content was determined in guar gum whose ash content was 0.922%.
3.2 Rheological Characteristics of Hydrocolloids
illustrates the variation in apparent viscosity of hydrocolloids and their interactions with guar gum versus shear rate. As it is seen, all gum solutions showed non-Newtonian pseudoplastic behavior of which apparent viscosity decreased with increasing shear rate. The highest apparent viscosity (206.43 mPas) at 50 s−1 was measured for tara gum while the lowest (17.21 mPas) was for carregeenan at the same shear rate (). It could be inferred from and that a synergistic effect was observed between some hydrocolloids and guar gum. In general, the mixtures of xanthan gum–guar gum, locust bean gum–guar gum, alginate-guar gum, and CMC–guar gum showed considerable synergistic interaction because of Iv values of these mixed systems were calculated to be in the range of 0.5 and 1.0. Sworn[Citation25] reported that xanthan gum exhibits a synergistic interaction with galactomannans, such as locust bean gum and guar gum, and their mixtures provide an increment in the apparent viscosity compared to the viscosities of the sole components. As seen in , apparent viscosity of these mixtures was determined to be higher than that of the solution containing the sole gum. The highest apparent viscosity was observed in the mixture of xanthan gum and guar gum to be 344.17 mPas at 50 s−1 and viscous synergism index was calculated to be 0.866. However, in the mixtures of carrageenan–guar gum and tara gum–guar gum, antagonistic interaction was observed (). Incorporation of guar gum into these gum solutions provided an increment in their apparent viscosity. Equations (3) and (4) were used for the calculation of predicted apparent viscosity for mixture hydrocolloid and comparison with experimental values (). In general, a good fit was not obtained from these equations because important differences were found between computed and measured apparent viscosity values. As an example, apparent viscosity values of locust bean gum and guar gum mixture computed using Eqs. (3) and (4) were 65.18 and 11.39 mPas. However, experimental results revealed that apparent viscosity of this mixture was 117.14 mPas. Similar results were obtained from other gum mixtures. Bird et al.[Citation26] reported that Eq. (2) gives the best results when the difference between molecular weights of the components is not too great. Hui[Citation27] reported that the molecular weight of guar galactomannan is approximately 1.9 × 105 Dalton. Similarly, molecular weight of xanthan gum was reported to be 15 × 106 Dalton.[Citation25] Because of the large differences between molecular weight of gums in the present study, close results from measured and computed apparent viscosity using Eqs. (3) and (4) were not obtained for the gums used.
Table 2 Viscous synergism index values of mixed gum solutions (0.5% w/v) at constant shear rate and temperature (50 s−1 and 25°C)
Figure 1 Variation in apparent viscosity values of gum samples versus shear rate at constant temperature (25°C).
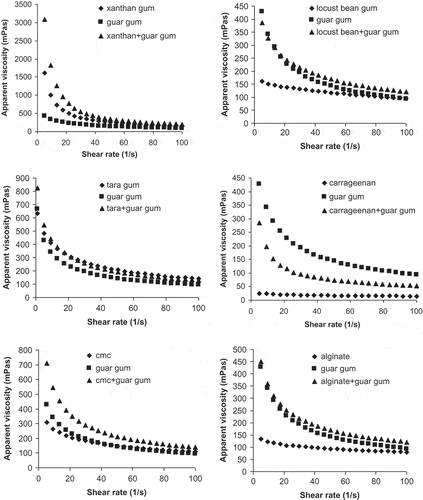
Table 3 Estimation of apparent viscosity of hydrocolloid solution using binary mixture model.a
The yield stress value of xanthan gum was comparably higher than those of other gums. Yield stress value of xanthan gum was calculated using Eq. (2) and found to be 4.07 Pa and this model showed a good fit for this gum with a high determination coefficient (0.998). It is clear that the highest apparent viscosity value was measured in the solution containing xanthan gum, while the lowest was in carregeenan (). The differences among the apparent viscosity of samples were found to be statistically significant (p < 0.05). Consistency coefficient and flow behavior index values of sole and combined gum sample solutions calculated using power law model were illustrated in . As can be seen from , the highest consistency coefficient was calculated for xanthan to be 5.131 Pa sn while the lowest consistency coefficient value (0.101 Pa sn) was in carrageenan. The consistency coefficient of tara gum was higher compared to those of guar, CMC, alginate, locust bean gum, and carrageenan. Flow behavior index values of sole hydrocolloids can be seen in . The highest flow behavior index was calculated in alginate gum to be 0.798. Flow behavior index of xanthan gum that has the highest apparent viscosity was found to be 0.118. The highest consistency coefficient values were calculated for xanthan-guar gum combination 11.570 Pa sn (), whereas tara–guar gum combinations showed the lowest consistency coefficient value (0.212 Pa sn). As stated before, an antagonistic interaction was determined between tara and guar gum when they were mixed. The lowest flow behavior index values were calculated to be 0.074 for xanthan–guar hydrocolloid mixtures. All flow behavior index values of sole or mixture hydrocolloids were calculated to be lower than unity that means they showed shear-thinning behavior.
Figure 2 Apparent viscosity values of selected gum samples at constant shear rate (50 s−1) and temperature (25°C).
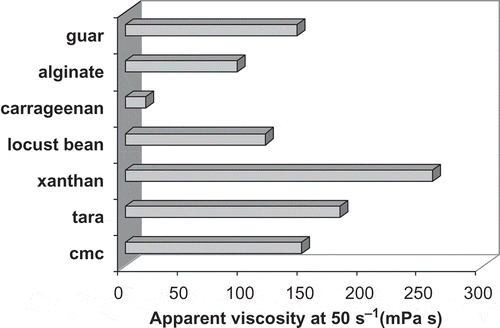
Figure 3 Variation in consistency coefficient (a) and (c) and flow behavior index (b) and (d) of selected gums at constant temperature (25°C). (a) Consistency coefficient of sole gums, (b) flow behavior index of sole gums, (c) consistency coefficient of interactions, and (d) flow behavior index of interactions.
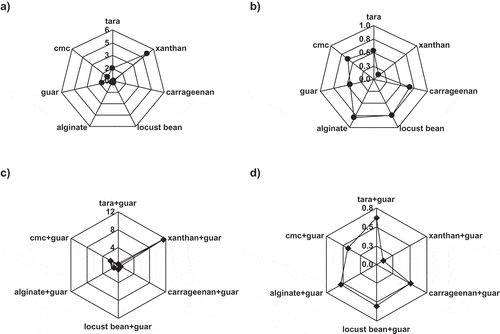
3.3 Correlation Matrix and Principal Component Analysis (PCA)
As it is seen from , there was no correlation between specific gravity and other variables. However, some parameters were highly correlated with moisture content of hydrocolloids and the correlation was statistically significant (p < 0.05). Water holding capacity and oil holding capacity of hydrocolloids were highly correlated with water content and pH and the correlation was found to be statistically significant (p < 0.05). Similarly, ash content of hydrocolloids was highly correlated with moisture content, pH, WHC, and OHC (p < 0.05). A significant negative correlation was determined between consistency coefficient and flow behavior index and as stated above, increasing consistency coefficient caused a decrease in the flow behavior index (p < 0.05).
Table 4 Correlation matrix of physicochemical and rheological characteristics of hydrocolloids
Table 5 Results from the principal component analysis for the first six principal components
Table 6 Principal component factor loadings of hydrocolloid samples
The results of PCA are presented in for hydrocolloids. The first two principal components with eigenvalues greater than 1.0 (Kaiser criterion[Citation15]) explained 88.72% of the total variation for measurements, while 60.61% of the total variance was explained by the first principal component (PC). The first PC summarizes better for the greater part of the variations in the original data matrix while the second PC explains the rest of the information better. Therefore, nine physicochemical and rheological variables were reduced to two PCs with only 11.58% variation loss. PC factor loadings and their contribution percentages in the PCs were tabulated in . The factor loadings of moisture, pH, WHC, OHC ,and ash values both in the first and second PC were similar. Consistency coefficient and flow behavior index provided higher contributions in the second PC while the most of physical parameters had the lowest value. As can be seen from the loading vectors plot (), the variables with a significant contribution are placed far from the origin of the first PC that means they are the most important variables, which can be characterized by the first PC. However, rheological parameters (K, n) are placed far from the origin of the second PC. It could be speculated that the specific gravity parameter is important for both first and second PC. Caneque et al.[Citation28] reported that the measurements and PCs are interpreted according to the correlations between each parameters and each PC. They stated that the measurements close to each other are positively correlated and measurements separated by 180° are negatively correlated and separated by 90° are independent. As it is clear in , consistency coefficient and flow behavior index separated approximately 180°, which means there is a negative correlation. The hydrocolloid samples analyzed are plotted as a function of PC1 and PC2 in . It can be seen that hydrocolloid samples are allocated in different positions from the right to the left side of the PC2 axis. Only CMC is located in the right of PC1 axes. Galactomannans, such as guar, tara, and locust bean gum, are located through the same axes due to having a similar structure. It could be said that any hydrocolloids except for CMC are represented by PC2 while CMC is characterized by PC1. , which illustrates the biplot of samples and parameters, shows those samples and their relationship with rheological and physicochemical parameters. Besides, specific gravity is located in the same region with several hydrocolloids analyzed in the present study and it could be said that specific gravity could be an important factor for characterization of hydrocolloids because the differences among the specific gravity values of samples are statistically significant (p < 0.05; ). Through PCA, two basic PCs were obtained, the first one containing moisture, WHC, OHC, and ash values and the second one consistency coefficient and water activity. In general, similar results would be obtained from the parameters that belong to the same group and this is a very useful technique to identify the different samples using fewer variables.
3.3 CONCLUSION
All hydrocolloid solutions that contain a sole or mixture of hydrocolloids showed non-Newtonian shear thinning behavior. A power law model showed good fit in describing the rheological characteristics of hydrocolloid solution with high coefficient of determination. In general, galactomannans showed lower apparent viscosity and consistency coefficient compared to microbial hydrocolloids, such as xanthan. It was determined that there were important differences among the hydrocolloids in terms of rheological and physicochemical characteristics and they can show good synergistic interaction when they mixed. Viscous synergism index is a very effective tool for the characterization of synergism or antagonism. PCA showed that two PCs could be effectively used to describe some physicochemical and rheological behavior of hydrocolloids.
REFERENCES
- Koocheki, A.; Mortazavi, S.A.; Shahidi, F.; Razavi, S.M.A.; Taherian, A.R. Rheological properties of mucilage extracted from Alyssum homolocarpum seed as a new source of thickening agent. Journal of Food Engineering 2009, 91, 490–496.
- Yaseen, E.I.; Herald, T.J.; Aramouni, F.M.; Alavi, S. Rheological properties of selected gum solutions. Food Research International 2005, 38, 111–119.
- Bai, H.M.; Ahn, J.K.; Yoon, Y.H.; Kim, H.U. A study of the development of the mixed hydrocolloid for ice cream manufacture. Korean Journal of Animal Science 1978, 20, 436–445.
- Krumel, K.L.; Sarkar, N. Flow properties of gums useful to the food industry. Food Technology 1975, 29, 36–44.
- Speers, R.A.; Tung, M.A. Concentration and temperature dependence of flow behavior of xantham gum dispersions. Journal of Food Science 1986, 51, 96–98.
- Kayacier, A.; Dogan, M. Rheological properties of some gums-salep mixed solutions. Journal of Food Engineering 2006, 72, 261–265.
- Williams, P.A.; Phillips, G.O. (Eds.). Introduction to Food Hydrocolloids, Second Edition; CRC Press: New York, 2009.
- Marcotte, M.; Hoshahili, A.R.T.; Ramaswamy, H.S. Rheological properties of selected hydrocolloids as a function of concentration and temperature. Food Research International 2001, 34, 695–703.
- Walkenström, P.; Kidman, S.; Hermansson, A.; Rasmussen, P.B.; Hoegh, L. Microstructure and rheological behaviour of xanthan/pectin mixed gels. Food Hydrocolloids 2003, 17, 593–603.
- Dunstan, D.E.; Chen, Y.; Liao, M.L.; Salvatore, R.; Boger, D.V.; Prica, M. Structure and rheology of the carrageenan/locust bean gum gels. Food Hydrocolloids 2001, 15, 475–484.
- Lundin, L.; Hermansson, A. Rheology and microstructure of Ca- and Na-K-carrageenan and locust bean gum gels. Carbohydrate Polymers 1997, 34, 365–375.
- Arnaud, L.C.; Choplin, L.; Lacrox, C. Rheological behavior of kappa-carrageenan/locust bean gum mixed gels. Journal of Texture Studies 1989, 19, 419–430.
- Stading, M.; Hermansson, A.M. Rheological behaviour of mixed gels of -carrageenan-locust bean gum. Carbohydrate Polymers 1993, 22, 49–56.
- Ahmed, J.; Ramaswamy, H.S.; Ngadi, M.O. Rheological characteristics of Arabic gum in combination with guar and xanthan gum using response surface methodology: Effect of temperature and concentration. International Journal of Food Properties 2005, 8, 179–192.
- Adamu, A.; Jin, Z.Y. Effect of chemical agents on physical and rheological properties of starch-guar gum extrudates. International Journal of Food Properties 2002, 5, 261–275.
- Mandala, I.G.; Palogou, E.D. Effect of preparation conditions and starch/xanthan concentration on gelation process of potato starch systems. International Journal of Food Properties 2003, 6, 311–328.
- Destefanis, G.; Barge, M.T.; Brugiapaglia, A.; Tassone, S. The use of principal component analysis (PCA) to characterize beef. Meat Science 2000, 56, 255–259.
- Massart, D.L.; Vandegniste, B.G.M.; Deming, S.N.; Michotte, Y.; Kaufmann, L. Principal component and factor analysis. In: Chemometrics: A Textbook; Elsevier: Amsterdam, Netherlands, 1988.
- Nguyen, T.A.; Kokot, S.; Ongarato, D.M.; Wallace, G.G. The use of chronoamperometry and chemometrics for optimization of conducting polymer sensor arrays. Electroanalysis 1999, 11, 1327–1332.
- American Association of Cereal Chemist (AACC). Approved Methods of the AACC, 9th Edition; AACC: St Paul, MN, 1995.
- Johnson, D.W. Functional properties of oil seed proteins. Journal of the American Oil Chemists’ Society 1970, 47, 402–407.
- Turabi, E.; Sumnu, G.; Sahin, S. Rheological properties and quality of rice cakes formulated with different gums and an emulsifier blend. Food Hydrocolloids 2008, 22, 305–312.
- Rao, M.A. Rheology of food gum and starch dispersions. In: Rheology of Fluid and Semisolid Foods; Rao, M.A.; Ed.; Aspen: Gaithersburg, MD, 1999; 153–218.
- Pellicer, J.; Delegido, J.; Dolz, M.; Hernández, M.J.; Herráez, M. Influence of shear rate and concentration ratio on viscous synergism. Application to xanthan-locust bean gum mixtures. Food Science and Technology International 2000, 6, 415–423.
- Sworn G. Xanthan gum. In: Handbook of Hydrocolloids, Second Edition; Philips, G.O.; Williams, P.A.; Eds.; CRC Press: New York, 2009.
- Bird, R.B.; Armstrong, R.C.; Hassager, O. Dynamics of Polymeric Liquids—Fluid Mechanics; John Wiley & Sons: New York, 1977.
- Hui, P. Unterschungen an galactomannanen, Prom. Nr. 3297, ETH. Juris Verlag: Zurich, 1962.
- Caneque, V.; Perez, C.; Velasco, S.; Diaz, M.T.; Laurizica, S.; Alvarez, I., de Huidobro, F.R., Onega, E.; la Fuente, J.D. Carcass and meat quality of light lambs using principal component analysis. Meat Science 2004, 67, 595–605.