Abstract
In decortication of wild apricot pit a mathematical model based on Hertz’s theory of contact stress was developed for the prediction of force required to break the pit. Using pits subjected to a uniaxial compression stress, in their lateral axis between rigid parallel plates, and those propelled to impinge a rigid cylindrical seat along its lateral axis, experimental verification of the model was conducted comparing the theoretical predictions with estimates from the representations of conventional methods employed in pit cracking. Properties including size, shape, and mass, required in the derived model were determined for wild apricot pits. Material stiffness was obtained from the force-deformation curve. Material stiffness obtained was 51.1 × 106 to 46.9 × 106 Nm-1 for different sizes of the pits (12, 11, 10, and <10 mm). The respective ranges of geometric mean diameters were between 16.92–15.05 mm. There was no significant variation of pit sphericity with size of pits. Force prediction from the Hertzian model proposed gave good prediction of cracking force that was not significantly different from that obtained from an impact force decorticator (1.2 kg weight reciprocating with 100 rpm and 30 cm stroke length) and compression force required calculated from a texture analyzer.
INTRODUCTION
The apricot pit is recovered as a major by-product in the processing of apricot fruit (Prunus armeniaca L.) for oil. The pit is a composite biomaterial consisting of an outer hard shell and embedded softer kernel, which is extracted for the production of apricot kernel oil. The high amounts of monounsaturated and polyunsaturated fatty acids present in wild apricot oil make it an effectual massage oil for aged and dry or irritated skins.[Citation1] It has ability to penetrate the skin and does not make the skin oily. Kernel oil is also used as treatment of gynaecological disorders which are skin related. The economic importance of the apricot kernel is further indicated by its use for humans and food, traditional medicine, cosmetics, and other industrial applications. However, the quality of the oil depends, among other things, on efficient nut cracking, which will enhance effective separation of the kernel from the shell. Akubuo and Eje[Citation2] reported some efforts on the separation of the kernel from the shell but the efficiency of the experimental machine was limited largely because some shell particles and kernels have comparable sizes.
FIGURE 1 Compressive loading of wild apricot pit between two parallel plates on the texture analyzer machine: (a) longitudinal loading; (b) transverse loading.
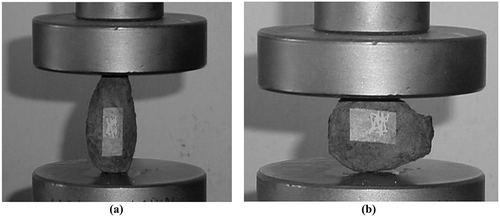
In relation to apricot kernel extraction, two major classes of apricot namely, “sweet” and “bitter,” are common, and are classified as big-sized and small-sized pits, respectively.[Citation3,Citation4] Prior to cracking, the pits are dried sufficiently to enable the kernel to shrink away from the shell, which minimizes kernel breakage. The nuts are then cracked in a machine or manually.
In cracking, material breaks without entire separation of shell and kernels, hence it is loaded without crushing of embedded kernel. Peasant farmers, who abound in the trade, break the nuts one at a time between two stones, judging the magnitude of the applied force by experience. The apricot pit, in a natural rest position, lies longitudinally so that the impact is applied along the lateral axis. Conventional mechanical decorticators are often of the roller type.[Citation4–Citation6] The nuts are fed in batches into the cracking chamber where pits are compressed by a very high speed rotating metal rollers that throws the cracked shell and kernel mixture at the bottom. The speed and clearance between rollers are adjusted for acceptable cracking efficiency.
The knowledge of minimum impact required for pit cracking is, therefore, paramount to design improvement of the existing mechanical decorticator. A quantitative analysis of the mechanical behavior and failure criteria of the pit would, therefore, lead to identification of important material-based parameters relevant in determining the minimum force required in pit cracking. In pursuit of this goal, application of Hertz’s law with some modification was contemplated. A similar analysis, applying a crack closure theory of a linearized shallow shell, is reported in the literature in respect to a Macadamia nutshell.[Citation7–Citation9]
Although agricultural materials are generally non-homogeneous, non-isotropic, and non-elastic, it has been possible to define an elastic range of behavior within which elastic parameters can be quantified.[Citation10] Observed that under small strains, most agricultural materials exhibit extensive elasticity, to which Hertz’s theory of contact stress is applicable. However, owing to the complexity of the stress system and inability to determine the failure area with sufficient accuracy, the force-deformation response of an egg[Citation11] and a cocoa pod[Citation12] was expressed in terms of stiffness modulus rather than elastic modulus. The stiffness modulus is the ratio of the maximum load to the maximum deformation at the straight-line portion of the force-deformation curve.[Citation25] Toughness is defined as the work required to cause the material to rupture. This can be approximated by the area under the stress-strain or force-deformation curve up to the rupture point.[Citation10]
Generally, biomaterials under compressive load may rupture after charting a straight force-deformation curve. This pattern was reported for soybeans,[Citation13] cocoa pods,[Citation14,Citation15] corncobs,[Citation16] and Macadamia nuts.[Citation7] In “brittle” material, rupture may occur in the early portion of the force-deformation curve beyond the linear limit, while it may take place after considerable plastic flow in “tough” material.[Citation10] Also, according to Timoshenko and Goodier[Citation17] in the application of Hertz’s law to practical situations, established equations for static conditions hold during impact if vibration of contacting bodies is neglected. This condition can be assumed for pit cracking with minimal impact force since cracking terminates vibration propagation at once, therefore, resulting in a longer duration of impact than the period of vibration. Furthermore, it was assumed that the errors which might result from applying Hertz’s analysis for small deformations to the problem of pit cracking would be minimized by considering only the elastic region of the force-deformation behavior of the pit, therefore, assuming that the pit cracks immediately after its elastic limit. Consequently, in order to gain a better understanding of the pit cracking behavior during decortication, this study is aimed at developing a Hertzian model to simulate its cracking response to quasi-static and impact loading. Experimental verification of the derivation was also undertaken.
Theoretical development
The Fundamental Hertz Relationships
Hertz relationships were developed for two bodies under a static compressive load based on some fundamental assumptions. These assumptions are that the material is homogeneous, contact stress is over a small area relative to the size of the parts, radii of curvature of the contacting solids by far exceed radius of the contact surface, and that the surfaces are smooth.[Citation10] The requirement for smooth surfaces implies that only a normal compressive stress arises over the contact surface, so that tangential forces are eliminated. The maximum contact stress due to force F in N at the center of the surface of contact, denoted by σmax in Nm–2, is given by:
where, α and β are the major and minor semi-axes in m of the elliptic contact area. The values of α and β in Eq (1) are expressed[Citation10] as follows:
where, the coefficient A is given by:
For the two bodies, Ri and R’i define the minimum and maximum radii of curvature in m, respectively; υ is Poisson’s ratio; E is the modulus of elasticity in Nm–2 of the contacting bodies. Subscripts 1 and 2 refer to bodies 1 and 2. The combined deformation D in m of the two bodies along the axis of load at the point of contact is given by:
The values of the factors k, m, and n depend on the principal curvature of the bodies at the point of contact and the angle between the normal planes containing the principal curvatures. The values are given in tables reported by Mohsenin[Citation10] and Timoshenko and Goodier.[Citation17] For manual cracking of the pits between two stones (which can be approximated by the compression of a spherical object between two parallel plates) or the case of a pit impacting the wall of a pit cracker (approximated by a sphere impinging on a large seat) the following conditions prevail:
for the flat plate, R1 = R’1= ∞;
for the large seat, radius of cylindrical seat R1 is much bigger than the radius of the pit R2,
R1 = R’1 >> R2
for the spherical body (pit) with diameter d in m, R2 = R’2 =
and[Citation10]
k = 1. 3514
Therefore, the combined deformation D in m at the point of contact becomes,
For the particular case of rigid plate or cylindrical seat made of steel or material with much higher elastic modulus of elasticity E1 than the sphere E2,
Basic Hertz Theory Equation for Pit Cracking during Decortication
In order to adapt the Hertz equation to loading of an apricot pit to failure, representing the condition for minimal cracking force, Eq. (6 and 7) were combined and written as,
However, for agricultural material, stiffness modulus S in Nm–1 rather than the elastic modulus is used and,
where, A0 is the actual contact area in m2 over which the force is acting.
Hence,
This is the basic Hertz theory equation for a palm nut under compressive axial load.
Modification of the Basic Hertz’s Equation
The sphericity index γ was introduced as a multiplier of the particle geometric mean diameter of the particle to take care of the deviation of the true shape of the nut from the theoretical spherical shape that was assumed in the analysis.
Hence,
where, da is the apparent diameter of the biomaterial in m. Thus,
If there is hollowness in pits, pods, shells, or husks, it offers to any appreciable resistance to cracking and necessary to incorporate correction factor for thickness in calculated modulus. Here, for the apricot pit, it was not necessary to apply a correction factor on the account of shell thickness since the material stiffness, which is to be determined experimentally, depends on shell thickness.[Citation12]
Experimental work
Sampling Technique
Apricot pits, from the “wild” variety were obtained from a College of Forestry and Hill Agriculture Ranichouri, Distt-Tehari, Uttarakhand, India. This is the only variety utilized for oil extraction. The samples of the pits were drawn from those already dried in the sun for kernel extraction to eliminate variation between test samples and actual field samples. The pit was cleaned to remove the foreign material like plant leaves, dust, mud balls, stones, etc. Cleaned samples were graded properly using wild apricot pit size grader developed by AICRP on PHT, G.B.P.U.A & T, Pantnagar, Uttarakhand (India) Center. Graded pits were stored properly in gunny bags or a metallic container for further experiments of decortication.
Guner et al.[Citation3] found that the cracking strength of the apricot pits significantly varies with size and shape, hence the grading of pits before the experimentation is a crucial factor. Also other properties like physical dimensions, surface contact areas, etc., are directly used for calculation and uniformity in their values is desirable, which obtained by proper grading.
Test Equipment and Methodology
Physical characteristics
The moisture content of the pits was obtained using the oven method (IS 4333-II, 1967).[Citation18] The pits were dried at 130ºC for 2 h as specified for oil-bearing seeds. The pits were passed through a set of sieves of wild apricot pit grader with 20 × 12, 20 × 11, 20 × 10 mm apertures to grade the pits. The three significant axial dimensions of 20 randomly chosen pits from each grade were measured using a vernier caliper to determine the geometric mean diameter.[Citation26] The thickness of the shell was measured using a micrometer screw gauge. Equivalent diameter da (g) of the nut was defined, according to Mohsenin[Citation10] as
where, a is the major diameter in m; b is the intermediate diameter in m; and c is the minor diameter in m.
Various required properties, i.e., a, b, c, S, A, D, d, W, etc., were calculated by procedures followed by.[Citation19,Citation20]
Uniaxial Compression Test
The quasistatic, uniaxial parallel plate compression test was performed on the pits. The load was applied along the lateral axis, using a texture analyzer (Stable Microsystem Model TAHD plus [double column]—XTZi, UK) using 75 mm compression platen stainless steel probe () (Probe code: P/75).[Citation21] During testing, the load was progressively increasing at a constant rate of 5 mm/min until the pit cracked. The slow speed allowed each pit to be compressed for an appreciable time before it cracked. Before the experimentation grading of the pits was done with pedal operated wild apricot pit grader with operating conditions and procedure given by Kate et al.[Citation22] and the oversize retained on each sieve was quantified. The pits retained on the 20 × 11 mm sieve, which was most abundant in the samples were used to eliminate variation with pit size. Each experimental value is an average of 20 replicates, loading one pit at a time until it fails. Samples of pits retained on other sieve sizes were loaded to failure to facilitate a comparison of observed cracking force for different pit grades with estimates from other experiments. The force-deformation curve obtained was subsequently analyzed to obtain the stiffness modulus and the toughness as the area under the curve. However, it was not possible to obtain the imprint of the actual area of contact (A0) under the uniaxial compression because the pits split up at cracking. Therefore, as an approximation the area was assumed to be equal to a cross-section through an equivalent sphere and at a distance of maximum elastic deformation of the pits from its surface. Thus, the equivalent contact area, Ae, was taken as,
where, Ae is equivalent area, m2 da is apparent diameter of irregularly shaped body, m, and D is elastic deformation, m.
Experimental Verification of Theory
Apricot pits were used in the experiments in this section. All the samples were drawn from the same lot for which the physical properties were determined. The apricot pit decorticator used for the experimentation was working on the principle of pit decortication due to combined impact and compression force produced by the reciprocating piston hammer. Hence, two forces were considered, i.e., impact and compression for cracking of wild apricot pits.
Reciprocating drop-weight impact experiment
The pits was decorticated by a properly designed piston of 1.5 kg weight impacted continuously on the apricot pits rest on the bottom platform during its reciprocating motion from constant properly designed height and optimized speed (100 rpm)[Citation5] and without bouncing on contacting the pit.[Citation20] At the initiation of crack, assuming no energy is lost during the impact, the kinetic energy of the falling weight is transformed in to elastic strain energy of the pit.[Citation20] It can be shown that, for a load W in N, dropping a certain height h in m on to a body with material stiffness S in Nm–1, the equivalent impact is:
Statistical Analysis
The analysis of variance (ANOVA) tables were generated for comparison of Hertzian model, axial compression, and falling impact. Tukey’s honestly significant difference (HSD) test was performed: for those responses data for which ANOVA was significant, and to determine differences between the means by using SPSS 16.0.2.
Results and discussion
Physical Properties
The moisture contents (percent wet-basis) of the pits at the time of experiments were 12.5% for selected samples of pits. The size distribution of the pit samples and other measured physical properties are shown in . The range of geometric means is varied between 16.92–15.05 mm. The sphericities of the pits are 0.723 to 0.711 for wild apricot pit. Statistical analysis () showed no significant variations in pit size and sphericities with different grades. This confirms the adequacy of assuming the same sphericity index for pits of different sizes and grades in the Hertzian model. The average mass of pits retained on each sieve size is reported in . As expected, the average mass varied significantly with size (). Therefore, this result in conjunction with Eq (15) indicates that pits of different sizes hurled at the different impacts with different pit size. Thus, the result provides support for differential cracking of apricot pits based on pit sizes. Otherwise, some pits will experience excessive impacts with attendant kernel breakage, while some will be discharged without cracking. Shell thickness is an important factor which affects the pit stiffness. Also, it varies with the different varieties and stage of maturities.[Citation23]
TABLE 1 Some physical properties of apricot pit samples used in the experiments
Visual observation of the shell after pit cracking shows that the shell is thinnest about its middle along the longitudinal axis. This feature causes the pit to be weakest along the transverse axis, which coincidentally is presented for impact in natural rest position, as is the case in manual cracking.[Citation5] The values obtained are shown in , and are within the ranges reported in the literature.[Citation19]
Uniaxial Compression Test
Results of compressing the pits between two parallel plates of texture analyzer at a constant loading rate are shown in . Although total deformation at pit cracking was quite variable, the overall behavior followed a predictable trend. Two regions are noticeable in the experimental plots, illustrated in with a wild apricot pit.
FIGURE 2 Force-deformation curves for two sizes of apricot pits along transverse axes at 5 mm/min loading rate of texture analyzer showing three stages of biomaterial deformation: I: transient; II: apparent linear elastic; III: plastic to failure point.
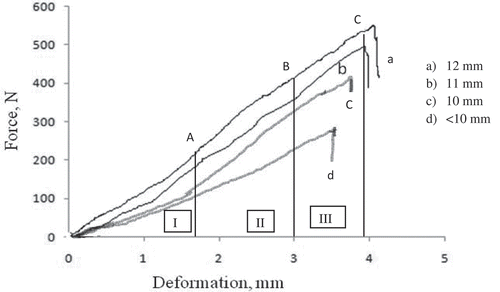
(i) Apparent elastic stage
During the apparent elastic stage, which is the primary resistance to deformation stage of the pit under compression, the behavior I is apparently linear elastic. This stage projects into the failure stage without any dissipative or plastic stage, normally presented in the ideal force-deformation curve.[Citation10] In the case of wild apricot pits, it was found in the rage of 0 to 200 N.
(ii) Failure stage
During the failure stage, the pit cracks and splits the shell into fragments. The gradient is gentler than the elastic stage so that resistance at cracking (point C) is only slightly higher than the value at elastic limit (point B). This observation, therefore, in a way corroborates the assumption that the pit cracks at near the upper limit of the elastic range. The gradient of the linear range, which indicates material stiffness S, gave values of 51.1×106 to 46.9×106 Nm–1 for different sizes of the pits (12, 11, 10, and <10 mm). These values are to be used in the derived Hertzian model.
Verification of the Hertzian Model
The results based on the derived Hertzian model and the estimates from experiments are summarized in . The predicted cracking force, based on Hertz’s theory, was compared with the values obtained from the three sets of experiments, using paired t-tests.[Citation24] Values obtained from the Hertzian model, though lower, were not significantly different from those obtained from either the impact type of decorticator or the axial compression test. The values obtained from the Hertzian model experiment were generally higher and hence, gives the maximum value of force for design purposes.
TABLE 2 Comparison of predicted and measured force required in apricot pit decortication
Therefore this observation gives credence to the suitability of using the derived model for prediction of force required in pit cracking, with the predicted value just greater than what is obtained for the falling impact and the axial compression. In the quasistatic compression test, whose result was not significantly different from the predictions from the Hertzian model, the shell split and scattered at pit failure. In comparison, the pit merely cracked in the manual or the falling impact and the shell was further removed from the kernel with additional impact. Therefore, the magnitudes of impact in the compression test (agreeing with mechanical pit cracker) should be higher than for the falling impact (approximation of manual cracking) as was the case in this report. Hence, observations in this respect are in agreement with practice.
Conclusion
Using a theoretical expression derived for the Hertzian linear elastic contact model developed for the apricot pit cracking process, the minimum force requirement for pit cracking can be determined from important material properties. These properties found to influence pit cracking were pit size, variety and shell thickness. The magnitude of the cracking force was found to also depend on pit stiffness, which could be determined from the slope of the apparent elastic region of the force-deformation curve. The curve upon integration also yields the modulus of toughness of the pit. Prediction of the fracture load obtained from the model for apricot pits is in good agreement with experimental results.
NOMENCLATURE
REFERENCES
- Anon. Properties of Apricot Seed Oil. Retrieved from www.shea-butter.com. Newsletter, March 12, 2003.
- Akubuo, C.O.; Eje, B.E. Palm Kernel and Shell Separator. Biosystems Engineering 2002, 81 (2), 193–199.
- Guner, M.; Vatandas, M.; Dursun, E. Determination of Cracking Characteristics of Some Variety of Apricot Stones. Journal of Agricultural Sciences 1999, 5 (1), 95–103.
- Sharma, P.C.; Tilakratne, B.M.K.S.; Gupta, A. Utilization of Wild Apricot Kernel Press Cake for Extraction of Protein Isolate. Journal of Food Science Technology 2010, 47 (6), 682–685.
- Kate, A.E.; Lohani, U.C.; Shahi, N.C.; Pandey, J.P. Optimization of the Wild Apricot Pit Decorticator using Response Surface Methodology. International Agricultural Engineering Journal 2013, 22 (1), 27–33.
- Ogunsina, B.S.; Bamgboye, A.I. Fracture Resistance of Cashew Nuts as Influenced by Pre-Shelling Treatment. International Journal of Food Properties 2013, 16 (7), 1452–1459.
- Wang, C.H.; Mai, Y.W. Deformation and Fracture of Macadamia Nuts—Part 2: Microstructure and Fracture Mechanics Analysis of Nutshell. International Journal of Fracture 1994, 69, 67–85.
- Wang, C.H.; Zhang, L.C.; Mai, Y.W. Deformation and Fracture of Macadamia Nuts—Part 1: Deformation Analysis of Nut-in-Shell. International Journal of Fracture 1994, 69, 51–65.
- Zhang, L.; Li, X.; Ren, H.; Liu, L.; Ma, L.; Li, M.; Bi, W. Impact of Using Exopolysaccharides (EPS)-Producing Strain on Qualities of Half-Fat Cheddar Cheese. International Journal of Food Properties 2015, 18 (7), 1546–1559.
- Mohsenin, N.N. Physical Properties of Plant and Animal Materials; Gordon and Breach Science Publishers: London, 1986.
- Reece, F.N.; Lot, B.D. The Effect of Loading Rate on the Breaking Force, Deformation, and Stiffness Modulus of Eggs. Poultry Science 1976, 55, 349–358.
- Faborode, M.O.; Dirinfo, R.R. A Mathematical Model of Cocoa Pod Deformation Based on Hertz Theory. International Agrophysics 1994, 8, 403–409.
- Paulsen, M.R. Fracture Resistance of Soybeans to Compressive Loading. Transactions of the ASAE 1978, 21 (8), 1210–1216.
- Faborode, M.O.; Oladosu, G.A. Development of a Cocoa Pod Processing Machine. The Nigerian Engineer 1991, 25 (4), 26–31.
- Maduako, J.N.; Faborode, M.O. (1994). Characterization of the Breaking Behavior of Whole Cocoa Pods. Journal of Agricultural Engineering Research 1994, 59, 89–96.
- Anazodo, U.G.N. Mechanical Properties of the Corncob under Quasi-Static Radial Compression. Transactions of the ASAE 1983, 26 (4), 1222–1228.
- Timoshenko, S.P.; Goodier, J.N. Theory of Elasticity; McGraw-Hill Book Company: Singapore, 1970.
- AOAC. Official Methods of Analysis, 16th Ed; Association of Official Analytical Chemists: Washington, DC, 1984; 1–15 pp.
- Haciseferogullar, H.; Gezer, I.; Ozcan, M.M.; Asma, B.M. Post Harvest Chemical and Physical-Mechanical Properties of Some Apricot Varieties Cultivated in Turkey. Journal of Food Engineering 2007, 79, 364–373.
- Adebola, K.O. Palm Nut Cracking under Repeated Impact Load. Journal of Applied Sciences 2006, 6 (11), 2471–2475.
- ASAE Standards. Compression Test of Food Material of Convex Shape. American Society of Agricultural Engineering 1998, S–368.3, 554–559.
- Kate, A.E.; Lohani, U.C.; Shahi, N.C.; Sarkar, A.; Pandey, J.P. On-Farm Grading of Wild Apricot Pits with Pedal Operated Grader and Process Optimization using Response Surface Methodology. International Agricultural Engineering Journal 2013, 22 (3), 39–47.
- Opeke, L.K. Tropical Tree Crops; Spectrum Books Limited: Lagos, Nigeria, 1997.
- Box, G.E.P.; Hunter, W.G.; Hunter, J.S. Statistics for Experimenters; John Wiley and Sons: New York, NY, 1978.
- Koya, O.A.; Ogunsina, B.S.; Opeyemi, O.O. Deformation and Dehulling of Sponge Gourd (Luffa aegyptiaca) Seeds. International Journal of Food Properties 2011, 14 (2), 432–440.
- Maghsoudi, H.; Khoshtaghaza, M.H.; Minaei, S. Selected Geometric Characteristics, Density, and Mechanical Properties of Un-Split Pistachio Nut. International Journal of Food Properties 2010, 13 (2), 394–403.