Abstract
The present study was carried out to investigate changes in fatty acids composition and their ratio by mixing of canola oil (CLO) with cold pressed stinging nettle oil (SNO) during heating at 180°C for 10 h, and analyzed by gas chromatography-mass spectrometry. The blended oils were prepared by gravimetrically mixing of CLO and SNO at various ratios of 60:40 and 40:60 w/w, respectively. Trans fatty acid in fresh CLO and SNO of 1.1 and 0.05%, whereas fresh blended oils of CLO:SNO (40:60, 60:40 w/w) contained 0.44, 0.91% and heated oils of CLO, SNO and CLO: SNO (40:60, 60:40 w/w) were found from 1.35 −2.49, 0.06 −0.09, and 0.46 −0.51, 1.02 −1.27%, correspondingly. The linolenic fatty acid is more prone to oxidation in heated oils and their percentage in fresh CLO, SNO and blended (40:60, 60:40) CLO:SNO samples of 10.58, 0.00, and 3.94, 6.64%, respectively. The linolenic acid was decreased from 10.27 −6.54, 0.00, and 3.93 −3.79%, 6.47 −5.68% in heated CLO, SNO and CLO:SNO (40:60, 60:40 w/w) oils, respectively. The oxidation parameters were also analyzed such as free fatty acids, peroxide value, iodine value, conjugated diene and triene using standard methods. The best results of free fatty acids, peroxide value, iodine value, conjugated diene and triene were obtained in blended CLO:SNO (40:60 w/w) oil at 0.31%, 0.63 meqO2/Kg, 0.79 g/100 g, 60 and 31.36% during 10 h. The obtained results show that mixing of CLO with SNO increased the stability against oxidation and consequently enhanced the worth of CLO during heating/frying route.
INTRODUCTION
Vegetable oils are an important part of the human diet and more than 90% are used in food products. Vegetable oils in the diet are a rich source of energy and contained the main fatty acids which are nutritionally vital. It is widely used for making desirable texture and unique flavors that improve the overall taste of many natural and processed foods. Edible oils are commonly used in the cooking and frying process as well as pharmaceutical, chemical, and cosmetic industries, etc.[Citation1] Vegetable oils mixed with non-edible oils, it produces a new source of edible oils which is more beneficial for health and the production of formulation due to the presence of more phytochemicals and vital antioxidative characteristics.[Citation2] Stinging nettle (Urtica dioica L.) oil has been used in the diet and as a pharmaceutical for a long time.[Citation3] Vegetable oils begin to decompose at the moment they are isolated from their natural environment when changes occur causing a disagreeable taste and smell. Atmospheric oxidation is the most important cause of deterioration in oils. This oxidative rancidity is accelerated by exposure to heat, light, humidity, and the presence of trace transition metals.[Citation4]
During frying/heating, oxidation and hydrolysis reactions takes place which leads to deterioration in vegetable oils.[Citation5−Citation7] The fatty acid composition (FAC) of fried and heated oils is an essential factor affecting the fried food taste and its stability. The changes in quality during frying are of extreme importance, as frying oil is immersed by the fried food and constitutes an important part of the diet. Free fatty acids (FFAs), peroxide value (PV), iodine value (IV), conjugated diene (CD), and conjugated triene (CT) are important oxidation parameters for determination of frying/heating oil deterioration. Polyunsaturated fatty acids (PUFAs) cannot be synthesized by the human body and can be considered as vitamin factors such as linoleic (C18:2) and linolenic (C18:3) fatty acid which are necessary for normal development and functioning of human tissues and known as essential fatty acids.[Citation8] During frying/heating, oxidation occurs in oils and capability of food get worse for eating, hence, it produced the major health effects in the human body. To improve efficiency of food during the frying process, the main components which protect the oxidation of vegetable oils are natural antioxidative compounds such as tocopherols and phenolic compounds, these antioxidative compounds are present in high amounts in non-edible oils.[Citation9] The synthetic antioxidants are not good to use in foods as compared to natural antioxidants because they produced carcinogenic actions, while natural antioxidants decreased the risks of heart disease and having anti-carcinogenic features. Subsequently they are best protector from unhealthy conditions of human beings.[Citation10, Citation11] The blending of different oils or fats has been used to produce a new type of oil or fat and their applications in food products superior due to occurrence a changes in their physicochemical properties without altering the chemical composition of oils and fats.[Citation12,] Blended oils possessed particular ingredients that are more stable against oxidation for increased the frying cycles and has a depleting effect on linolenic acid.[Citation13,Citation14] To the best of the author’s knowledge, no work has been reported on blending of canola oil (CLO) with stinging nettle oil (SNO) to enhance the stability of CLO during heating process. In the literature, there are some reports on the study of blending of canola, corn, palm, sunflower, rice bran, bene kernel oils with other edible oils, seed oils, extracts or others.[Citation15−Citation22] In the present study, it was determined that the competence of CLO with mixing of SNO (Urtica dioica L.) during heating at a constant temperature of 180°C, because CLO contained a high amount of C18:3c fatty acid and possessed a poor stability toward oxidation. Therefore, to enhance the capability of CLO, it mixed with different portions of SNO to improve the oxidative stability of CLO during heating procedure.
MATERIALS AND METHODS
Reagents and Samples
All chemicals and reagents were purchased from the E. Merck (Darmstadt, Germany). Fatty acid methyl esters (FAMEs) standards named as (GLC 607/481B) were obtained from Nu-Check Prep, Inc. (Elysian, MN, USA). Refined bleached deodorized (RBD) CLO and cold-pressed SNO were purchased from the commercial market of Konya, Turkey.
Heating Procedure and Oil Sampling
The Westpoint deep fryer of 2 L capacity (E-2016) Bordeaux, France was used for carrying out the thermal oxidation of fresh CLO, SNO, and blended oil samples at a constant temperature of 180°C for 10 h. After each 2 h heating interval, about 20 mL of the heated oil samples were placed into a screw capped vial and stored at 4°C until analysis. The total volume of oil in the fryer was 1 L, heating continued without refilling of fresh oil for 10 h period.
Determination of Fatty Acids Profile and Gas Chromatography-Mass Spectrometry (GC-MS) Conditions
The FAMEs of fresh and blended oil samples were prepared by IUPAC standard method 2.301.[Citation23] The FAMEs were determined by GC-MS using an Agilent Technologies gas chromatograph (GC-6890 N, Little Fall, NY, USA) equipped with an Agilent auto sampler 7683-B injector and an inert XL Mass selective (MS-5975) detector. For the separation of FAMEs, the capillary column of RT-2560 Bis-cyanopropylsiloxane (100 m × 0.25 mm i.d × 0.25 micron film thickness) was used. The initial temperature of 140°C was maintained for 2 min, raised to 230°C at the rate of 4°C/min and kept at 230°C for 5 min. The split ratio was 1:50, and helium was used as a carrier gas with a flow rate of 0.8 ml/min. The injector and detector temperatures were 240 and 260°C, respectively.
The mass spectrometer was operated in electron impact (EI) mode at 70eV; with ion source temp: (230°C), a quadruple temp: (150°C), and a translating line temperature of 270°C. The mass scan was found in the range between 50 and 550 m/z with an Em voltage, 1035V. Peak identification of fatty acids for the fresh and blended oil samples were performed by comparison with MS spectra and retention times (Rt) of standards. The FAMEs standards were applied to confirm the results of GC-MS libraries. For the quantitative analysis only those methyl esters were included whose matching percentage of their mass spectra were above 97% and quoted as relative percentage with respect to all methyl esters.
FFAs Analysis
The FFAs were analyzed by standard AOCS method Ca 5a-40.[Citation24] Its content as a percentage of oleic acid determined by the titration; first oil dissolved in hot neutral ethanol (C2H5OH) and followed by the titration with 0.1 N sodium hydroxide (NaOH) solutions in the presence of phenolphthalein indicator.
PV
PV was determined by AOCS official method Cd 8–53.[Citation24] The results of PV were represented as milli-equivalents of oxygen Kg−1 of oil (meqO2/Kg of oil). First, oil was dissolved in chloroform (CHCl3) and then a mixture of chloroform and glacial acetic acid (40:60v/v) was formed and allowed to react with freshly prepared potassium iodide (KI) solution in the absence of light and the free iodine (I2) was determined by titrating against standard sodium thiosulfate (0.01 M) solution using starch as an indicator.
IV
IV was determined by IUPAC official Method 2.205.[Citation23] IV showed the unsaturation degree present in fats/oils. The IV determined by Wijs method using a carbon tetrachloride as a solvent. Oil was mixed in Wijs solution and potassium iodide solution (10%). Free iodine was determined by titrating with standard sodium thiosulphate solution (0.1 M) in the presence of starch indicator.
Determination of CD and Triene
The CD and CT in fresh and blended oil samples were determined using European Communities official methods.[Citation25] For the determination of CD and CT, the oil sample (0.1 g) was dissolved in iso-octane (10 mL) and further diluted by taking 0.1 mL from prepared stock solution and 9.9 mL of iso-octane. Absorbance was measured at 232 and 270 nm.
UV-Visible Spectrophotometer
PerkinElmer (Lambda 35 Model) UV/Visible spectrophotometer has been used to record the respective absorbance value at specific wavelength, using standard 1 cm quartz cell.
SB-ATR FT-IR Measurements
All infrared (IR) spectra of oil samples were taken using the FT-IR spectrometer (Thermo Nicolet 5700, USA) fixed with a deuteratedtriglycinesulfate (DTGS) detector. The OMINIC software version 7.2 was used for data acquisition and instrument control. IR spectra were collected in the mid IR region (4000–650 cm−1) at 32 scans/sample and 4 cm−1 resolutions using a removable ZnSe SB-ATR sampling accessory (Spectra-Tech, Shelton, CT). Approximately 50 μl of oil sample poured onto the crystal for spectra recording. After each sample, the crystal was carefully cleaned with soft tissue then n-hexane, followed by acetone to remove the contamination of previous sample. Prior to recording the spectra of each sample, the new background spectrum of air was taken followed by new spectra.
Statistical Analyses
The fresh and blended oil samples (n = 2 for each) were taken two times and analysis of each sample was performed three times. The program Origin-Pro 7.5 (SRO, Origin Lab Corp., Northampton, MA, USA) was used to report the obtained data as mean (n = 2 × 3).
RESULTS AND DISCUSSION
Fatty Acids (FAs) Analysis
The representative chromatogram of SNO indicates the FAs analysis as shown in . It clearly shows the absence of linolenic acid (C18:3), which is highly responsible for oxidation of oils and decrease the stability of edible oils during the frying/heating process. The FAC of fresh (100% CLO and 100% SNO) and blended oil samples (CLO:SNO as 40:60 and 60:40 w/w) are shown in . The saturated fatty acids (SFAs) like palmitic, stearic, arachidic, and heneicosylic acids (C16:0, C18:0, C20:0, and C21:0) were found in the range of (4.73, 2.20, 3.36, 3.59, 2.06, 2.31, 2.24, 2.38, 11.33, 4.78, 0.39, 0.33, 0.22% and 0.35, 0.26, 0.27%) in CLO, SNO, CLO: SNO (40:60 and 60:40 w/w), respectively. The oleic acid (C18:1c) was major monounsaturated fatty acids (MUFAs) found in the range of 56.92, 28.26, 41.71, and 44.47% in CLO, SNO, CLO+SNO (40:60, 60:40 w/w), respectively. Among the trans MUFAs, elaidic acid (C18:1t) was present in significant amount in CLO at 1.08%, but lowest in SNO and blended CLO:SNO (40:60, 60:40 w/w) at 0.05, 0.44, and 0.90%, correspondingly. The PUFAs such as linoleic (C18:2c) was found in the range of 24.59, 66.44, 47.72, 41.52% in CLO, SNO, CLO+SNO (40:60 and 60:40 w/w), linoelaidic (C18:2t) at 0.02, 0.01 in CLO and CLO+SNO (60:40w/w), linolenic (C18:3c) was present at 10.58, 3.94, 6.64 in CLO, CLO:SNO (40:60 and 60:40 w/w) and linolenelaidic acid (C18:3t) in CLO at 0.02, respectively. From the fresh CLO, SNO, and blended oil samples, the amount of UFAs is greater than SFAs in SNO and blended oil samples. Basically, these unsaturated oils used for human diets. Moreover, these unsaturated oils reduce the occurrence of heart disease and cancer.[Citation26]
TABLE 1 Fatty acid composition (%) of fresh CLO, SNO, and blended oil samples of CLO+SNO (40:60, 60:40w/w)
FIGURE 1 Representative chromatogram of stinging nettle oil shows the absence of linolenic acid (C18:3c).
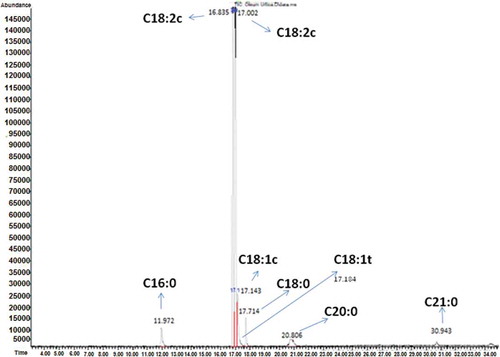
shows the FAs profile of fresh and blended oil samples during heating process from 2 to 10 h at a constant temperature of 180°C. From the results, it was observed that SFAs and TFAs were increased but UFAs was decreased throughout the heating process. The highest amount of C16:0 and C18:0 was increased in CLO and lowest was found in SNO, CLO:SNO (40:60 and 60:40 w/w) from the range of 4.90–5.87, 2.21–2.28, 3.35–3.40, 3.61–3.71%, 2.19–4.53% and 2.19–4.53, 2.33–2.42, 2.26–2.37, 2.42–2.90%, respectively after 10 h of heating period. Along with the UFAs, the MUFA (C18:1c) was decreased in CLO from the range of 56.85–56.52% but increased in SNO and CLO+SNO (40:60 and 60:40 w/w) in the range of 28.28–28.29, 41.72–41.77, 44.49–44.63%, during the heating process. The C18:1t was observed in all heated oil samples from the range of 1.28–2.18, 0.06–0.14, 0.46–0.50, and 1.01–1.23 in CLO, SNO, and CLO: SNO (40:60, 60:40 w/w). One more main class of UFAs is PUFA, in which C18:2c was found between the range of 24.44–24.05, 66.35–66.01, 47.70–47.60, 41.50–41.30% in heated oil samples of CLO, SNO, and CLO:SNO (40:60 and 60:40 w/w) but C18:3c was present in CLO and CLO:SNO (40:60 and 60:40 w/w) between the range of 10.27–6.54, 3.93–3.79, and 6.47–5.68%. The C18:2t was present in CLO and CLO:SNO (40:60 and 60:40) from the range 0.05–0.28, 0.01, 0.01–0.03% and C18:3t was determined in CLO and CLO:SNO (60:40) from the range of 0.02–0.03, 0.01%, respectively.
TABLE 2 Fatty acid composition (%) of fresh CLO, SNO, and blended oil samples of CLO+SNO (40:60, 60:40w/w) at frying temperature of 180°C
TABLE 3 Main groups and ratios between the types of fatty acids from the composition of fresh and blended oil samples of CLO and SNO at frying temperature of 180°C with different hours
represents the fatty acid groups and ratios of CLO, SNO, blended and heated oil samples of CLO:SNO (40:60 and 60:40 w/w). From the previous results, it is very clear that with the increase of heating/frying time, total SFAs were increased, while UFAs decreased. The highest percentage of SFAs after 10 h heating was determined at 53.17, 4.95, 2.75, and 10.22% in CLO, SNO, and CLO: SNO (40:60, 60:40 w/w), respectively. Similarly, decrease in percentage of UFAs were observed at 3.87, 0.27, 0.15, and 0.70% in CLO, SNO, and CLO:SNO (40:60, 60:40 w/w), respectively. The highest percentage of total SFAs were increased in CLO and also maximum percentage of total UFAs decreased in CLO. The percentage of PUFAs were decreased at 12.24, 0.57, 1.20, and 2.38% in CLO, SNO, and CLO:SNO (40:60, 60:40 w/w). PUFAs have advantageous effects on both normal health and chronic diseases, such as regulation of lipid levels.[Citation27,Citation28] The SNO and blended oil samples higher stability toward the oxidation, because in SNO the amount of C18:3c FA is totally absent but CLO contained the highest amount of C18:3c FA, therefore, oxidative stability was poor as compared to blended oils. The C18:3c FA containing oils like soybean and canola have attained the poor stability toward oxidation, prone to flavor worsening because of their high quantity of C18:3c FA.[Citation29] It has been clearly defined from the literature that reaction rate of C18:3FA with oxygen is much faster than that of C18:2 and C18:1FAs, therefore, the rate of oxidation increases in relation to the degree of PUFAs with C18:3c oxidizing twice as fast as C18:2c and 25 times faster than C18:1c.[Citation30] Oxidation of UFAs is one of major reasons for the formation of off-flavor compounds and decreased dietary values of foodstuff.[Citation31] The amount of total trans FAs (C-18:1t, C-18:2t, and C18:3t) in CLO and blended oil samples were analyzed in the range of 1.12–2.49, 0.05–0.09, 0.44–0.51, 0.91–1.27% during heating at frying temperature. The maximum percentage of TFA was increased in CLO 122.32%, due to the conversion of PUFA mostly high content of C18:3c. The mixing of CLO with SNO (40:60 and 60:40 w/w) produced the minimum percentage of TFA at 15.90 and 39.56%.
The FAs ratios of fresh and heated oil samples are shown in . The ratio of SFA/UFA indicates the association among two major FA groups. The amount of SFA/UFA ratio in CLO, SNO, and CLO:SNO (40:60, 60:40 w/w) at 58.90, 5.45, 3.03, and 11.59 was increased during heating process. The highest percentage was observed in CLO at 58.90, which shows a high amount of SFA formed during heating process. The lowest percentage of SFA/UFA was observed in blended CLO:SNO (40:60 w/w) at 3.03, it means a low proportion of SFA obtained, this is considered good for dietary value of an oil. The smaller ratio of SFA/UFA is considered being positive from the nutritional point of view.[Citation32] The linoleic and linolenic to palmitic acid ratios (C18:2/C16:0 and C18:3/C16:0), are considered for level of deterioration.[Citation33−Citation35] The present study shows a decreased pattern in C18:2/C16:0 and C18:3/C16:0 ratios of the analyzed samples. The C18:2/C16:0 and C18:3/C16:0 ratios increased in percentage of 20.38, 4.07, 1.40, 4.23, and 50, 00, 5.12, 17.29 in CLO, SNO and CLO:SNO (40:60, 60:40 w/w). The maximum percentage of C18:2/C16:0 and C18:3/C16:0 ratio was observed in CLO and lowest in blended of CLO:SNO (40:60 w/w). The C18:3/C16:0 is faster reducing in CLO because it contained the high amount of C18:3c FA. The mixing of CLO with SNO has possessed the good oxidative stability especially more in the ratio of (40:60 w/w). The C18:3/C16:0 was faster reducing than C18:2/C16:0,[Citation33] this same behavior was observed in the present study.
FT-IR Study
The CLO and SNO were differentiated by FT-IR spectroscopy through different peaks related to special functional groups. The major components of fresh CLO, SNO, and 10 h heated oil samples are well differentiated in the representative FT-IR spectrum (). The common peaks present in CLO and SNO are given as. The band at 3000–3010 cm−1 is due to the CH stretching vibration of the cis-double bond (–C=H) of all unsaturated acyl group chains of FAs. The bands appear at 2922 and 2853 cm−1 corresponding to the asymmetric and symmetric stretching bands of aliphatic (–CH3 and –CH2) groups present in the acyl group chains.[Citation36] The carbonyl –C=O (ester) group of triglycerides shows a stretching vibration band at 1744 cm−1. Disubstituted cis-olefins (–C=C–) absorb at about 1652 cm−1and the absorption of this band is stronger than that of trans-olefins.[Citation37] For this reason, the disubstituted cis-olefins band can be attributed to C=C stretching vibration of disubstituted cis C=C of acyl groups of C18:1 and C18:2.[Citation36] The bending vibration (scissoring) of the methylene group at 1463 cm−1, could be attributed to the vibration of –CH bands of cis-disubstituted olefins.[Citation36,Citation38] The band at 1376 cm−1 is due to the symmetrical bending vibration of –C–H (CH3) methyl groups. The bending and stretching vibration of C–O and methylene bands in esters group appear at 1236 and 1158 cm−1,[Citation39] while C–O bending vibrations observed at 1118 and 1098 cm−1. The band at 1031 cm−1 represents the stretching vibrations of –C–O group in esters,[Citation37] whereas bands at 960–970 and 845 cm−1 are the characteristics of –HC–– CH– (trans) out of plane vibrations. The bands at 700–725cm−1 result from the overlapping of the methylene rocking vibration and the out-of-plane bending vibration of cis-disubstituted olefins.[Citation36] To further check the stability of oils toward an oxidation with reference to heating in CLO, SNO, and blended oils CLO:SNO (40:60, 60:40 w/w), the other quality parameters were analyzed such as FFAs, PV, IV, CD, and CT.
FFAs
FFAs are used for measure the quantity of FAs hydrolyzed on the triacylglycerols backbone. FFAs of fresh and blended oil samples are shown in . From the results, it was observed that as heating process get longer, FFAs significantly increased in CLO, SNO, and blended oil samples. Higher amounts of FFAs were observed in CLO, SNO, CLO:SNO (60:40 w/w) at 1.62, 0.68, 0.44% and lesser in CLO:SNO (40:60 w/w) at 0.31% during frying process. The formation of FFAs in heated or fried oil samples, it may be due to the oxidation and thermal degradation taking place in UFAs.[Citation40]
PV
PV is used to determine the hydroperoxide in oils and fats which measure the primary oxidation.[Citation41] shows the PV of fresh and blended oil samples at frying temperature of 180°C. PV was increased throughout the heating process with respect to time in CLO, SNO, and blended (40:60 and 60:40 w/w) oil samples. Higher amounts of PV was observed in CLO, SNO, CLO: SNO (60:40 w/w) at 3.19, 0.92, 0.94 meqO2/Kg and lesser amount in CLO: SNO (40:60 w/w) at 0.63 meqO2/Kg, respectively, during the 10 h heating process. From the previous studies, it has been clearly defined that PV was more increased in which oil that has possess the more amount of C18:3c FA,[Citation42,Citation43] this study also showed the same behavior with the reported one.
IV
IV is a quality parameter, it shows a presence of unsaturation in an oils and fats.[Citation44] shows IV of CLO, SNO, and blended oils during heating procedure at 180°C. The IV of CLO, SNO and blended CLO:SNO (40:60 and 60:40 w/w) oils in the range of 107.23–104.65, 99.42–99.08, 101.89–101.08, and 103.45–102.39 g/100 g, respectively. IV indicated the decreasing manner due to the destruction of double bonds in the presence of oxidation and polymerization process. The IV decreases with an increase of time could be attributed to the changes occurred in FAs during frying/heating process.[Citation45]
CD and CT
The changes in CD and CT values of CLO, SNO, and blended oil samples during heating at 180°C are shown in and . The values of CD and CT increased from the range of 3.01–10.56, 1.76–5.14, 1.28–3.22, 1.43–4.56 mmol/L and 1.76–2.87, 0.73–1.43, 0.81–1.18, 0.92–1.51 mmol/L in CLO, SNO, and CLO:SNO (40:60 and 60:40 w/w).
CONCLUSION
The main results of present research work indicated that blending of CLO with different division of SNO is a novel source of oil for frying purpose with high amount of MUFAs, lower in linoleic and especially less amount of linolenic fatty acids. The results show that changes of FAC and other oxidation parameters for quality evaluation were controlled and considerably reduced in CLO:SNO (40:60 w/w). Proposed blended oils had superior stability against oxidation for more heating/frying cycles at frying temperature of 180°C.
FUNDING
The authors would like to thank the Scientific and Technological Research Council of Turkey (TUBITAK) under the 2216 Research Fellowship Program for Foreign Citizens and YOK, Turkey research program for providing the financial support to carry out this research work.
REFERENCES
- Dugo, G.; Pera, L.; Torre, G.L.; Giuffrida, D. Determination of Cd (II), Cu (II), Pb (II), and Zn (II) content in commercial vegetable oils using derivative potentiometric stripping analysis. Food Chemistry 2004, 87, 639–645.
- Ramadan, M.F.; Moersel, J.T. Screening of the anti-radical action of vegetable oils. Journal of Food Composition and Analysis 2006, 19, 838–842.
- Guil-Guerrero, J.L.; Rebollose-Fuentes, M.M.; Isasa, M.E. Fatty acids and carotenoids from stinging nettle (Urtica dioica L.). Journal of Food Composition and Analysis 2003, 16, 111–119.
- Primo Yufera, E.; Quımica Agrıcola III, Alimentos, 1st Ed; Alhambra: Madrid, Spain, 1979.
- Paul, S.; Mittal, G.S.; Chinnan, M.S. Regulating the use of degraded oil/fat in deep-fat/oil food frying. Critical Reviews in Food Science and Nutrition 1997, 37, 635–662.
- Romero, A.; Cuesta, C.; Sánchez-Muniz, F.J. Trans fatty acid production in deep fat frying of frozen foods with different oils and frying modalities. Nutrition Research 2000, 20, 599–608.
- Romero, A.; Cuesta, C.; Sánchez-Muniz, F.J. Cyclic fatty acid monomers in high oleic acid sunflower oil and extra virgin olive oil used in repeated frying of fresh potatoes. Journal of the American Oil Chemists’ Society 2003, 80, 437–442.
- Kamal-Eldin, A.; Appelqvist, L.A. Aldehydic acids in frying oils: Formation, toxicological significance, and analysis. Grasas Y Aceites 1996, 47, 342–348.
- Blazevic, I.; Mastelic, J. Glucosinolate degradation products and other bound and free volatiles in the leaves and roots ofradish (Raphanussativus L.). Food Chemistry 2009, 113, 96–102.
- Jayaprakasha, G.K.; Singh, R.P.; Sakariah, K.K. Antioxidant activity of grape seed (Vitisvinifera) extracts on peroxidation models in vitro. Food Chemistry 2001, 73, 285–290.
- Emad, S.S. Antioxidative effect of extracts from red grapeseed and peel on lipid oxidation in oils of sunflower. LWT-Food Science and Technology 2006, 39, 883–892.
- Chu, Y.H.; Kung, Y.H. A study on oxidative stability of vegetable oil blends. Food Science 1997, 24, 389–397.
- Toliwal, S.D.; Tiwari, M.R.; Verma, S. Studies on thermal stability of palm-corn oil blends. Journal of Oil Technologists Association of India 2005, 37, 18–20.
- Chakra, W.; Claudio, C.; Peter, F.; Amy, R.; Zhiping, S. Canola oil with improved oxidative stability: Potential utilization of phenolic antioxidants naturally occurring in canola. CSIRO Food and Nutritional Sciences, Werribee, Australia. ISF, Sydney, 2009.
- Khaled, M.M.; Rafaat, M.E.; Mohamed, F.R.H. Improving thermal stability of high linoleic corn oil by blending with black cumin and coriander oils. International Journal of Food Properties 2014, 17(3), 500–510.
- Che Man, Y.B.; Marina, A.M.; Abdul, R.; Al-Kahtani, H.A.; Norazura, O. A fourier transform infrared spectroscopy method for analysis of palm oil adulterated with lard in pre-fried french fries. International Journal of Food Properties 2014, 17(2), 352–362.
- Ramli, N.; Nafar, M.; Jaswir, I. Oxidative stability of blend oil during deep-fat frying of potato chips. Pakistan Journal of Nutrition 2012, 11, 828.
- Monika, C.; Kiran, G. Blended rice bran and olive oil—moving towards a new cooking media. International Journal for Life Sciences and Educational Research 2013, 1, 14–20.
- Reza, F.; Reza, E.K.; Hashem, P. Frying stability of canola oil blended with palm olein, olive, and corn oils. Journal of Oil & Fat Industries 2009, 86, 71–76.
- Ashraf, G.A.; Reza, F.; Mohammad, H.H.K. Frying stability of canola oil in presence of pumpkin seed and olive oils. European Journal of Lipid Science and Technology 2010, 112, 871–877.
- Manel, B.A.; Khalil, D.; Mohamed, D.; Noureddine, A. Stabilization of sunflower oil during accelerated storage: Use of basil extract as a potential alternative to synthetic antioxidants. International Journal of Food Properties 2014, 17, 7.
- Sneha, S.; Shriyash, P.; Sumit, A.; Vivek, S. Oxidative stability of ghee incorporated with clove extracts and BHA at elevated temperatures. International Journal of Food Properties 2014, 17, 7.
- IUPAC Official Methods, 6th Ed; Pergamon Press: Oxford, UK, 1979.
- AOCS Official Methods and Recommended Practices, The American Oil Chemists’ Society: Champaign, IL, 1997.
- European Community, Commission Regulation 2568/91, Off. J. Eur. Commun. 1991, L248, 1–82.
- Parker, T.D.; Adams, D.A.; Zhou, K.; Harris, M.; Yu, L.J. Fatty acid composition and oxidative stability of cold-pressed edible seed oils. Journal of Food Science 2003, 68, 1240–1243.
- Mori, T.A.; Burke, V.; Puddey, I.B.; Watts, G.F.; O’Neal, D.N.; Best, J.D.; Beilin, L.J. Purified eicosapentaenoic and docosahexaenoic acids have differential effects on serum lipids and lipoproteins, LDL particle size, glucose, and insulin in mildly hyper lipidemic men. American Journal of Clinical Nutrition 2000, 71, 1085–1094.
- Kris-Etherton, P.M.; Harris, W.S.; Appel, L.J. Fish consumption, fish oil, omega-3 fatty acids, and cardiovascular disease. Circulation 2002, 106, 2747–2757.
- White, J.P. Fatty acids in oilseeds. In: Fatty Acids in Foods and Their Health Implications; Chow, C.K.; Ed.; Marcel Dekker Inc.: New York, NY, 2000.
- Vever-Bizet, C.; Dellinger, M.; Brault, D.; Rougee, M.; Bensasson, R.V. Singlet molecular oxygen quenching by saturated and unsaturated fatty-acids and by cholesterol. Photochemistry and Photobiology 1989, 50, 321–325.
- Hemalatha, G.; Ghafoorunissa, Sesame lignans enhance the thermal stability of edible vegetable oils. Food Chemistry 2007, 105, 1076–1085.
- Aftab, A.K.; Sherazi, S.T.H.; Rubina, S.; Razia, S.; Ambrat, Arfa, Y. Consequence of fatty acids profile including trans fat in chocolate and pastry samples. International Food Research Journal 2013, 20, 601–605.
- Aladedunye, F.A.; Przybylski, R. Degradation and nutritional quality changes of oil during frying. Journal of the American Oil Chemists Society 2009, 86, 149–156.
- Sanibal, E.A.A.; Mancini filho, J. Fatty acids trans fat and oil hydrogenated soybean in the frying process. Ciencia Tecnol. Alimentos 2004, 24, 27–31.
- Moya Moreno, M.C.M.; Mendoza Olivares, D.; Amezquita Lopez, F.J.; Gimeno Adelantado, J.V. Analytical evaluation of polyunsaturated fatty acids degradation during thermal oxidation of edible oils by Fourier transform infrared spectroscopy. Talanta 1999, 50, 269–275.
- Guillen, M.D.; Cabo, N. Characterization of edible oils and lard by Fourier transform infrared spectroscopy: Relationships between composition and frequency of concrete bands in the fingerprint region. Journal of the American Oil Chemists Society 1997, 74, 1281–1286.
- Silverstein, R.M.; Blaser, G.C.; Morril, T.C. Spectrometric Identification of Organic Compounds, 3rd Ed.; John Wiley & Sons: New York, NY, 1974.
- Silverstein, R.M.; Bassler, G.C.; Morrill, T.C. Infrared Spectrometry, 5th Ed.; Wiley: New York, NY, 1991.
- Safar, M.D.; Bertrand, P.; Robert, M.; Devaux, F.; Genot, C. Characterization of edible oils, butters, and margarines by Fourier transform infrared spectroscopy with attenuated total reflectance. Journal of the American Oil Chemists Society 1994, 71, 371–377.
- Nasirullah. Development of deep frying edible vegetable oils. Journal of Food Lipids 2001, 8, 295–304.
- Daya, G.; Padmini, S.; Arvind, R. Effect of deep-fat frying of potato chips and chicken on the quality of soybean oil. Journal of Consumer Studies and Home Economics 2000, 24, 223–223.
- Abdulkarim, S.M.; Long, K.; Lai, O.M.; Muhammad, S.K.S.; Ghazali, H.M. Frying quality and stability of high-oleic Moringaoleifera seed oil in comparison with other vegetable oils. Food Chemistry 2007, 105, 1382–1385.
- Talpur, M.Y.; Sherazi, S.T.H.; Mahesar, S.A.; Aftab, A.K. Effects of chicken frying on soybean, sunflower, and canola oils. Pakistan Journal of Analytical and Environmental Chemistry 2009, 10, 59–66.
- Haryati, T.; Che Man, Y.B.; Ghazali, H.M.; Asbi, B.A.; Buana, L. Determination of iodine value of palm oil based on triglyceride composition. Journal of the American Oil Chemists Society 1998, 75, 789–792.
- Tynek, M.; Hazuka, Z.; Pawlowicz, R.; Dudek, M. Changes in the frying medium during deep-frying of food rich in proteins and carbohydrates. Journal of food Lipids 2001, 8, 251–261.