Abstract
Dielectric properties of apple, apricot, peach, and pear nectars were studied in the frequency range from 15 kHz to 30 MHz and the temperature range from 25 to 60°C. Both the relative dielectric constants and the dielectric loss factors decreased by increasing frequency and increased linearly with increasing temperature with values in the order 104–102 and 105–102, respectively. The power dissipation densities and the power penetration depths were found to increase linearly with temperature. Power dissipation densities remained essentially constant for all the samples while power penetration depths decreased significantly on increasing frequency. The dependence of each of the two dielectric properties on frequency and temperature has been described by two simple equations that proved to be adequate to describe the trend of the relative dielectric constant and dielectric loss factor for all the frequencies temperatures considered. It has been established that the dominant mechanism for the dielectric loss is ionic conductivity.
INTRODUCTION
Fruits and vegetables juices are among the top selling beverages worldwide and represent a convenient way to improve consumption of fruits and vegetables with beneficial effects on consumers’ health, as recommended by international organizations.[Citation1] Food processing that involves inactivation of pathogenic microorganisms is used to prevent food poisoning during the consumption of raw products. In fact, fruit juices have been the source of several outbreaks, mostly involving Salmonella and E. coli O157.[Citation2]
Today, the vast majority of fruit juices is pasteurized by heating the juice to a high temperature, typically between 92 and 105°C for a time sufficient to remove all microbiological hazards. Although thermal treatment has proven to be very effective at inactivating pathogenic organisms, it also alters other properties of juices such color, texture or taste, as well as their nutritional value.[Citation3] Therefore, there is a great interest in developing alternative inactivation methods that are able to guarantee the same effectiveness in reducing health hazards while avoiding the previously mentioned disadvantages.[Citation4] Among these, high hydrostatic pressure (HHP) and electro-heating are options.
HHP treatments result in a better final product from the organoleptic point of view and have been increasingly used worldwide. Nevertheless, HHP does not seem to be sufficiently effective in the removal of some pathogens and a residual surviving population is sometimes observed after HHP inactivation.[Citation2] Electroheating seems to be a very promising way to address the problem of inactivation, due to the very high heating rate and uniformity.[Citation5–Citation7] In electroheating methods, an electrical current can be applied directly to the food as in ohmic heating (OH) or can be previously converted to electromagnetic radiation that, in turn, interacts with the sample. Depending on the frequency range used, it is called radio frequency (RF) or microwave (MW) heating. In particular, RF heating has attracted a considerable interest in recent years due to the fact that, in contrast with OH, it can be applied directly to packed food and that RF radiation penetrates much deeper in the sample than MW, thereby generating less hot or cold spots or surface overheating.[Citation5]
RF conventionally occupies the portion of the electromagnetic spectrum between 3 kHz and 300 MHz[Citation8] with most industrial applications using frequencies that lie in the range of 10–50 MHz (6.78, 13.56, 27.12, and 40.68 MHz are reserved for industrial, scientific, and medical applications).[Citation5,Citation9] When RF radiation interacts with a sample the oscillation of the polarity of the electric field makes the ions oscillate forward and backward—ionic polarization—dissipating part of the kinetic energy by friction as heat. In principle, also continuous reorientation of dipolar molecules—dipole rotation—may lead to the production of heat by friction. But this latter phenomenon usually has a significant effect in the case of MW heating (300 MHz–300 GHz).[Citation5,Citation10,Citation11]
In addition to thermal methods that rely on the previously mentioned mechanism, also non-thermal sterilization methods using RF radiation have been recently proposed by Geveke and Brunkhorst.[Citation12–Citation15] In this method high electric fields are applied to liquids for a very short time (<1 ms) at moderately low temperature (<50°C) and the microorganisms are inactivated by electroporation that consist in the rupture of cell membrane due to the induced voltage formed across the membrane because of its capacitance.[Citation14,Citation16] The frequencies used for this application are quite lower than in thermal methods usually ranging from 15 to 70 kHz.
Dielectric properties are the most important attribute needed to understand and characterize the interaction between food samples and the electric field produced at the operating RF. Hence, knowledge of the dielectric properties of materials is important to the development of RF treatment protocol and subsequent scale up to industrial production.[Citation17,Citation18] The dielectric properties of interest for most applications are the relative dielectric constant, and the dielectric loss factor,
, that constitute the real and imaginary part, respectively, of the complex relative permittivity,
. The relative dielectric constant is associated with the ability of a sample to store energy in the electric field while the dielectric loss factor is related with the dissipation of electrical energy in the material as heat.
Many studies have been conducted on the dielectric properties of solid or semisolid foods such as fruit, vegetables, bread, eggs, meat, and seafood[Citation17] and fluids such as milk, vinegar, fruit juices, and honey.[Citation17,Citation19] However, most of these studies have explored only the higher portion of the frequency spectrum focusing mostly on the MW region or the RF spectrum above 20 MHz. Moreover, many of the published studies were conducted at room temperature only. Therefore, a parallel-plate probe was used to study the dielectric properties of several commercial fruit nectars at frequencies from 15 kHz to 30 MHz (typically used in low frequency RF inactivation processes) within the temperature range of 25–60°C. The results obtained were used to estimate the power dissipation density and power penetration depth for the fruit nectars.
MATERIALS AND METHODS
Materials
The four fruit nectars (from apple, apricot, peach, and pear) were commercially available long-life products (Zuegg S.p.A., Verona, Italy) that were stored in tetra brick® (Tetra Pak, Sweden) aseptic package and were purchased from a local store in Palermo (Italy). The samples were used without any further treatment. Three of them contained citric acid as acidifier and all of them contained L-Ascorbic acid as antioxidant.
Dielectric Properties Measurements
The dielectric properties were measured with the parallel plate method[Citation17] using a precision impedance analyzer Agilent model 4293A equipped with a 16452A liquid test fixture connected with a 16452-61601 cable.[Citation20] The parallel plate method consists of sandwiching a thin sheet of the sample between two electrodes to form a capacitor. The measured capacitance is then used to calculate permittivity.
For each measurement, 800 points were acquired on a logarithmic scale from 15 kHz to 30 MHz. The range includes some of the frequency allocated for industrial, scientific and medical (ISM) applications: 6.78, 13.56, and 27.12 MHz.[Citation9] Before measuring the dielectric properties, the impedance analyzer was allowed to warm up for at least 1 h. The instrument was calibrated at the beginning of every measurement session using the procedure suggested in the operating manual.[Citation20]
The following procedure was used for dielectric measurement: fixture was heated at the maximum experimental temperature—namely 60°C—and capacitance of the void test fixture, C0, was measured and assumed equal to that of air at the same temperature, as indicated in operating manual and other supporting documentation.[Citation20] After air capacitance was measured, 3.4 mL sample was poured into the test fixture and the temperature was monitored with a K-type thermocouple temperature sensor. Permittivity measurement were conducted pre-heating the measurement cell above 60°C in an oven. Once the sample was introduced in the cell, it thermally equilibrated with the cell very quickly due to its small mass with respect to that of the cell. The measurements at different temperatures were performed at 5°C intervals as the sample cooled from 60 to 25°C: When the temperature reached each targeted value, the thermocouple was removed and the measurement was performed. Temperature remained constant during the measurement that was virtually instantaneous. After finishing the measurements for each replicate, the nectar was drained out from the test fixture, which was disassembled, cleaned with water and acetone, and, finally, dried at room temperature. Relative dielectric constant and dielectric loss factor of the nectar samples were calculated using the following equations:
where, , is the relative dielectric constant;
is the dielectric loss factor; CP is the equivalent parallel capacitance of the sample and C0 is that of the air; RP is the equivalent parallel resistance and f is the frequency; α is a correction function, depending on CP, RP, and f calculated following the procedure indicated in the operating manual.[Citation20] All measurements were repeated three times with a seven days interval, following the procedure above described. For each of the 800 frequencies the mean value of the three replicates and the standard deviation were calculated. For clarity reasons, all the figures show only the average values of the three measurements as the trends of the three replicates overlapped.
RESULTS AND DISCUSSION
Effect of Frequency on Dielectric Properties
Dielectric constant, , and dielectric loss factor,
, for the four fruit nectars are shown in and , respectively, as a function of the frequency (15 kHz to 30 MHz) and temperature of 25°C; the same values, together with standard deviations, are listed for selected frequencies and temperatures in –. Overall, the maximum relative error, defined as ratio between the standard deviation and the mean value, was of 15 % for the relative dielectric constant and of 5 % for the dielectric loss factor. It can be clearly seen that for all the different nectars both
and
decrease sharply on increasing the frequency.
TABLE 1a Relative dielectric constant and dielectric loss factor at different temperatures and selected frequencies for the apple nectar (mean ± standard deviation for three replicates)
TABLE 1b Relative dielectric constant and dielectric loss factor at different temperatures and selected frequencies for the apricot nectar (mean ± standard deviation for three replicates)
TABLE 1c Relative dielectric constant and dielectric loss factor at different temperatures and selected frequencies for the peach nectar (mean ± standard deviation for three replicates)
TABLE 1d Relative dielectric constant and dielectric loss factor at different temperatures and selected frequencies for the pear nectar (mean ± standard deviation for three replicates)
In particular, for all the samples, the relative dielectric constant assumes very high values in the range 15–150 kHz, decreases on increasing frequency until it approaches values similar to that of water (≈80). This behavior seems to indicate the presence of α and/or β relaxations[Citation21,Citation22] which are quite common in biological samples with complex membrane structures. The α relaxation can be mainly attributed to the lateral movement of the ions forming a counter-ion layer around colloidal particles present in the sample (macromolecules, subcellular structures, etc.). In the presence of an external electric field, the ions move as a whole like a large dipole determining a low-frequency relaxation. Movements of ions through membrane pores may also represent a contribution to the overall effect. The β relaxation is caused by the accumulations of ions at membrane surfaces under an external electric field due to the blocking of ions movements by the membrane. This phenomenon is also known as Maxwell-Wagner effect. It can be noted that the four samples show a qualitatively similar behavior, even though there are small differences for the rate at which falls with frequency. Perusal of the experimental data suggested to the authors to perform a non-linear regression of results via the following analytical expression:
TABLE 2 Parameter b of dielectric constant model, calculated at 25°C (a = 5.00), with correlation coefficient R and standard error SE for the four nectars
Parameter b corresponds to the frequency at which the relative dielectric constant is about 40 times that of water; smaller b values entail that will fall more rapidly with frequency. However, the nature of the biological structures involved in the dispersion phenomena is quite complex and the physical modeling of b is made difficult by the fact that the similarities between biological structures are more in their functions and composition and less in their exact structure.[Citation21]
The dielectric loss factor can be generally expressed as:[Citation17]
presents log at 25°C for the four nectars. It can be clearly noted that the respective experimental data form straight lines parallel to each other. These findings strongly indicate that the ionic conductivity loss mechanism is dominant in the frequency range considered and it is consistent with previous results at higher frequency range:[Citation19] Indeed, assuming negligible the contribution of the other mechanisms
in Eq. (4) it follows that:
that represents, in logarithmic terms, a sheaf of parallel straight lines.
Fitting Eq. (6) to the experimental data permitted to estimate the conductivity σ for each sample, reported in together with the statistical parameter R and SE. Since proved to be constant with frequency in the range evaluated, it follow from Eq. (5) that the ionic contribution decreases with increasing frequency.
TABLE 3 Intercept, correlation coefficient R, standard error SE of the linear regression, and conductivity σ of the four nectars, calculated at 25°C
Effect of Temperature on Dielectric Properties
Temperature could have a great effect on the dielectric properties of many substances.[Citation24,Citation25] In this study for the four fruit nectars considered an increase in temperature led to an increase in both the relative dielectric constant and the dielectric loss factor, although effects do not occur in the same manner for the two properties.
Mean values with standard deviations of and
in the temperature range of 25–60°C for selected frequencies are listed in –.
The increase of dielectric constant on increase temperature is more noticeable in the lower part of the frequency spectrum investigated (f < 1 MHz approximately); at higher frequencies the temperature influence becomes less important till the trend of , tend to flatten where it becomes negligible. Conversely, the temperature effect on the dielectric loss factor is significant over the whole frequency range with increases up to about 15% going from one temperature to the next.
Equation (3) proved to be adequate to describe the trend of the relative dielectric constant for all the temperature considered, keeping unaltered the parameter b and substituting the parameter a with a linear function of temperature, which is the same for all nectars, i.e.:
As for the dielectric loss factor, the bi-logarithmic model continues to be an adequate description of experimental data for each nectar and at each temperature considered. Linear regression of Eq. (6) results in correlation factors above 0.999 and SEs smaller than 2.17 10–2.
shows how conductivity linearly increases with temperature for all the samples, while reports the linear fitting parameters together with the correlation coefficients and the standard errors.
FIGURE 4 Graph of conductivity, as a function of temperature for the four fruit nectars (circle apple, square apricot, triangle peach, diamond pear).
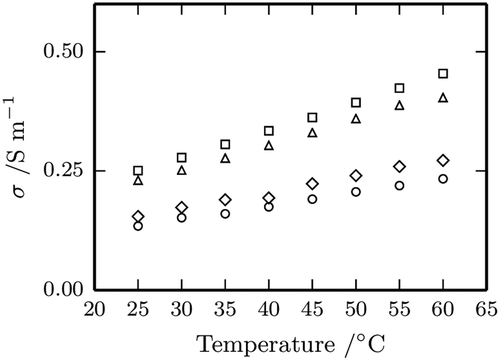
TABLE 4 Linear fitting parameters with correlation coefficients R and standard errors SE for conductivity σ with temperature of the four nectars
Effect of Frequency and Temperature on Power Dissipation Density and Penetration Depth
During RF heating, the electromagnetic radiation is converted in heat by the interaction of the electromagnetic field with the charges present in the sample. The overall effect depends on the as well as on the intensity and frequency of the electric field applied and other physical properties of the sample.[Citation16,Citation22,Citation25] The amount of energy dissipated per unit of time and volume is the power dissipation density that depends on
of the sample as illustrated in the following equation:
FIGURE 5 Penetration depth, dp as a function of frequency for apple nectar in the temperature range from 25 to 60°C.
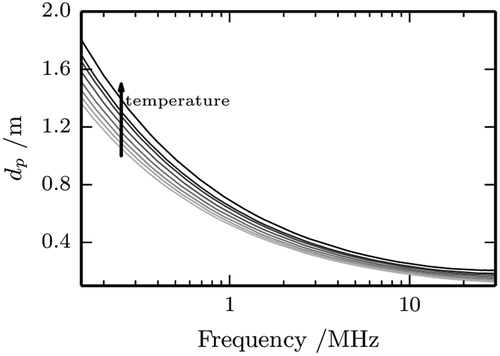
where, T is the absolute temperature in K; t is time in s; ρ is the density of the material in kg m–3 and Cp is its specific heat in JK–1kg–1. The increase of the dielectric loss factor and PV with temperature may lead to the so called thermal runaway effect in which a heated region will continue to increase its temperature, leaving colder regions elsewhere. The resulting non-uniform heating could hinder the inactivation process and should be avoided by stirring the sample during the treatment.
Equation (8) shows how the dielectric loss factor has a direct effect on the energy conversion. Nevertheless, also the effect of the relative dielectric constant should be taken into consideration. Actually, determines the intensity of the electric field in the sample which also has a crucial role in determining the amount of energy converted into heat.[Citation26] In order to take into account this effect, it is convenient to use a parameter known as the penetration depth, dp. It is defined as the depth into a sample where the radiation power has dropped to 1/e (e = 2.718) corresponding to 36.8% of the value at the surface of the sample. It is an important parameter to evaluate the ability of a radiation to provide uniform heating.[Citation17,Citation18] The penetration depth (in meters) for RF and MW radiations is a function of the relative dielectric constant,
, and the dielectric loss factor,
. It can be calculated by the following equation:[Citation17]
where, c is the speed of light in free space in m s–1 and f is the frequency of the radiation in Hz. As it can be easily deduced, the penetration depth decreases on increasing frequency and high values for the relative dielectric constant and loss factor lead to a reduction of its value. shows a representative plot of dp vs. f. It can be noted that the penetration depth is strongly reduced on increasing frequency, going from nearly 2 m to a little less than 10 cm. Increasing temperature affects also dp: at 60°C its value is reduced by roughly one third of its value at 25°C.
CONCLUSIONS
The characterization of the dielectric properties of four different fruit nectars in the frequency range from 15 kHz to 30 MHz and in the temperature range from 25 to 60°C demonstrated how the dielectric behavior of this kind of materials is influenced by frequency and temperature in the low region of the RF spectrum. Both the relative dielectric constant and the dielectric loss factor show a decrease on increasing frequency and an increase on increasing temperature. The dependence of each of the two dielectric properties on frequency and temperature has been described by two simple equations with very good results. It has been established that the dominant mechanism for the dielectric loss is ionic conductivity. In addition, power dissipation densities and penetration depths calculated for all the samples were found to increase linearly with temperature. The data obtained and the proposed models provide useful information about the dielectric properties of fruit juices and could be of great value for the development of RF pasteurization processes at low frequencies. The data obtained show that RF inactivation processes could benefit from the use of radiation in the lower portion of the RF spectrum where penetration depths are quite large so that, in principle, it would be possible to treat large volumes both in continuous or batch processes. At the same time, the trends observed for the power dissipation densities with temperature indicate that it will probably be needed to assure a continuous mixing of the samples in order to avoid a possible thermal runaway effect.
FUNDING
This research is part of the DIT (Dynamic Irreversible Thermoporation) project supported by PO FESR 2007/2013 fund of European Union.
Additional information
Funding
REFERENCES
- WHO & FAO. Diet, nutrition, and the prevention of chronic diseases. WHO technical report series 916, 2003.
- Nguyen-The, C. Biological hazards in processed fruits and vegetables—Risk factors and impact of processing techniques. LWT–Food Science and Technology, 2012, 49, 172–177.
- Qin, B.L.; Pothakamury, U.R.; Vega, H.; Martin, O.; Barbosa-Canovas, G.V.; Swanson, B.G. Food pasteurization using high-intensity pulsed electric fields. Food Technology 1995, 49, 55–60.
- Cammalleri, M.; Pipitone, E.; Rubino, T.; Saporito, L.; Mammina, C. Journal of Food Process Engineering, 2015.
- Marra, F.; Zhang, L.; Lyng, J.G. Radio frequency treatment of foods: Review of recent advances. Journal of Food Engineering, 2009, 91, 497–508.
- Piyasena, P.; Dussault, C.; Koutchma, T.; Ramaswamy, H.S.; Awuah, G.B. Radio frequency heating of foods: Principles, applications, and related properties—A review. Critical Reviews in Food Science and Nutrition, 2003, 43, 587–606.
- Zhao, Y.; Flugstad, B.; Kolbe, E.; Park, J.W.; Wells, J.H. Using capacitive (radio frequency) dielectric heating in food processing and preservation—A review. Journal of Food Process Engineering, 2000, 23, 25–55.
- Hitchcock, R.T. Radio-Frequency and Microwave Radiation; AIHA: Falls Church, 2004, p. 1.
- International Telecommunication Union (ITU). Radio Regulations: Articles; ITU: Geneva, Switzerland, 2012, p. 60.
- Buffler, C.R. Microwave Cooking and Processing: Engineering Fundamentals for the Food Scientist; Springer: Berlin, Germany, 1993, p. 16.
- Rowley, A.T. Radio Frequency Heating. In Thermal Technologies in Food Processing. Woodhead Publishing Limited: Cambridge, UK. Number 55 in Woodhead Publishing Series in Food Science, Technology and Nutrition, 2001, pp. 163–177.
- Geveke, D.J.; Brunkhorst, C.; Fan, X. Radio frequency electric fields processing of orange juice. Innovative Food Science & Emerging Technologies 2007, 8, 549–554.
- Geveke, D.J.; Brunkhorst, C. Radio frequency electric fields inactivation of Escherichia Coli in apple cider. Journal of Food Engineering 2008, 85, 215–221.
- Geveke, D.J.; Brunkhorst, C. Inactivation of in apple juice by radio frequency electric fields. Journal of Food Science, 2004, 69, FEP134–FEP0138.
- Geveke, D.J.; Brunkhorst, C. Inactivation of Saccharomyces Cerevisiae with radio frequency electric fields. Journal of Food Protection, 2003, 66, 1712–1715.
- Zimmermann, U. Electrical Breakdown, Electropermeabilization, and Electrofusion. In Reviews of Physiology, Biochemistry, and Pharmacology, Vol. 105, Springer: Berlin, Germany, 1986; pp. 175–256.
- Sosa-Morales, M.; Valerio-Junco, L.; López-Malo, A.; García, H. Dielectric properties of foods: Reported data in the 21st century and their potential applications. LWT–Food Science and Technology, 2010, 43, 1169–1179.
- Schubert, H.; Regier, M.; Tang, J. Dielectric Properties of Foods. In The Microwave Processing of Foods; Woodhead Publishing Limited. Number 112 in Woodhead Publishing Series in Food Science, Technology, and Nutrition: Cambridge, UK, 2005; pp. 22–40.
- Zhu, X.; Guo, W.; Wu, X. Frequency- and temperature-dependent dielectric properties of fruit juices associated with pasteurization by dielectric heating. Journal of Food Engineering, 2012, 109, 258–266.
- Agilent Technologies. Technical support: 16452A liquid test fixture Agilent, 2003.
- Kuang, W.; Nelson, S. Low-frequency dielectric properties of biological tissues: A review with some new insights. Transactions of the American Society of Agricultural Engineers 1998, 41, 173–184.
- Pliquett, U. Bioimpedance: A review for food processing. Food Engineering Reviews 2010, 2, 74–94.
- Ryynänen, S. The electromagnetic properties of food materials: A review of the basic principles. Journal of Food Engineering 1995, 26, 409–429.
- Shrestha, B.L.; Baik, O.D. Dielectric behaviour of whole-grain wheat with temperature at 27.12 MHZ: A novel use of a liquid dielectric test fixture for grains. International Journal of Food Properties 2015, 18 (1), 100–112.
- Ngadi, M.; Dev, S.R.S.; Raghavan, V.G.S.; Kazemi, S., Dielectric properties of pork muscle. International Journal of Food Properties 2015, 18 (1), 12–20.
- Nelson, S. Dielectric properties of agricultural products-measurements and applications. IEEE Transactions on Electrical Insulation 1991, 26, 845–869.