Abstract
The performances and separation characteristics of nine widely used macroporous resins for the enrichment and purification of anthocyanins from red raspberries extracts were investigated. AB-8 resin offered the maximum adsorption and desorption behavior for anthocyanins among the resins tested, and its adsorption behavior was fitted to the Langmuir and Freundlich isotherms at different temperatures. In order to optimize the operating process, the dynamic adsorption and desorption experiments were carried out on an AB-8 resin-packed column. The optimum parameters for adsorption were sample solution anthocyanins concentration 0.2912 mg/mL, processing volume 3.5 BV, flow rate 0.5 mL/min; optimum parameters for desorption were elution solvent ethanol–water (60:40, v/v) 2.5 BV and flow rate 1.0 mL/min. After one run treatment with AB-8 resin, the anthocyanins purity increased 19.1-fold with a recovery yield of 98.84%. Two kinds of anthocyanins were obtained by further processing with a Sephadex LH-20 column, which were identified as cyanidin-3-glucoside and cyanidin-3-sophoroside using high-performance liquid chromatography–mass spectrometry, and the purity was 95.52 and 94.76%, respectively. The separation process developed via column chromatography in this study provided a potential approach for scale-up purification of anthocyanins from red raspberries or other similar berries.
INTRODUCTION
Color is usually considered as one of the most important quality attributes of foodstuff and strongly affects consumer acceptance and preference. A number of synthetic colorants have been widely used as food additives to obtain the most adequate color. However, negative perception was produced to general consumers for possible toxicity and side effects of synthetic colorants.[Citation1] Anthocyanins are a widespread source of natural water-soluble pigments and are responsible for colors ranging from pale pink to red, purple, and deep blue displayed in many fruits, vegetables, and plants.[Citation2,Citation3] Besides the colorant property, anthocyanins also have been found to contribute toward antioxidant capacities, anti-inflammatory effects, anticancer activities, antimicrobial activities, reducing eyestrain, protecting against DNA damage, etc.[Citation4Citation−Citation6] Therefore, the interest on anthocyanins for their potential application as food colorants and dietary antioxidant supplements keeps increasing in recent years.[Citation4,Citation7]
Red raspberry (Rubus idaeus L.) is an important commercial fruit with good flavor and attractive color widely grown throughout the world. Red raspberry fruits contain typical phytochemicals, such as ellagic acid, anthocyanins, gallotannins, quercetin, kaempferol-based flavonol conjugates, and trace levels of (-)-epicatechin, which show excellent biological activity.[Citation8,Citation9] Its economic significance is notable and its derived health-promoting foods and beverages are popular in China. Red raspberry is abundant in anthocyanins; the total anthocyanins content in red raspberry is 10–60 mg/100 g fresh weight, and the major anthocyanins are cyanidin-3-glucoside and cyanidin-3-sophoroside.[Citation10Citation−Citation12] Anthocyanins are normally obtained from plants by means of solid–liquid extraction with acidified methanol, ethanol, acetone, water, or mixed solvents. The use of acid stabilizes anthocyanins in the flavylium cation form, which is red at low pH.[Citation8,Citation13] Usually, the conventional solvent extraction process is time-demanding and inefficient. Moreover, thermal extraction with long time could cause the degradation of anthocyanins and decrease the antioxidant activity of the extracts.[Citation14,Citation15] Recently, a number of methods have been developed, such as microwave-assisted extraction (MAE),[Citation16] ultrasound-assisted extraction (UAE),[Citation17] and techniques based on the use of compressed fluids as extracting agents, including subcritical water extraction (SWE),[Citation18] supercritical fluid extraction (SFE),[Citation19] pressurized fluid extraction (PFE),[Citation20] or accelerated solvent extraction (ASE)[Citation21] were also applied in the extraction of anthocyanins from plant materials. In general, all of these compressed fluid-base extraction techniques are more environmental friendly procedures than other methods. However, the cost of these methods on the industrial scale is high, which often outweighs the technical benefits.[Citation22] MAE is a process utilizing microwave energy to facilitate partition analytes from the sample matrix into the extractant. Compared to conventional extraction, the main advantage of this technique is the reduced extraction time, lower solvent volume, and higher yield.[Citation16,Citation23] In our previous study, the process optimization of anthocyanin extraction from red raspberry by MAE was studied, and the effects of operating conditions, such as the ratio of solvents to materials, microwave power, and extraction time, on the extraction yield of anthocyanins were investigated through response surface methodology (RSM).[Citation24] However, in the anthocyanin-based crude extracts, there are some impurities, such as carbohydrates, Vc, and other phenolic compounds, which could affect the stability and biological activities evaluation of anthocyanins from red raspberry.[Citation10,Citation17,Citation22,Citation25] To evaluate the biological effects of anthocyanins from red raspberry, an efficient and low-cost technology for obtaining large quantities of highly purified anthocyanins from red raspberry is needed.
Preliminary enrichment and purification of active constituents from plant extracts are an important step in industrial practices. The conventional separation method of bioactive compounds is normally carried out by liquid-liquid extraction or preparative high-performance liquid chromatography (HPLC).[Citation2,Citation8] However, the former is inefficient and the latter is expensive and inconvenient to popularize.[Citation26] Column chromatography has been also employed for purification of anthocyanins, and it provides greater amounts of fractions for subsequent isolation and identification of pure substances. Typically utilized column sorbents are RP-C18,[Citation25] Toyopearl,[Citation27] macroporous adsorption resin,[Citation28] and Sephadex LH-20.[Citation29] Adsorption by macroporous resins is an efficient method to separate bioactive components from plant crude extracts because of their unique adsorption properties (higher adsorption specificities and easier desorption). Furthermore, this method has some other advantages, such as low cost, simple procedure, and high efficiency.[Citation30Citation−Citation32] So far, macroporous resins have been successfully used in the separation and enrichment of phytochemicals, such as saponins,[Citation30] paclitaxel,[Citation31] isoflavone,[Citation32] anthocyanins,[Citation28] and levan[Citation33] from natural resources. Amberlite XAD-7 and XAD-16 resins have also been widely used in purifying anthocyanins.[Citation34,Citation35] Sun et al.[Citation36] purified the anthocyanins from the extracts of red raspberry fruits through successively using Amberlite XAD-7 column chromatography, Sephadex LH-20 gel filtration and preparative HPLC. However, Amberlite XAD adsorbent resins are expensive for scale-up purification of anthocyanins. Therefore, it is necessary to find a cheap and highly efficient macroporous resin to purify the anthocyanins from red raspberry, and the purification process also required a detailed study.
TABLE 1 Physical properties of the test macroporous resins
In order to develop a simple and efficient method for preliminary enrichment of anthocyanins from extracts of red raspberry, nine resins with different polarity were used to investigate the adsorption and desorption properties of anthocyanins. The process parameters (adsorption and desorption time, flow rate, sample concentration, processing volume, and ethanol concentration and volume for desorption) based on the chosen resin were optimized. The experimental equilibrium data at different temperatures were fitted to Langmuir and Freundlich isotherms to predict the resin performances. Finally, the major anthocyanins of red raspberry, purified via column chromatography, were identified by HPLC-ESI-MS.
MATERIALS
Chemicals
Analytical-grade methanol, ethanol, acetic acid, ethyl acetate, and hydrochloric acid were purchased from Tian Li Chemicals Co., Ltd. (Tianjin, China). Chromatographic grade methanol was obtained from Concord Technology Co., Ltd. (Tianjin, China). All aqueous solutions were prepared with deionized water purified by a Milli-Q Water Purification systerm (Millipore, MA, USA). The standard sample of cyanidin-3-glucoside was supplied by the Novel Ingredient Services (New Jersey, USA) and the appropriate amounts of standards were dissolved in 0.1% hydrochloric acid solution to yield the stock solutions at the concentration of 1.0 mg/mL. All solutions prepared for HPLC-MS were filtered through 0.45-μm nylon membranes (Millipore, MA, USA) before use. Nine macroporous resins, including NKA-9, D102, S-8, D103, AB-8, D101, D4020, D3520, and D4006, were provided by the chemical plant of Nankai University (Tianjin, China). Their physical properties were summarized in . The resins were soaked in ethanol for 24 h, then were treated with 5% HCl and NaOH solutions successively to remove monomers and porogenic agents trapped inside the pores during synthesis process, and finally washed by deionized water thoroughly. The moisture contents of the tested resins were determined by drying the beads to a constant weight at 60°C under vacuum.[Citation37]
METHODS
Preparation of Red Raspberry Extracts
Red raspberry (Rubus idaeus L., Autumn Bliss) fruits were obtained from Harbin (Heilongjing, China). Anthocyanins were extracted according to the method described in our previous study.[Citation24] A portion of homogenate (50 g) was extracted with ethanol-water (70:30, v/v; 1500 mL, pH = 2) solution in a laboratory scale microwave extraction apparatus (XH-100A, Xianghu Science and Technology Development Co., Ltd., China) for 3.5 min with microwave power 400W, and repeated three times. The extraction solutions were gathered by membrane filtration (0.45 μm). The filtrates were concentrated by evaporation at 45°C with a rotary evaporator device (R-205, Shanghai Shensheng Co., Ltd., China) to eliminate the alcoholic fraction. The concentrated extracts were partitioned against ethyl acetate five times to remove liposoluble fraction and the remaining water layer was concentrated under reduced pressure at 45°C to remove a small amount of ethyl acetate. Deionized water was added to get sample solutions at the concentration range of 0.1052–0.3705 mg/mL for anthocyanins.
Static Adsorption and Desorption Tests
All macroporous resins were screened through static adsorption and desorption tests, which were performed with the following procedure: nine aliquots of 50 mL sample solutions obtained were added to 100-mL flasks containing pre-weighed amounts of different adsorbents (equal to 0.71 g dry resin). The flasks were sealed tightly and agitated at 100 rpm for 10 h in a constant temperature oscillator at 25°C. The concentrations of anthocyanins were determined by spectrophotometer analysis. After adsorption equilibrium was reached, the resins were first washed twice with deionized water, and then ethanol-water (80:20, v/v; 50 mL; pH = 2) solution was added to the 100-mL flasks containing the adsorbate-laden resins. The flasks were shaken at 100 rpm for 10 h at 25°C. The desorbed anthocyanins were determined by spectrophotometer analysis. The adsorption and desorption kinetic curves of anthocyanins on the preliminarily selected AB-8 resin and D3520 resin were studied according to the method described above. The respective concentration of anthocyanins in the sample solution after adsorption or desorption of a certain time was monitored at equal time intervals till adsorption or desorption equilibration.
Adsorption isotherms on AB-8 resin were conducted by mixing a known amount of resins (equal to 0.71 g dry weight) with sample solutions (50 mL) at different initial concentrations. The initial concentrations were 0.1052, 0.1468, 0.2023, 0.2495, 0.2912, 0.3265, and 0.3705 mg/mL for anthocyanins. After shaking for 5 h in a constant temperature oscillator at 25, 30, and 35°C, respectively, their degrees of fitness to Freundlich and Langmuir equations were evaluated. The effect of ethanol concentration on desorption was investigated using a similar procedure as described above. After static adsorption equilibrium, the resins were adequately washed with deionized water and then different concentrations of ethanol-water (v/v; 50 mL; pH = 2) solutions were added to the 100-mL flasks containing resins. The flasks were shaken at 100 rpm for 2 h and the desorbed anthocyanins were determined by spectrophotometer analysis.
Dynamic Adsorption and Desorption Tests
The dynamic adsorption and desorption tests were conducted in a glass column (15 mm × 300 mm) wet-packed with selected AB-8 resin (4.0 g dry weight). The bed volume (BV) of resin was 26.5 mL. The sample solution was pumped through the glass column at a prescribed flow rate by a peristaltic pump (ZT60-600, Baoding Lange Constant Pump Co., Ltd., Hebei, China) at 25°C and the concentration of anthocyanins in sample solution was 0.2912 mg/mL. After adsorption equilibrium at the optimized condition, the adsorbate-laden column was successively eluted by deionized water and ethanol-water (60:40, v/v; pH = 2) solution at different flow rates. The concentrations of anthocyanins in the effluents (6 mL/tube collected) were determined by spectrophotometer analysis.
Determination of Anthocyanins Concentration
Quantitative analysis of anthocyanins concentration was determined at 520 nm using a 1-cm cuvette with spectrophotometer (TU–1901; Beijing Puxi Co., Ltd., China). The anthocyanins concentration C (mg/100 mL) was calculated as A × 1000/E1% 1cm, where A is the absorbency of sample solution and E1% 1cm is the average color value of purified anthocyanins with macroporous resin and is equal to 74.8.
Determination of Anthocyanins Purity
Purity of two anthocyanins obtained by AB-8 macroporous resin and Sephadex LH-20 were determined by a pH-differential method.[Citation38] It was calculated by Eq. (1) and expressed as cyanidin-3-glucoside equivalent. All of the samples were analyzed in triplicate:
Adsorption Capacities, Adsorption and Desorption Ratio
The adsorption and desorption properties were quantified with the following equations:
Adsorption evaluation:
(2)
where qe is the adsorption capacity at adsorption equilibrium (mg/g dry resin) and E is the adsorption ratio; C0 and Ce are the initial and equilibrium concentration of analyte in the solutions, respectively (mg/mL); Vi is the volume of sample solutions(mL); and W is the weight of dry resin (g).(3)
Desorption evaluation:
(4)
where D is the desorption ratio; Cd is the concentration of the solute in the desorption solution (mg/mL); Vd is the volume of the desorption solution (mL); C0, Ce, and Vi are the same as described above.
Equilibrium Models
The Langmuir and Freundlich equations are frequently used to describe adsorption behavior between sorbent and adsorbed material. The model of Langmuir, for diluted solutions, can be expressed by the following mathematical formula:
Separation of Anthocyanins by Sephadex LH-20
After being treated with macroporous resin at the optimal conditions, Sephadex LH-20 column chromatography was used for further separation. The bed volume and the packed length of the Sephadex LH-20 column were 78.5 mL and 25 cm, respectively. The operation conditions were as follows: processing volume 0.1 BV, concentration of feed solution 0.50 mg/mL, and flow rate 0.5 mL/min; the gradient elutions were as follows: 30% methanol for 1 BV, then 50% methanol for 0.5 BV, and 70% methanol for 1.5 BV, flow rate 0.75 mL/min. The fractions were concentrated by evaporation at 45°C on a rotary evaporator to remove ethanol, and the removal of water was carried out on a freezing drier (FDU-1100, Tokyo Rikakikai Co., Ltd., Tokyo, Japan).
HPLC-ESI-MS Analysis of Anthocyanins
For structure identification, a 5-μl solution of the purified anthocyanins samples was subjected to mass spectrometry analysis using an Agilent HPLC-MS system (Agilent 1100 LC-MSD-TRAP, Agilent, USA), which was equipped with electro-spray ionization (ESI) interface. A SB-C18 (150 mm × 2.1 mm i.d., 5 μm) column fitted with a 30 mm × 2.1 mm guard column of the same material was used, and UV-Vis detection was performed at 520 nm. The mobile phase consisted of A: formic acid-water (3:97, v/v) and B: formic acid-water-methanol (3:47:50, v/v/v) with the following gradient program: first, it was operated with the ratio of 60% A to 40% B for 2 min; then within 40 min the solvent A was reduced to 0% and B was increased to 100%. The flow rate was kept at 0.8 mL/min.[Citation17]
The mass analyzer was controlled by Windows NT-based ChemStation software (version 5.2, Agilent, USA), and the mass spectrometer was operated in the positive or negative ionization mode. MS conditions were as follows: drying nitrogen gas at 350°C was used at a flow rate of 10 L/min; probe voltage 3.5 kV; capillary temperature 250°C; using full scan acquisition (m/z 100–1000 amu).
Identification of Anthocyanins
The anthocyanins were identified based on HPLC-EIS-MS spectral analysis of all the anthocyanins, and on comparison of the MS and MS2 of anthocyanins 1 with an authentic sample, and comparison of the MS of anthocyanins 2 with literature data.[Citation17,Citation29,Citation34,Citation39,Citation40]
RESULTS AND DISCUSSION
Adsorption and Desorption Ratios, Adsorption Capacity
The adsorption and desorption performances of nine macroporous resins for anthocyanins were listed in . The adsorption capacities of AB-8 and D3520 resins towards anthocyanins were higher than those of other resins. The adsorption and desorption performances correlate with the property of the resins and the chemical features of the solute.[Citation41] The weak-polar AB-8 resin and non-polar D3520 resin exhibited the best adsorption capabilities not only because of their similar polarity with the weak-polar anthocyanins, but also because of their higher surface area. In addition, AB-8 resin had a larger average pore diameter than that of D3520 resin; hence, the adsorption capacity of AB-8 resin was the higher. Compared with other resins, AB-8 and D3520 possessed better adsorption and desorption capacities for anthocyanins. As a result, adsorption and desorption kinetics experiments were carried out on AB-8 and D3520 resins.
Adsorption and Desorption Kinetics on AB-8 and D3520 Resins
Adsorption and desorption kinetics curves for anthocyanins on AB-8 and D3520 resins were shown in and . The desorption capacity of AB-8 resin towards anthocyanins was higher than that of D3050 at any time. For both resins, the adsorption capacity increased rapidly in the first 1.5 h, increased slowly after 1.5 h, and reached equilibrium at around 5 h. As for the desorption process, the equilibrium time for AB-8 resin was 1.5 h, whereas for D3520 resin it was 2 h, which indicated that the desorption speed of anthocyanins on AB-8 resin was quicker. Thus, AB-8 resin was selected as the most suitable resin and was used in further tests.
FIGURE 2 Adsorption and desorption kinetics for anthocyanins on two resins, and adsorption isotherm, dynamic breakthrough, and diffusion curves for anthocyanins on AB-8 resin. (A) Adsorption kinetics curves for anthocyanins on two resins; (B) desorption kinetics curves for anthocyanins on two resins; (C) adsorption isotherms for anthocyanins on AB-8 resin; (D) dynamic breakthrough curve for anthocyanins on AB-8 resin; (E) dynamic desorption curve for anthocyanins on AB-8 resin.
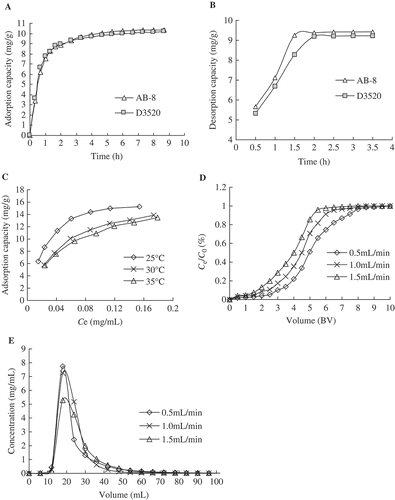
Adsorption Isotherms
Equilibrium adsorption isotherms on AB-8 resin at different temperatures for anthocyanins were obtained (). It was shown that the adsorption capacity of anthocyanins increased with the initial concentration, and reached the saturation plateau when the initial concentration of anthocyanins was 0.2912 mg/mL. The result provided a reference value for crude extract concentration before the chromatographic procedure. The Langmuir and Freundlich equations are used to reveal the linearity fitting and describe how adsorbate interacts with the resins. Generally, the Langmuir model assumes monomolecular layer adsorption with a homogeneous distribution of adsorption energies and without mutual interaction between adsorbed molecules.[Citation42] The Freundlich equation is used extensively in the physical adsorption and chemical adsorption. It can be used to describe the adsorption behavior of monomolecular layer as well as that of the multi-molecular layer. The Langmuir and Freundlich parameters were summarized in .
TABLE 2 Langmuir and Freundlich adsorption parameters of anthocyanins on AB-8 resin at different temperatures
As can be seen from , the correlation coefficients of both Langmuir and Freundlich equations for anthocyanins were rather high, and the correlation coefficient (>0.995) of the Langmuir equation was higher than that (<0.985) of the Freundlich equation, which indicated the adsorption process was a monomolecule layer adsorption. Within the ranges of temperatures investigated, the adsorption capacities decreased with the increasing temperature at the same sample concentration. Therefore, 25°C was chosen as the processing temperature in the following tests. In the Freundlich equation (Eq. 7), the adsorption can take place easily when the 1/n value is between 0.1 and 0.5, and it does not easily happen if 1/n value is between 0.5 and 1.[Citation43] As shown in , the values of 1/n are all between 0.1 and 0.5, which indicated that the adsorption on AB-8 resin could take place easily and the AB-8 resin was appropriate for separating anthocyanins.
Optimization of Ethanol Concentration
In order to choose the proper desorption solution, a different concentration of aqueous ethanol was used to perform the desorption test. The results were shown in . It could be seen that the desorption ratio increased with the increase of ethanol concentration and reached the peak value at the concentration of 60%, then decreased with the increase of ethanol concentration. Therefore, 60% aqueous ethanol was selected as the appropriate desorption solution for anthocyanins and was used in the dynamic desorption experiment.
TABLE 3 Effect of different ethanol-water solutions on the static desorption ratio of anthocyanins on AB-8 resin
Dynamic Adsorption and Desorption
Breakthrough volume was important in solid phase extraction because it represented the sample volume that could be pre-concentrated without loss of analyte during the loading of the sample. In this test, the investigated flow rate was 0.5, 1.0, and 1.5 mL/min, respectively. As shown in , at the lowest flow rate (0.5 mL/min), the best adsorption performance was obtained. Under this condition, anthocyanins in solution were almost completely adsorbed by the AB-8 resin before 3.5 BV (breakthrough point), and then the concentration of anthocyanins in leak solution increased rapidly until it reached a steady plateau in 7 BV. At the concentration of 0.2912 mg/mL, the absorption capacity was 18.20 mg/g, which was coincident with the results obtained from adsorption isotherms. Consequently, the volume of sample solution (3.5 BV) and flow rate (0.5 mL/min) were determined for the following tests.
The dynamic desorption curve on AB-8 resin was obtained based on the volume of effluent and the concentration of solute therein. As shown in , when the desorption flow rate decreased, the desorption ability of anthocyanins increased. In addition, the varying trend of different flow rates was similar. The concentration of anthocyanins reached a maximum when the volume of desorption solution (60% ethanol) was 18 mL. Furthermore, the anthocyanins completely flowed out of the column in about 66 mL (2.5 BV). However, a slower flow rate of eluent would increase the time required to run through the column. After the comprehensive consideration, 1.0 mL/min was selected as the proper desorption flow rate.
Purification of Anthocyanins
After being treated with AB-8 resin under the optimal separation conditions, the anthocyanins purity increased 19.1-fold from 4.65 to 88.83%, with a recovery yield of 98.84%. Two major fractions of anthocyanins were obtained after further purification with a Sephadex LH-20 column, and were chosen for further analysis by HPLC-ESI-MS method.
Identification of Individual Anthocyanin
Based on the literature data, individual anthocyanin in red raspberries was identified by HPLC-ESI-MS spectral analysis.[Citation18,Citation23,Citation27Citation−Citation29] MS and MS2 spectra of fraction 1 were shown in and . As could be seen, the MS analysis of fraction 1 showed a molecular anion at m/z 446.9 and a major fragmentation in MS2 at m/z 284.7, corresponding to the cyanidin aglycone, as a result of the loss of glucoside (162.2). This anthocyanin was definitively identified as cyanindin-3-glucoside by comparing its HPLC-MS spectra with an authentic standard ( and ).
FIGURE 3 Mass spectra of fraction 1 and 2. (A) MS spectra in negative mode of fraction 1; (B) MS2 spectra in negative mode of fraction 1; (C) MS spectra in negative mode of standard of cyaniding-3-glucoside; (D) MS2 spectra in negative mode of standard of cyaniding-3-glucoside; and (E) mass spectrum of fraction 2 obtained with ESI ionization.
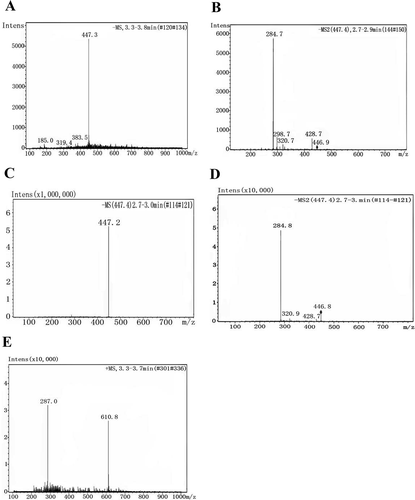
The mass chromatograph profile of fraction 2 was shown in ; it demonstrated a molecular cation (M+) at m/z = 610.8 and a fragment ion at m/z = 287.0. The fragment ion at m/z 287.0 was produced leaving the cyanidin aglycone, suggesting that the loss of sophorosyl substitute ([M–324]+) took place. Some papers also came to the same conclusion.[Citation29,Citation40] All of these evidences confirmed that fraction 2 was cyanidin-3-sophoside. The purity of cyanidin-3-glucoside and cyanidin-3-sophoroside were 95.52 and 94.76%, respectively, which was calculated by Eq. (1) and expressed as cyanidin-3-glucoside equivalents.
CONCLUSION
In the present study, AB-8 resin was the most appropriate one for purifying anthocyanins among nine resins that had been tested. The equilibrium experimental data of the adsorption of anthocyanins on AB-8 resin at different temperatures were well fitted to Langmuir and Freundlich isotherms, and a better separation power for anthocyanins on AB-8 resin was at 25°C. Furthermore, the optimal conditions, including sample concentration, flow rate, etc., were determined. The purity of anthocyanins in the extract was increased from 4.65 to 88.83% after a single run in a scale-up test. Cyanidin-3-glucoside and cyanidin-3-sophoroside were isolated and the purity was above 94%. In conclusion, the separation method developed via column chromatography provided a potential approach for scale-up purification of anthocyanins from red raspberries or other general berries.
FUNDING
The authors gratefully acknowledge the financial support by the Innovative Professional Project Foundation of Northeast Agricultural University (190205) and Scientific and Technological Research Project Foundation of Heilongjiang Provincial Education Department (12531023).
Additional information
Funding
REFERENCES
- Seeram, N.P. Berry fruits: Compositional elements, biochemical activities, and the impact of their intake on human health, performance, and disease. Journal of Agricultural and Food Chemistry 2008, 56, 627–629.
- Byamukama, R.; Kiremire, B.T.; Andersen, O.M.; Steigen, A. Anthocyanins from fruits of Rubus pinnatus and Rubus rigidus. Journal of Food Composition and Analysis 2005, 18, 599–605.
- Legua, P.; Melgarejo, P.; Martínez, J.J.; Martínez, R.; Hernández, F. Evaluation of Spanish pomegranate juices: Organic acids, sugars, and anthocyanins. International Journal of Food Properties 2012, 15, 481–494.
- Kassim, A.; Poette, J.; Paterson, A.; Zait, D.; Mccallum, S.; Woodhead, M.; Smith, K.; Hackett, C.; Graham, J. Environmental and seasonal influences on red raspberry anthocyanin antioxidant contents and identification of quantitative traits loci (QTL). Molecular Nutrition and Food Research 2009, 53, 625–634.
- Navindra, P.S.; Lynn, S.A.; Zhang, Y.J.; Rupo, L.; Daniel, S.; Henry, S.S.; David, H. Blackberry black raspberry, blueberry, cranberry, red raspberry, and strawberry extracts inhibit growth and stimulate apoptosis of human cancer cells in vitro. Journal of Agricultural and Food Chemistry 2006, 54, 9329–9339.
- Jakobet, L.; Seruga, M. Influence of anthocyanins, flavonols and phenolic acids on the antiradical activity of berries and small fruits. International Journal of Food Properties 2012, 15, 122–133.
- Verbeyst, L.; Crombruggen, K.V.; der Plancken, I.V.; Hendrickx, M.; Loey, A.V. Anthocyanin degradation kinetics during thermal and high pressure treatments of raspberries. Journal of Food Engineering 2011, 105, 513–521.
- Ancos, B.; Gonzalez, E.; Cano, M.P. Differentiation of raspberry varieties according to anthocyanin composition. Zeitschrift für Lebensmittel-Untersuchung und -Forschung 1999, 208, 33–38.
- Bordignon-Luiz, M.T.; Gauche, C.; Gris, E.F.; Falcão, L.D. Colour stability of anthocyanins from Isabel grapes (Vitis labrusca L.) in model systems. LWT–Food Science and Technology 2007, 40, 594–599.
- Horbowicz, M.; Kosson, R.; Grzesiuk, A.; Dębski, H. Anthocyanins of fruits and vegetables—Their occurrence, analysis and role in human nutrition. Vegetable Crops Research Bulletin 2008, 68, 5–22.
- Mullen, W.; Stewart, A.J.; Lean, M.E.J.; Gardner, P.; Duthie, G.G.; Crozier, A. Effect of freezing and storage on the phenolics, ellagitannins, flavonoids, and antioxidant capacity of red raspberries. Journal of Agricultural and Food Chemistry 2002, 50, 5197–5201.
- Wu, X.; Beecher, G.R.; Holden, J.M.; Haytowitz, D.B.; Gebhardt, S.E.; Prior, R.L. Concentrations of anthocyanins in common foods in the United States and estimation of normal consumption. Journal of Agricultural and Food Chemistry 2006, 54, 4069–4075.
- Huang, Z.-L.; Wang, B.-W.; Williams, P.; Pace, R.D. Identification of anthocyanins in muscadine grapes with HPLC-ESI-MS. LWT–Food Science and Technology 2009, 42, 819–824.
- Hillmann, M.C.R.; Burin, V.M.; Bordignon-Luiz, M.T. Thermal degradation kinetics of anthocyanins in grape juice and concentrate. International Journal of Food Science and Technology 2011, 46, 1997–2000.
- Robards, K. Strategies for the determination of bioactive phenols in plants, fruit and vegetables. Journal of Chromatography A 2003, 1000, 657–691.
- Pap, N.; Beszédes, S.; Pongrácz, E.; Myllykoski, L.; Gábor, M.; Gyimes, E.; Hodúr, C.; Keiski, R.L. Microwave-assisted extraction of anthocyanins from black currant marc. Food Bioprocess and Technology 2013, 6, 2666–2674.
- Chen, F.; Sun, Y.-Z.; Zhao, G.-H.; Liao, X.-J.; Hu, X.-S.; Wu, J.-H.; Wang, Z.-F. Optimization of ultrasound-assisted extraction of anthocyanins in red raspberries and identification of anthocyanins in extract using high–performance liquid chromatography– mass spectrometry. Ultrasonics Sonochemistry 2007, 14, 767–778.
- Ju, Z.; Howard, L.R. Subcritical water and sulfured water extraction of anthocyanins and other phenolics from dried red grape skin. Journal of Food Science 2005, 70, 270–276.
- Cardoso, L.C.; Serrano, C.M.; Quintero, E.T.; López, C.P.; Antezana, R.M.; de la Ossa, E.J.M. High pressure extraction of antioxidants from Solanum stenotomun peel. Molecules 2013, 18, 3137–3151.
- Truonga, V.D.; Hua, Z.; Thompsona, R.L.; Yenchob, G.C.; Pecota, K.V. Pressurized liquid extraction and quantification of anthocyanins in purple–fleshed sweet potato genotypes. Journal of Food Composition and Analysis 2012, 26, 96–103.
- Monrad, J.K.; Howard, L.R.; King, J.W.; Srinivas, K.; Mauromoustakos, A. Subcritical solvent extraction of anthocyanins from dried red grape pomace. Journal of Agricultural and Food Chemistry 2010, 58, 2862–2868.
- Dai, J.; Mumper, R.J. Plant phenolics: Extraction, analysis and their antioxidant and anticancer properties. Molecules 2010, 15, 7313–7352.
- Proestos, C.; Komaitis, M. Application of microwave-assisted extraction to the fast extraction of plant phenolic compounds. LWT–Food Science and Technology 2008, 41, 652–659.
- Yuan, X.-H.; Zhang, L.; Xu, Y.-Q.; Li, X.-G. Study on microwave-assisted extraction of pigment from red raspberry fruits optimized by response surface analysis. The Food Industry 2011, 7, 7–9.
- Bowen-Forbes, C.S.; Zhang, Y.J.; Nair, M.G. Anthocyanin content, antioxidant, anti-inflammatory and anticancer properties of blackberry and raspberry fruits. Journal of Food Composition and Analysis 2010, 23, 554–560.
- Cao, S.-Q.; Pan, S.-Y.; Yao, X.-L.; Fu, H.-F. Isolation and purification of anthocyanins from blood oranges by column chromatography. Agricultural Sciences in China 2010, 9, 207–215.
- Shoji, T.; Yanagida, A.; Kanda, T. Gel permeation chromatography of anthocyanin pigments from Rosé cider and red wine. Journal of Agricultural and Food Chemistry 1999, 47, 2885–2890.
- Chang, X.-L.; Wang, D.; Chen, B.-Y.; Feng, Y.-M.; Wen, S.-H.; Zhan, P.-Y. Adsorption and desorption properties of macroporous resins for anthocyanins from the calyx extract of roselle (Hibiscus sabdariffa L.). Journal of Agricultural and Food Chemistry 2012, 60, 2368–2376.
- Zhang, Y.; Liao, X.-J.; Chen, F.; Wu, J.-H.; Hu, X.-S. Isolation, identification, and color characterization of cyanidin-3-glucoside and cyanidin-3-sophoroside from red raspberry. European Food Research and Technology 2008, 226, 395–403.
- Jia, G.-T.; Lu, X.-Y. Enrichment and purification of madecassoside and asiaticoside from Centella asiatica extracts with macroporous resins. Journal of Chromatography A 2008, 1193, 136–141.
- Fu, Y.-J.; Zu, Y.-G.; Li, S.-M.; Sun, R.; Thomas, E.; Liu, W.; Jiang, S.G.; Luo, H.; Wang, Y. Separation of 7-xylosyl-10-deacetyl paclitaxel and 10-deacetylbaccatin III from the remainder extracts free of paclitaxel using macroporous resins. Journal of Chromatography A 2008, 1177, 77–86.
- Liu, W.; Zhang, S.; Zu, Y.-G.; Fu, Y.-J.; Ma, W.; Zhang, D.-Y.; Kong, Y.; Li, X.-J. Preliminary enrichment and separation of genistein and apigenin from extracts of pigeon pea roots by macroporous resins. Bioresource Technology 2010b, 101, 4667–4675.
- Liu, J.; Luo, J.-G.; Sun, Y.; Ye, H.; Lu, Z.-X.; Zeng, X.-X. A simple method for the simultaneous decoloration and deproteinization of crude levan extract from Paenibacillus polymyxa EJS-3 by macroporous resin. Bioresource Technology 2010, 101, 6077–6083.
- De Rosso, V.V.; Hillebrand, S.; Montilla, E.C.; Bobbio, F.O.; Winterhalter, P.; Mercadante, A.Z. Determination of anthocyanins from acerola (Malpighia emarginata DC.) and acai (Euterpe oleracea Mart.) by HPLC–PDA–MS/MS. Journal of Food Composition and Analysis 2008, 21, 291–299.
- Sadilova, E.; Stintzing, F.C.; Carle, R. Thermal degradation of acylated and nonacylated anthocyanins. Journal of Food Science 2006, 71, 504–612.
- Sun, J.-X.; Cao, X.-M.; Bai, W.-B.; Liao, X.-J.; Hu, X.-S. Comparative analyses of copigmentation of cyanidin 3-glucoside and cyanidin 3-sophoroside from red raspberry fruits. Food Chemistry 2010, 120, 1131–1137.
- Fu, Y.-J.; Zu, Y.-G.; Liu, W.; Efferth, T.; Zhang, N.-J.; Liu, X.-N.; Kong, Y. Optimization of luteolin separation from pigeonpea [Cajanus cajan (L.) Millsp.] leaves by macroporous resins. Journal of Chromatography A 2006, 1137, 145–152.
- Giusti, M.M.; Wrolstad, R.E. Current Protocols in Food Analytical Chemistry; Wrolstad, R.E.; Ed.; John Wiley & Sons: New York, NY, 2001; 59–71.
- Barritt, B.H.; Torre, L.C. Fruit anthocyanin pigments of red raspberry cultivars. Journal of the American Society for Horticultural Science 1975, 100, 98–100.
- Tian, Q.-G.; Giusti, M.M.; Stoner, G.D.; Schwartz, S.J. Screening for anthocyanins using high-performance liquid chromatography coupled to electrospray ionization tandem mass spectrometry with precursor-ion analysis, production analysis, common-neutral-loss analysis, and selected reaction monitoring. Journal of Chromatography A 2005, 1091, 72–82.
- Liu, X.-P.; Li, X.-N.; Xu, H.-X. Separation Engineering of Chinese Herb Medicine; Chemical Industry Press: Beijing, China, 2005; 95–100.
- Amara, M.; Kerdjoudj, H. Modification of the cation exchange resin properties by impregnation in polyethyleneimine solutions. Talanta 2003, 60, 991–1001.
- Treybal, R.E. Mass Transfer Operation, 3rd Ed.; McGraw-Hill Book Co.: Singapore, 1981; 590.