Abstract
Considering the importance of pasta as a food having low starch digestibility, and the global increase in diabetes, an attempt was made to develop low glycaemic spaghetti from sweet potato incorporating gums and fibers. Gums such as guar, xanthan, and locust bean were incorporated at 0.5, 1.0, and 1.5 g/100 g mix while the fibers such as apple, oat, and wheat were incorporated at 10 and 20 g/100 g mix levels along with 1.0 and 1.5 g/100 g of xanthan gum, respectively. Gum fortified spaghetti retained more protein on cooking than fiber + xanthan gum fortification. Higher swelling index was observed for gum fortified spaghetti than fiber + xanthan gum fortification, whereas cooking loss followed a reverse pattern. Lowest in vitro starch digestibility was observed for xanthan gum (1.5%) fortification, which was significantly less than the fiber + xanthan gum fortification. Resistant starch was retained to a high extent in the fortified spaghetti. The estimated glycaemic index for control spaghetti was 66.62, which could be reduced to 58–60 and 57–59 through 1.5% gum 20% fiber + xanthan gum fortification, respectively. Gums increased the firmness (N) and decreased the toughness (Ns) of dry spaghetti, while cooking drastically reduced the firmness. Formation of starch-gum-protein network was evident in the scanning electron microscopic pictures of most of the gum fortified samples. Ultrastructural studies showed that fiber + xanthan gum fortification had less firm structure of cooked spaghetti than xanthan gum alone. The study showed that guar gum and xanthan gum (1.5%) fortification can produce sweet potato spaghetti with medium glycaemic index of 58.
INTRODUCTION
Pasta or spaghetti (long pasta) is gaining popularity as a food rich in complex carbohydrates which produce slow glycaemic and postprandial insulinaemic response.[Citation1−Citation3] The low glycaemic nature of pasta has been attributed to its compact structure resulting from the formation of starch-protein network during the pasta production process.[Citation4,Citation5] Although traditionally made from durum wheat semolina, various workers have attempted fortification with non-wheat ingredients to enhance the nutritive and functional value of pasta.[Citation6−Citation9] The high incidence of metabolic diseases such as type 2 diabetes, cardiovascular conditions, and obesity among people consuming foods rich in carbohydrates has led to increased research efforts in the development of low glycaemic foods.[Citation10,Citation11] Glycaemic index (GI) is a ranking of carbohydrate rich foods, based on their relative ability to release glucose into blood.[Citation1] Sweet potato (SP; Ipomoea batatas L.) has assumed great significance in recent years as a health food due to the various bioactive principles in the roots. It is a root crop rich in starch with low GI (<55) and is reported to have anti-diabetic property and the components contributing to this effect have been isolated and studied from white-skinned SPs.[Citation12] Nevertheless, cooking methods were reported to affect the GI of SP, with baked and microwaved SP having GI of 64 and 66, respectively.[Citation13] The possibility of producing low glycaemic pasta from SP was investigated by various workers.[Citation6,Citation7,Citation14,Citation15] SP starch noodles are a popular food in China and Japan and its quality enhancement through fortification has been attempted.[Citation16,Citation17]
Hydrocolloids (gums) have been extensively used in the food industry to modify the dough properties.[Citation18−Citation20] The polymeric structure of gums enables their use as gluten substitutes, leading to a nice texture for the fortified foods.[Citation19] Gums have been reported to reduce the starch digestibility of pasta and noodles and the differential behavior of various gums such as xanthan gums (XG; glucomannan) and guar/locust bean gums (GG/LBG; galactomannan) depends on their structural features and interaction with starches.[Citation21−Citation23] GG and LBG have also been reported as hypocholesterolaemic agents and of use in the treatment of type 2 diabetes.[Citation23]
High dietary fiber diets are also implicated in the prevention of some diseases like coronary heart disease, diverticular disease, and colon cancer.[Citation24,Citation25] Soluble dietary fibers have reported hypocholesterolaemic and postprandial glucose effect in humans.[Citation26,Citation27] The effect of gums in improving the quality of SP pasta dried at low and high temperatures has been studied in our laboratory.[Citation28] Nevertheless, the cooked product had higher firmness than desirable, due to the high level of incorporation of refined wheat flour (27%). Besides, the protein content was also low, due to the high level of sweet potato flour (SPF; 70–72%). Hence, it was thought worthwhile to develop low glycaemic spaghetti from SP combining high nutritional value with low starch digestibility. Earlier studies on the effect of fortification of SPF with resistant starch (RS) sources like banana and legume in lowering starch digestibility showed that even though the in vitro starch digestibility of SP spaghetti was reduced significantly, the estimated glycaemic index (EGI) values were in the range of 62–67.[Citation29] The objectives of the present study were to compare the effect of different gums on SP spaghetti and also their synergistic effect along with dietary fiber sources in the cooking and starch digestibility characteristics.
MATERIALS AND METHODS
Materials
Freshly harvested roots of pale cream fleshed variety of SP, Sree Arun grown at the Institute Farm were selected for the study. Edible grade flour was prepared from SP as per the method reported before.[Citation29] Refined wheat flour (commercial grade) was purchased from the local market. Commercial gums such as GG, XG, and LBG were purchased from M/s Lucid Colloids Pvt. Ltd., Mumbai, India. Fiber sources such as apple fiber (AF), oat fiber (OF), and wheat fiber (WF) were purchased from M/s Rettenmaier India Pvt. Ltd., Mumbai, India. Whey protein concentrate (WPC) with a crude protein and fat content of 70 and 4.32%, respectively was purchased from M/s Mahaan Proteins Ltd., Uttar Pradesh, India. Other additives like ascorbic acid and glyceryl monostearate (GMS) used were of analytical grade.
Spaghetti Formulations
The control formulation contained SPF (71.4%), ascorbic acid (0.5%), and GMS (0.1%), besides WPC (10%), refined sunflower oil (5%) and refined wheat flour (13%). In Experiment 1, the gum sources were incorporated into the dry mixes at three levels such as 0.5, 1.0, and 1.5%, at the expense of SPF, keeping the other additives constant. Whilst the control formulation was common for Experiment 2, the fiber sources were added at two levels as shown in .
TABLE 1 Formulations for fiber enriched sweet potato spaghetti (g/100 g)
Preparation of Spaghetti
Adequate quantity of water was added to the various mixes, to ensure proper hydration so that spaghetti with smooth outer finish, without checking could be obtained by standardizing the level for each formulation and it ranged from 35–42%. The mix was extruded using the pasta making machine (P3 model from M/s La Monferrina, Italy), with the spaghetti die no. 9 (with holes having internal diameter of 0.1 mm). The extruded samples were dried overnight (18 h) at 50°C, to reduce the moisture content to less than 12%.
Cooking Characteristics
Before cooking, the spaghetti samples were uniformly dried at 105°C for 2 h. Fifty grams of dry spaghetti were added to 500 ml water containing 1.0 g NaCl. Optimum cooking time was standardized for each spaghetti using the Approved Method 66–50[Citation30] and corresponded to the disappearance of the white central core of the strands. Cooked spaghetti was drained through a strainer vessel and the water was collected in a pre-weighed dish. Swelling index (SI) was computed from the weight of the cooked spaghetti using the formula of Mestres et al.[Citation31] as:
Cooking loss (%) was determined by drying the drained water at 105°C and expressing the weight of dry residue as percentage of the original spaghetti sample.[Citation32] Protein, being the most important nutritional parameter, its level was assayed in the cooked spaghetti by the Kjeldahl method,[Citation33] in order to understand how the gums or fiber affected the protein leaching from the spaghetti.
In Vitro Starch Digestibility Kinetics
In vitro starch digestibility was monitored over a period of 2 h at 20 min intervals as per the procedure modified from the original methods of Englystet al.,[Citation34] McCleary and Monaghan,[Citation35] and Kim et al.[Citation5] Spaghetti was cooked as described above and surface dried by draining on Whatman No.1 filter paper. Cooked spaghetti (50 g) was cut into small pieces of approximately 1.0 mm length (to simulate human consumption with a low level of mastication) and samples weighing 5.0 g were mixed with HCl-KCl buffer (pH 1.5; 10.0 mL). The samples were equilibrated for 10 min at 37°C in a thermostatic water bath (SW 21; M/s Julabo Industries, Germany). Pepsin (EC 3.4.23.1; M/s SIGMA, USA) was added to each sample to start proteolysis (0.4 mL per sample from an enzyme solution containing 1.0 g pepsin in 10 mL HCl-KCl buffer). Samples were incubated for 1 h at 37°C, after which 40 mL sodium phosphate buffer (0.02 M; pH 6.9 containing 0.12 M NaCl) was added. Panzynorm-N (manufactured by M/s German Remedies India Ltd., Mumbai, India) containing 10,000 units of lipase, 9000 units of amylase, and 500 units of protease per tablet was dissolved in 5.0 mL sodium phosphate buffer (0.1 M; pH 8.0) and 1 mL each was added to the samples and incubation continued for 120 min. Enzyme and substrate blanks were maintained for each sample. Sample aliquots (1.0 mL) were drawn from the assay system after every 20 min interval up to 120 min and added to 3.0 mL sodium acetate buffer (0.2 M; pH 4.8) and incubated at 60°C for further 10 min with 0.25 mL Dextrozyme GA (M/s Novo Industries, Denmark). Glucose released in each system was quantified using the Glucose oxidase (EC 1.1.3.4) - PAP assay kit (M/S Beacon Diagnostics Pvt. Ltd., Gujarat, India). Glucose released from 20 to 120 min was expressed on 100 g starch basis, for each spaghetti sample, in order to nullify the difference in starch content between the formulations. The total starch content in the cooked spaghetti was estimated by the method of Moorthy and Padmaja,[Citation36] details of which were given earlier.[Citation14] Starch fractions such as rapidly digested starch (RDS), slowly digested starch (SDS), and RS were computed from the in vitro kinetics data of starch hydrolysis, as given below:
The hydrolysis index (HI) was calculated as:
EGI was computed using the formula of Goñi et al.[Citation37]
Instrumental Texture Profile Analysis
Textural properties of the dry as well as cooked spaghetti (Duplicate samples from two replicates) were measured using a Food Texture Analyser TAHDi (M/s Stable Microsystems, UK). Shear test/Cutting test was performed using HDP/BSK Blade set with knife and the experimental conditions were: Method: Measure force in compression; Mode: Return to start; Pre-test speed: 2 mm/s; Test speed: 2 mm/s; Post-test speed: 2 mm/s; Distance: 10 mm; Trigger force: 5.0 g. From the force-distance/time curve, the peak force is taken as the firmness (hardness; Newtons) and corresponds to the work required to shear a defined amount of pasta. The area under the curve is taken as the toughness (Newtons second).
Ultrastructure
Immediately after cooking, the samples were surface dried by blotting the adherent water on a filter paper (Whatman No. 1) and were transversely cut using a razor blade. The sample was mounted onto brass stubs using double-sided carbon conductive adhesive tape. Gold coating (10–15 nm thick) was then applied using JEOL JFC 1600 magnotron sputtering unit with 10 mA current for 80 s. The coated samples were examined at 10 kV and 1 Pa vacuum using a JEOL JSM6390 LV scanning electron microscope (Tokyo, Japan).
Statistical Analysis
The data reported were the mean of triplicate analysis and were analyzed using the statistical package SAS 9.3 to perform ANOVA.[Citation38] The treatments were considered statistically significant at 5% level (p ≤ 0.05). The mean comparisons were made by the Duncan’s Multiple Range Test (DMRT).
RESULTS AND DISCUSSION
The cooking, starch digestibility, textural, and ultrastructural characteristics of gum fortified and commercial dietary fiber fortified SP spaghetti were compared.
Protein Content
Control SP spaghetti with 10% WPC had a crude protein content of 9.9% (dwb) in the cooked sample. Nevertheless, the various gum fortified samples retained higher levels of protein in the cooked sample, resulting from lower leaching of proteins into the cooking medium and also due to the better adhesion between protein, gum, and starch. Except in LBG fortified spaghetti, higher protein levels were observed in 1.0 and 1.5% gum fortified samples (). Comparing these values with the three fiber fortified SP spaghetti, it could be seen that there was much lower crude protein levels in the treatments than gum fortified spaghetti (). This difference has mainly occurred due to the dilution of SPF because of the higher level of addition of fiber sources such 10 and 20% compared to 0.5 to 1.5% for gums and also due to the greater extent of leaching of proteins resulting from the loose starch-protein network in fiber-fortified spaghetti. WPC used in the present study (Procon 3700) had a crude protein content of 70%. The formation of a strong starch-protein network in SP pasta was established through ultrastructural studies.[Citation14] There are several reports on the effect of fortification of durum wheat semolina pasta with non-wheat ingredients in enhancing nutritional and functional value.[Citation8,Citation39,Citation40] Jyothi et al.[Citation14] reported the production of SP pasta with high protein nutritional quality, through fortification with three protein sources such as WPC, defatted soy flour and fish powder. Legume starch fortification was reported to elevate the crude protein content of SP spaghetti possibly by contributing through the starch bound protein and also by reducing the cooking loss.[Citation29] Hydrocolloids have reported effect on the pasting properties of starch in the dough, its retrogradation, etc., as well as in extending action as gluten substitutes due to their specific polymeric structure.[Citation18,Citation19,Citation41] Although the effect of hydrocolloids as gluten-substitutes has been extensively studied in bread making, relatively little studies have been made on their role in pasta and spaghetti manufacture.[Citation15,Citation21,Citation22,Citation42,Citation43] Inglett et al.[Citation22] reported no significant change in protein content in white salted and yellow alkaline noodles from wheat-rice flour blends, fortified with oat cereal hydrocolloids.
Cooking Loss and SI
The amount of residue in the cooking water expressed as cooking loss (%) is directly correlated to the cooking quality of the spaghetti. High loss of solids results in poor quality of cooked spaghetti and is due to the less firm structure of the spaghetti. The cooling loss of SP spaghetti fortified with the gum sources viz., GG, XG, and LBG was found to be significantly lower than the control at 1.0 and 1.5% levels for the former two gums and at 0.5 and 1.0% levels for LBG (). The differential behavior of the various gums could be attributed to the differences in their polymeric structure. While GG and LBG are galactomannans, XG is an anionic glucomannan.[Citation23] Christianson et al.[Citation18] found that strong association of soluble starch (amylose) occurred with GG and XG during pasting and this led to viscosity stability. It is possible that such associations taking place in the SP spaghetti during cooking might have led to the lower leaching of amylose into the cooking water. Nevertheless, higher SI resulting from the competitive hydration of gums was evident for all the gum fortified spaghetti compared to the control (). Among the gums, highest SI was observed in the case of LBG and GG-fortification (1.0 and 1.5%), which was significantly higher than XG (1.5%). Higher SI was also reported for LBG fortified SP pasta dried at 55 and 70°C.[Citation28] Higher capacity of LBG than XG and GG in retaining water in yellow layer cakes was reported by Gomez et al.[Citation19] Nevertheless, Silva et al.[Citation44] reported that hydrocolloids like LBG and GG with lower water binding capacity than XG had no significant effect on shear rheology of SP noodle dough. They also reported lower cooking loss in SP starch noodles fortified with gums.
TABLE 2 Cooking characteristics of gum and fiber fortified sweet potato spaghetti
The combined effect of fiber sources along with XG (1.0 and 1.5%) in reducing the GI of SP spaghetti was also investigated. Among the three fiber sources such as AF, OF, and WF, least cooking loss (%) was observed for OF (20%) and WF (10%) along with 1.5 and 1.0% XG, respectively, which was not significantly different from the control (). Nevertheless, the CL was higher than the gum fortified spaghetti, indicating a less firm structure when fiber is also incorporated along with the gum (). The type of additives in the pasta mix has been reported to influence the loss of soluble matter during cooking, which is more from loose textured pasta.[Citation45] Higher cooking loss (14%) was reported from SP pasta having 70% SPF and 27% refined wheat flour, having no protein additive.[Citation15] This indicates that WPC has a major role to reduce cooking loss by facilitating the formation of a firm network with starch. Kordonowy and Youngs[Citation46] reported proportionately higher CL in wheat semolina spaghetti fortified with wheat bran. Disruption of protein matrix due to incorporation of cereal fibers was reported to promote the leaching of soluble amylose into the cooking water.[Citation47] Lowering of CL on increasing the fiber levels was reported for SP pasta,[Citation15] while Chillo et al.[Citation9] reported reduced CL in wheat bran fortified durum wheat spaghetti. Significantly lower CL was observed in the present study also, when AF and OF levels were increased from 10 to 20% along with an increase in XG levels from 1.0 to 1.5% ().
Significant increases in SI were observed for spaghetti fortified with AF1 and 1.0% XG as well as in AF2 and OF2 plus 1.5% XG fortified spaghetti () as compared to the control. SI of 1.85 was reported for durum wheat semolina pasta by Tudoricăet al.,[Citation47] while fortification with pea or inulin fiber increased the SI values at 12.5 and 10% incorporation levels, respectively. SP pasta fortified with 20% wheat bran or oat bran was reported to have lower SI than the control pasta.[Citation15] Restricted swelling of starch due to the competitive hydration of fiber within the pasta matrix could lead to low SI values.[Citation47] In the present study, fiber sources have been mixed with XG and both these additives may be competing with starch for water and hence, SI values less than the gum fortified spaghetti were obtained (). Chen et al.[Citation48] observed that coarse wheat bran could retain more water than fine bran and the breakage strength of cooked wheat noodles was decreased with increase in particle size. The fibers used in the present study had particle size in the range of 31–74 μm and, therefore, did not retain high amount of water during cooking, resulting in medium SI values.
In Vitro Starch Digestibility (IVSD)
Time course release of glucose during digestion of SP spaghetti with amylase under in vitro conditions was monitored from 20–120 min. The in vitro digestion simulated the in vivo digestion with all the digestive enzymes such as pepsin, α- amylase, protease, and lipase. It was found that the various gum fortified spaghetti samples released significantly lower amounts of glucose at 20 min and the pattern continued up to 120 min. The level of gum fortification did not seem to influence the glucose release at the initial stages. Nevertheless, toward the end of the 2 h digestion period, 1.5% fortification with the three gums significantly lowered the IVSD, compared to the control as well as 0.5–1.0% fortification levels (). Among the gum sources, XG had the maximum effect in reducing IVSD and hence, this was combined with the various fiber sources in the latter part of the study.
TABLE 3 In vitro starch digestibility (g glucose released as % of total starch) of gum fortified sweet potato spaghetti
TABLE 4 In vitro starch digestibility (g glucose released as % of total starch) of sweet potato spaghetti fortified with various fiber sources
The combined effect of fiber sources along with XG (1.0 and 1.5%) in reducing IVSD was found to be less than XG alone ( vs. ). The weakening of the gluten network due to dilution of the mix with fiber (added at 10 and 20%) compared to the fortification with XG alone (low levels of 0.5–1.5%) might have led to a less firm spaghetti structure, which on cooking could increase the accessibility to extraneous α-amylase added to the in vitro system. This might have resulted in the higher glucose release from the spaghetti samples fortified with fiber and gum. The “lente” (slow release) property of carbohydrates from pasta has been reported to result from the compact starch- protein network, which restricts the entry of amylase during digestion.[Citation49−Citation51] SP is already documented as a low glycaemic (GI < 55) food.[Citation1] Fortification of SP pasta with oat and wheat bran has been reported to reduce its starch digestibility.[Citation15] We found that the time course release kinetics of glucose was lower at all points from 20 to 120 min, when the fiber levels were increased from 10–20% along with XG (1.5%) (). Similar decreases were also reported in chick pea flour fortified wheat pasta.[Citation10]
TABLE 5 Starch fractions (g per 100 g starch in cooked spaghetti on dry basis) in gum and fiber fortified sweet potato spaghetti
Quantification of the starch fractions such as RDS, SDS, and RS in the cooked spaghetti fortified with gums, showed that RDS was the lowest for 0.5% gum fortified samples, irrespective of the type of gum (). Nevertheless, the SDS was the highest for this level of fortification. The RS was the highest for the 1.5% gum fortified spaghetti and among the gums, XG gave cooked spaghetti with the highest RS content (53.52%) indicating that approximately 54% of the starch was remaining undigested after 2 h. Even though the RDS fraction was significantly lower than the control for the three fiber + XG fortified spaghetti, the values were higher than the respective 1.5% XG alone fortified spaghetti (). Further, increasing the fiber levels from 10 to 20% significantly reduced the RDS. There was also significant reduction in SDS in AF and OF fortification only when fiber and XG levels were increased to 20 and 1.5%, respectively. Nevertheless, RS values were significantly elevated for the three types of fiber-fortified spaghetti, although these values were much lower than 1.5% XG alone fortified spaghetti (). Kim et al.[Citation5] reported higher SDS values in durum wheat semolina pasta and attributed this to the firm starch-protein network, which resisted the initial digestion of starch. Pasta or spaghetti/noodles is getting wide acceptance in many countries as a health food which could slow down the glycaemic response and hence, of use in the management of type 2 diabetes and obesity.[Citation11,Citation52] RS rich foods are also reported to be beneficial in reducing the risk from coronary disease, inflammatory bowel disease, colorectal cancer, and gastrointestinal diseases.[Citation53,Citation54] Strong association between GG, XG, and soluble amylose has been demonstrated in in vitro systems.[Citation18] which has led to a decrease in the susceptibility of the complex to β-amylase. The estimate glycaemic index (EGI) of the fortified spaghetti was computed using the formula of Goni et al.[Citation37] The control SP spaghetti (cooked sample) had an EGI of 66.62, while the various gum fortified spaghetti had EGI in the range of 58.65 to 62.11 (). Among the gums, lowest EGI was obtained for 1.5% GG and XG fortified spaghetti. Nevertheless, these also fall under the category of medium EGI foods. When fiber sources were also combined with 1.5% XG, the EGI could be brought down to 57.29 in 20% OF-fortified spaghetti and 58.2 and 58.8, respectively in WF and AF fortified spaghetti (). The study showed fortification with fiber at 10 and 20% levels along with gum was not beneficial in reducing the EGI substantially, despite the dilution in starch. This is because of a less firm structure of the spaghetti, which would have permitted better accessibility of amylase, thereby degrading the starch to a greater extent than in the gum fortification alone. This could be further substantiated through textural and ultrastructural studies.
Texture of SP Spaghetti
The texture of foods is mostly determined by the moisture and fat content and the types and quantity of structural carbohydrates (cellulose, starch, and pectic materials) and proteins that are present. Changes in texture are caused by loss of moisture, fat, formation or breakdown of emulsions and gels, hydrolysis of polymeric carbohydrates, and coagulation or hydrolysis of proteins.[Citation55,Citation56] The effect of fortification of SPF with various gum and dietary fiber sources on the textural properties of spaghetti was studied. The spaghetti samples were cooked until the optimum cooking time and drained prior to analysis. Average of two different samples from each treatment, measured in triplicate is given in .
TABLE 6 Texture profile analysis of gum and fiber fortified sweet potato spaghetti
Fortification with gums increased the firmness of the dry SP spaghetti, although the type of gums as well as their fortification levels affected the firmness (). Maximum firmness was obtained at 0.5 and 1.0% levels of GG, while the least firmness (38.62; N) was obtained for 1.5% XG fortified spaghetti. On the contrary, toughness (Ns) values were considerably decreased for all the gum fortified samples, compared to the control. Nevertheless, GG fortified samples had the highest toughness as well, except LBG2 (1.0%), in the dry state. Cooking brought about drastic reduction in the firmness and toughness values. The difference observed among the gums as well as their concentrations also narrowed down on cooking (). Least toughness was observed for both dry and cooked samples from 1.0% XG fortification.
Among the various dietary fiber sources, only apple and OFs elevated the firmness of dry spaghetti at both 10 and 20% levels, compared to the control (). WF did not change the firmness, although the toughness was significantly lower than the control. Although the AF fortified spaghetti has the highest firmness and toughness in the dry state, these were reduced to a greater extent than others on cooking. The pectin associated firmness of the AF fortified dry spaghetti might have been lost due to its solubilization and leaching into water, and hence, the cooked spaghetti had a soggy texture. Silva et al.[Citation44] reported that XG at 0.5 and 1.0% levels increased the stiffness of SP starch noodles fortified with 20% broccoli powder and despite the highest water binding capacity of XG, it suppressed the swelling of starch. We also found that among the three gums, XG fortification resulted in the lowest SI. Nevertheless, the firmness of cooked spaghetti was the highest (3.85N) only at 1.5% level of XG (). Increased noodle firmness on addition of XG has also been reported.[Citation57] There are many reports on the effect of hydrocolloids on starch and certain hydrocolloids limit the gelatinization of starch during cooking through their competitive hydration ability.[Citation58,Citation59]
Bran-fortified products, despite their functional superiority are often less preferred by consumers due to the gritty mouthfeel. Therefore, in the present study we have only used fine fiber fractions with particle size in the range of 31–74 μm. The spaghetti products were without any “checking” on the surface. Earlier studies in our laboratory showed that cooked SP pasta fortified with oat bran and wheat bran had less firmness than the control pasta. Nevertheless, WPC was not an additive in these formulations and hence, even the control pasta had low firmness.[Citation15] Inglett et al.[Citation22] found that fortification of white salted and yellow alkaline noodles with 10% oat cereal hydrocolloids resulted in products having similar tensile strength as those without the fortification. Chen et al.[Citation48] reported that particle size of wheat bran significantly influenced the hardness of cooked Chinese noodles and large size bran resulted in less hard product. We found that in the case of spaghetti fortified with fibers of similar particle size, OF along with 1.5% XG gave a firmer cooked product and such combined effect of fiber and gum has not yet been reported.
Ultrastructure
The softening of the spaghetti during cooking was also substantiated through the ultrastructural studies using the scanning electron microscopy (×500 magnification) and only the pictures of the most effective combinations have been included. Thickly packed starch granules were evident in the dry samples of control spaghetti made from SP-maida blends. However, on cooking, the granules lost shape due to swelling of amylose which then leached out ( and ). Fortification with GG resulted in complex formation of gum with starch and starch granules were not visible due to such widely spread masses. On cooking, formation of several folded structures like sheets due to the formation of starch-gum-protein network were evident ( and ), which explains the restricted leaching of swollen starch into the cooking water, thereby reducing the cooking loss. The formation of the viscous starch-gum-protein layer on the surface of cooked spaghetti could prevent further leaching of amylose and this also forms a barrier for the amylases to enter and attack starch. This leads to the low in vitro starch digestibility observed in the gum fortified spaghetti.
FIGURE 4 SEM of gum fortified spaghetti: (a) GG3 Dry; (b) GG3 Cooked; (c) XG3 Dry; (d) XG3 Cooked; (e) LBG3 Dry; (f) LBG3 Cooked.
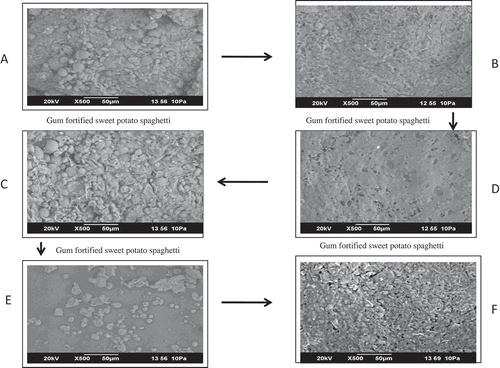
Agglomerated masses of starch-gum complexes with much space in between were evident in the dry samples of 0.5% XG fortified samples. Nevertheless, on cooking the gum as well as starch could absorb water and distribute uniformly over the surface of the spaghetti. Higher levels of fortification with XG resulted in a more uniformly spread mass in the dry samples (). Cooking resulted in swollen masses of gum and starch and granules of starch were also visible in the 0.5% and 1.0% XG fortified samples, which meant a looser complex between starch-XG-protein than the GG fortification. This is also adequately substantiated by the lower values of firmness and toughness for the XG-fortified samples. However, XG (1.5%) gave a better binding with starch, as evidenced from the SEM pictures (). Higher fortification levels of LBG (1.5%) resulted in thickly packed complexes which were evident in the cooked samples ( and ).
Christianson et al.[Citation18] found that out of the three gums such as guar, xanthan, and cellulose gums, XG had the least starch digestibility. Stronger binding of XG (1.5%) with starch as evidenced from SEM pictures of fortified SP spaghetti, observed in the present study has contributed to the least starch digestibility. Tudorică et al.[Citation47] studied the microstructure of durum wheat pasta fortified with 3% GG and found that protein-fiber complex integrated into a compact pasta matrix similar to the control pasta. These authors also reported that the reduced starch digestibility of GG enriched pasta resulted from the encapsulation of starch granules by guar. Coating of gum layer on the cooked spaghetti surface was evident in our study as well. Insoluble fiber, when incorporated into pasta are reported to disrupt the protein matrix.[Citation47] And in the present study, a better starch-fiber-protein network formation was observed, as XG (1.5%) was also combined with the three fiber sources. We have used hydrocolloids (gums) and WPC, both having reported effect of mimicking gluten,[Citation14,Citation60] and hence, the overall textural quality of the spaghetti was excellent. The functional properties of rice having 2.6 times higher RS content compared to native rice and low in vitro carbohydrate digestibility were reported by Manisha et al.,[Citation61] and the scanning electron microscopic examination revealed the continuous rigid matrix in the product.
The starch granules were widely dispersed and ruptured granules were also visible in all the fiber fortified samples (Figs. 5a–5f). The inter-granular spaces indicated only a loose ultrastructure for the dry spaghetti. Agglomerated masses of fiber-protein-starch complexes were also visible in the treatments. Cooking brought about significant alterations in the ultrastructure. In the control SP spaghetti, swollen and diffused starch granules were seen, which might have resulted from the leaching of amylose (). AF fortified spaghetti, on cooking resulted in an almost similar structure as the control spaghetti, but with more darkened spaces in between, indicative of a loose network (). Texture studies also showed that fiber fortification resulted in less firmness of cooked spaghetti. OF (20%) fortification resulted in cooked spaghetti having large complexes of starch and fiber with protein, as evidenced from the SEM () and this actually might be preventing the accessibility of amylase to starch, resulting in low starch digestibility. WF fortification also produced cooked spaghetti with total loss of granular starch structure. The starch-fiber-protein complex may form a layer over the cooked spaghetti, which might prevent the effective diffusion of amylase to hydrolyze the starch ().
CONCLUSION
The comparative effect of edible gums such as guar, xanthan, and LBGs, and dietary fibers such as apple, oat, and WFs along with XG in reducing the starch digestibility and GI of SP spaghetti was investigated. Gums at low levels of incorporation (0.5–1.5%) produced spaghetti with medium GI and XG was superior to the other gums as well as its combination with the fiber sources. While SI was enhanced through gum and (fiber + XG) fortification, cooking loss was reduced than the control only by gums. Gum fortification enhanced the firmness of dry spaghetti through better adhesion with starch. Formation of a strong starch-gum-protein network was evidenced in the gum fortified spaghetti through ultrastructural studies using scanning electron microscopy. Due to the gluten mimicking effect of gums and WPC, their synergistic action resulted in spaghetti with good textural quality. The study offers further scope in understanding the interactive mechanisms of gums with whey proteins, which could help develop low glycaemic spaghetti with GI of <55.
NOMENCLATURE
SPF | = | Sweet potato flour |
RWF | = | Refined wheat flour |
WPC | = | Whey protein concentrate |
GG | = | Guar gum |
XG | = | Xanthan gum |
LBG | = | Locust bean gum |
AF | = | Apple fiber |
OF | = | Oat fiber |
WF | = | Wheat fiber |
CL | = | Cooking loss |
DMRT | = | Duncan’s Multiple Range Test |
IVSD | = | In vitro starch digestibility |
SI | = | Swelling index |
RDS | = | Rapidly digested starch |
SDS | = | Slowly digested starch |
RS | = | Resistant starch |
SEM | = | Scanning Electron Microscopy |
ACKNOWLEDGMENTS
The facilities provided by the Director, CTCRI for the study as well as the help extended by the Sophisticated Testing & Instrumentation Centre, Cochin University of Science & Technology, Kerala in the Scanning Electron Microscopic studies are gratefully acknowledged. The authors are also thankful to Dr. J. Sreekumar, senior scientist (Agricultural Statistics), CTCRI, for helping in the statistical analysis.
FUNDING
The study was funded by a research grant (IRIS ID 2009- 06980) from the Indian Council of Medical Research, New Delhi, India for which the authors are thankful.
REFERENCES
- Björck, I.; Liljeberg, H.; Ostman, R. Low glycaemic index foods. British Journal of Nutrition 2000, 83, S149–S155.
- Giese, J.; Pasta: New twists on an old product. Food and Technology 1992, 46, 118–126.
- Malcolmson, L.J.; Matsuo, R.R.; Balshaw, R.; Textural optimization of spaghetti using response surface methodology: Effects of drying temperature and durum protein level. Cereal Chemistry 1993, 70, 417–423.
- Fardet, A.; Hoebler, C.; Baldwin, P.M.; Bouchet, B.; Gallant, D.J.; Barry, J.L.; Involvement of the protein network in the in vitro degradation of starch from spaghetti and lasagne: A microscopic and enzymic study. Journal of Cereal Science 1998, 27, 133–145.
- Kim, E.H.J.; Petrie, J.R.; Motoi, L.; Morgenstern, M.P.; Sulton, K.H.; Mishra, S.; Simmons, L.D. Effect of structural and physico-chemical characteristics of the protein matrix in pasta on in vitro starch digestibility. Food Biophysics 2008, 3, 229–234.
- Collado, L.S.; Corke, H. Use of wheat-sweet potato composite flour in yellow-alkaline and white-salted noodles. Cereal Chemistry 1996, 73, 439–444.
- Limroongreungrat, K.; Huang, Y.W. Pasta products made from sweet potato fortified with soy protein. LWT Food Science & Technology 2007, 40, 200–206.
- Manthey, F.A.; Yalla, A.R.; Dick, T.J.; Badaruddin, M.; Extrusion properties and cooking quality of spaghetti containing buckwheat bran flour. Cereal Chemistry 2004, 81, 232–236.
- Chillo, S.; Laverse, J.; Falcone, P.M.; Protopapa, A.; Del Nobile, M.A. Influence of the addition of buckwheat flour and durum wheat bran on spaghetti quality. Journal of Cereal Science 2008, 47, 144–152.
- Goñi, I.; Valentin-Gamazo, C.; Chickpea flour ingredient slows glycaemic response to pasta in healthy volunteers. Food Chemistry 2003, 81, 511–515.
- Gelencsér, T.; Gal, V.; Hodsayi, M.; Salgo, A. Evaluation of quality and digestibility characteristics of resistant starch enriched pasta. Food and Bioprocess Technology: An International Journal 2008, 1, 171–179.
- Kusano, S.; Abe, H.; Anti-diabetic activity of white-skinned sweet potato (Ipomoea Batatas L.) in obese Zucker fatty rats. Biology & Pharmacology Bulletin 2000, 23, 23–26.
- Allen, J.C.; Corbitt, A.D.; Maloney, K.P.; Bult, M.S.; Truong, V.D. Glycaemic index sweet potato as affected by cooking methods. The Open Nutrition Journal 2012, 6, 1–11.
- Jyothi, G.K.; Renjusha, M.; Padmaja, G.; Sajeev, M.S.; Moorthy, S.N. Nutritional and functional characteristics of protein-fortified pasta from sweet potato. Food and Nutrition Sciences 2011, 2, 944–955.
- Jyothi, G.K.; Renjusha, M.; Padmaja, G.; Sajeev, M.S.; Moorthy, S.N. Evaluation of nutritional and physico-mechanical characteristics of dietary fiber enriched sweet potato pasta. European Food Research and Technology 2012, 234, 467–476.
- Chen, Z. Physicochemical properties of sweet potato starches and their application in noodle products. PhD Thesis, Wageningen University: The Netherlands, 2003.
- Lee, C.H.; Cho, J.K.; Lee, S.J.; Koh, W.; Park, W.; Kim, C.H. Enhancing β-carotene content in Asian noodles by adding pumpkin powder. Cereal Chemistry 2002, 79, 593–595.
- Christianson, D.D.; Hodge, J.E.; Osborne, D.; Detroy, R.W. Gelatinization of wheat starch as modified by xanthan gum, guar gum, and cellulose gum. Cereal Chemistry 1981, 58, 513–517.
- Gomez, M.; Ronda, F.; Cabellero, P.A.; Blanco, C.A.; Rosell, C.M. Functionality of different hydrocolloids on the quality and shelf-life of yellow layer cakes. Food Hydrocolloids 2007, 21, 167–173.
- Shittu, T.A.; Aminu, R.A.; Abulude, E.O. Functional effects of xanthan gum on composite cassava-wheat dough and bread. Food Hydrocolloids 2009, 23, 2254–2260.
- Briani, G.; Bruttomesso, D.; Bilardo, G.; Giorato, C.; Duner, E.; Iori, E.; … Tiengo, A. Guar-enriched pasta and guar gum in the dietary treatment of type ii diabetes. Phytotherapy Research 2006, 1, 177–179.
- Inglett, G.E.; Peterson, S.C.; Carriere, C.J.; Maneepun, S. Rheological, textural, and sensory properties of Asian noodles containing an oat cereal hydrocolloid. Food Chemistry 2005, 90, 1–8.
- Mandala, I.; Karabela, D.; Kostaropoulos, A. Physical properties of breads containing hydrocolloids stored at low temperature. I. Effect of chilling. Food Hydrocolloids 2007, 21, 1397–1406.
- Anderson, J.W.; Smith, B.M.; Guftanson, N.S. Health benefit and practical aspects of high-fiber diets. American Journal of Clinical Nutrition 1994, 595, 1242–1247.
- Faivre, J.; Bonithon-Kopp, C. Diets, fibres, and colon cancer. Advances in Experimental Medicine and Biology 1999, 472, 199–206.
- Kahlon, T.S.; Chow, F.I. Hypocholesterolaemic effects of oat, rice, and barley dietary fibers and fractions. Cereal Food World 1997, 42, 86–92.
- Yokoyama, W.H.; Hudson, C.A.; Knuckles, B.E.; Chu, M.-C.M.; Sayre, R.N.; Tunlund, J.R.; Schneeman, B.O. Effect of barley β-glucan in durum wheat pasta on human glycemic response. Cereal Chemistry 1997, 74, 243–296.
- Jyothi, G.K. Process optimization for the production of traditional and functional pasta and flakes from sweet potato. Thesis, University of Kerala: Kerala, India, 2012; p. 313.
- Renjusha, M.; Padmaja, G.; Sajeev, M.S.; Sheriff, J.T. Effect of fortification with different starches on the starch digestibility, textural, and ultrastructural characteristics of sweet potato spaghetti. Journal of Root Crops 2012, 38, 157–167.
- AACC. Approved Methods of the American Association of Cereal Chemists, (Method 66–50). 10th Ed; St. Paul, Minnesota, USA: AACC/Eagan Press, 2000.
- Mestres, C.; Colonna, P.; Buleon, A. Characteristics of starch networks within rice flour noodles and mungbean starch vermicelli. Journal of Food Science 1988, 53, 1809–1812.
- Debbous, A.; Doctkott, C. Effect of process variables on spaghetti quality. Cereal Chemistry 1996, 73, 673–677.
- AOAC. Official Methods of Analysis, 16th Ed; Washington, DC. 1995.
- Englyst, H.N.; Veenstra, J.; Hudson, J. Measurement of rapidly available glucose (RAG) in plant foods: A potential in vitro predictor of the glycaemic response. British Journal of Nutrition 1996, 75, 327–337.
- McCleary, B.V.; Monaghan, D.A. Measurement of resistant starch. Journal of the Association of Analytical Chemists 2002, 85, 665–675.
- Moorthy, S.N.; Padmaja, G. A rapid titrimetric method for the determination of starch content of cassava tubers. Journal of Root Crops 2002, 28, 30–37.
- Goñi, I.; Garcia-Alonso, A.; Calixto, F.S. A starch hydrolysis procedure to estimate glycaemic index. Nutrition Research 1997, 17, 427–437.
- SAS/STAT Software Version 9.3, SAS Institute Inc., Cary, NC, 2010.
- Sadehi, M.A.; Bhagya, S. Quality characteristics of pasta enriched with mustard protein isolate. Journal of Food Science 2008, 73, S229–S237.
- Petitot, M.; Boyer, L.; Minier, C.; Micard, V. Fortification of pasta with split pea and faba bean flours: Pasta processing and quality evaluation. Food Research International 2010, 43, 634–641.
- Rojas, J.A.; Rosell, C.M.; Benedito B.C. Pasting properties of different wheat flour hydrocolloids systems. Food Hydrocolloids 1999, 13, 27–33.
- Collar, C.; Andreu, P.; Martinez, J.C.; Aremero, E. Optimization of hydrocolloids addition to improve wheat bread functionality: A response surface methodology study. Food Hydrocolloids 1999, 13, 467–475.
- Kim, J.C.; De Ruiter, D. Bread from non-wheat flour. Food Technology 1968, 22, 867–878.
- Silva, E.; Birkenhake, M.; Scholton, E.; Sagis, L.M.C.; Van der Linden, E. Controlling rheology and texture of sweet potato starch noodles with high broccoli powder content by hydrocolloids. Food Hydrocolloids 2013, 30, 42–52.
- Del Nobile, A.; Baiano, A.; Conte, A.; Mocci, G. Influence of protein content on spaghetti cooking quality. Journal of Cereal Science 2005, 41, 347–356.
- Kordonowy, R.K.; Youngs, V.L. Utilization of durum bran and its effect on spaghetti. Cereal Chemistry 1985, 62, 301–308.
- Tudorică, C.M.; Kuri, V.; Brennan, C.S. Nutritional and physicochemical characteristics of dietary fiber enriched pasta. Journal of Agricultural and Food Chemistry 2002, 50, 347–356.
- Chen, J.S.; Fei, M.J.; Shi, C.L.; Tian, J.C.; Sun, C.L.; Xhang, H.; … Dong, H.X. Effect of particle size and addition level of wheat bran on quality of dry white Chinese noodles. Journal of Cereal Science 2011, 53, 217–224.
- Colonna, P.; Barry, J. L.; Cloarec, D.; Bornet, F.; Gouilloud, S.; Galmiche, J.P. Enzymic susceptibility of starch from pasta. Journal of Cereal Science 1990, 11, 59–70.
- Granfeldt, Y.; Björck, I. Glycemic response to starch in pasta: A study of mechanisms of limited enzyme availability. Journal of Cereal Science 1991, 14, 47–61.
- Jenkins, D.J.A.; Wolever, T.M.S.; Jenkins, A.L.; Lee, R.; Wong, G.S.; Josse, R.G. Glycemic response to wheat products: Reduced response to pasta but no effect of fiber. Diabetes Care 1983, 6, 155–159.
- Nugent, A.P. Health properties of resistant starches. Nutrition Bulletin 2005, 61, 82–89.
- Cassidy, A.; Bingham, S.A. Colorectal cancer risk: An international comparison. British Journal of Cancer 1994, 69, 937–942.
- Tungland, B.C.; Meyer, D. Non-digestible oligo- and polysaccharides (dietary fiber): Their physiology and role in human health and food. Comprehensive Review of Food Science and Food Safety 2002, 1, 73–77.
- Fellows, P. Food Processing Technology-Principles and Practice; Woodhead Publishing Ltd.: London, 1997.
- Kindt, M.; Lercker, G.; Mazzaracchio, P.; Barbiroli, G. Effects of lipids on the quality of commercial frozen ready pasta meals. Food Control 2006, 7, 847–855.
- Brennan, C.C.; Tudorică, C.M. Fresh pasta quality as affected by enrichment of non-starch polysaccharides. Journal of Food Science 2007, 72, S 659–S665.
- Chaisawang, M.; Suphantharika, M. Pasting and rheological properties of native and anionic tapioca starches as modified by guar gum and xanthan gum. Food Hydrocolloids 2006, 20, 641–649.
- Funami, T.; Kataoka, Y.; Omoto, T.; Goto, Y.; Asai, T.; Noshinari, K. Effects of non-ionic polysaccharides on the gelatinization and retrogradation behavior of wheat starch. Food Hydrocolloids 2005, 19, 1–13.
- Lazaridou, A.; Duta, D.; Papagergiou, M.; Bele, N.; Biliaderis, C.G. Effect of hydrocolloids on dough rheology and bread quality parameters in gluten- free formulations. Journal of Food Engineering 2007, 79, 1033–1049.
- Manisha, G.; Shankar Shetty, U.; Yella Reddy, S.; Malleshi, N.G. Functional properties of slow carbohydrate digestible rice produced adapting hydrothermal treatment. International Journal of Food Properties 2011, 14, 1305–1317.