Abstract
The aim of this study was to identify suitable starch sources that can improve the structural integrity of plant protein-based extruded feeds, specifically aquatic feeds. Extrusion trials were performed, using a single-screw extruder. Extruder temperature, screw speed, and moisture content were varied in a factorial design with a replicated central composite point. The effects of these variables on functional and structural properties of the extrudates were evaluated. Temperature had the dominant effect on all properties. Increasing extruder temperature resulted in notable increases in onset temperature of gelatinization and glass transition temperature of all extrudates. The lowest water absorption, alkaline viscosity, and the highest water solubility, in addition to the lowest Rapid Visco Analyzer profile were found for corn starch extrudates. The lowest thermal stability was observed for the potato extrudate. It is expected that cassava and potato starches can contribute better in the formation of a cohesive structure and the generation of more expanded extrudates, specifically in the production of aquafeed. The higher enthalpy transition of corn extrudates indicated the higher thermal stability of corn starch which can lead in production of more durable aquafeed extrudates.
INTRODUCTION
Food and feed products are different in specific properties, which greatly depend on the type of the food ingredients and their behaviors during processing. Starch is the primary carbohydrate source used in various types of the food and feed blend formulations. Its utilization in a food matrix has a decisive role in the formation of the product texture in most of the cases.[Citation1,Citation2] Chemically, starch consists of two major molecules: linear amylose and branched amylopectin. The effectiveness of the starch on the product quality is highly affected by the ratio of amylose to amylopectin.[Citation3] Also, due to its heterogeneous physics, it has two regions of amorphous and crystalline.[Citation4] It is well-known that the physiochemical properties of the starchy products are strongly related to the extent of microstructural alteration of the starch component during the process.[Citation5] This is mainly due to its contribution in gelatinization phenomenon which affects the intermolecular bindings of both amylose and amylopectin macromolecules. Theoretically, gelatinization is an irreversible process cleaving the intermolecular bonds of starch molecules at a high temperature and moistened condition. This can greatly impact the rheological characteristics such as apparent viscosity, and thus the functionality of the materials being processed. In fact, increased viscosity (η) due to gelatinization is the main purpose of the utilization of starch in various applications.[Citation3] The swelling ability of starch, solubility, amount of amylose leaching out during gelatinization, and thus the ability to produce a viscous paste, all indicate the importance of the starch properties.[Citation6]
The extrusion cooking process has specific features that make it different from other heating and cooking processes. Simultaneous effects of high shear forces developing in a short time process, moisture, and high temperature induce several physiochemical changes, altering the structure of the extruding blend ingredients at the molecular level, and thus intimately influencing the characteristics of the extrudates.[Citation7] Chemical alterations occur due to structural changes of the macromolecules in the feed blend, such as starch gelatinization and protein denaturation, as well as incorporation of water into the molecular matrix, all of which convert the raw feed particles into a viscoelastic dough under a pressurized environment. Physical changes, on the other hand, are related to product expansion due to a drastic pressure drop and water evaporation during die exit.[Citation7,Citation8] The structural transformations of starch in the course of extrusion processing have been classified into gelatinization, melting, and fragmentation.[Citation9] These molecular structure alterations can impart certain functional and nutritional properties to the starchy extrudates. Several factors during extrusion processing affect the extent of starch transformations at all three previously mentioned classes. Apart from the effect of extrusion processing parameters (such as temperature, moisture content [MC], and screw speed [SS]),[Citation10,Citation11] other factors also influence the extent of the physiochemical reactions, particularly starch gelatinization, during the process and consequently impact the resulting extrudates properties. These can include starch type, the extruder itself, such as screw and barrel configurations, die dimensions,[Citation11] and residence time of the ingredient in the barrel.[Citation12] Since the utilization of water is limited in extrusion processing, it is possible that the starch component of the extrudate contains a wide spectrum of starch molecules associated with the different stages of the gelatinization process, such as un-gelatinized starch granules, damaged granules, un-swelled gelatinized granules, swelled gelatinized granules, and even completely disrupted starch granules.[Citation13] The loss of crystallinity and molecular size reduction during extrusion processing are practically governed by the mechanical disruption of the intermolecular bindings of the starch due to the high shear stress and strain,[Citation14] which by themselves depend on the amount of energy input under the processing condition. The applied energy converts the crystalline portion of the starch granule to a highly viscose plastic melt, in which the strength of the melt strongly depends on the level of energy input.[Citation15] Thus, the starch granules may disintegrate either entirely or partially. The extrudates obtained from a very low-moisture extrusion processing can have a mixture of all these granules implying the simultaneous occurrence of gelatinization, melting, and fragmentation. Mild extrusion processing of starch may result in extrudates containing limited amount of deformed starch granules.[Citation13,Citation16] Should the starch be subjected to extensively dry heat and high shearing forces, it would undergo dextrinization and break down into dextrin which can partially alter the color of the extrudates into a yellow to brown color. They suggested that starch fragmentation kinetic is mostly governed by dextrinization due to amylopectin debranching.[Citation16] In addition, starch sources with small granules (such as rice and corn) are difficult to gelatinize due to their more compacted molecular structure, while tuber starch sources (such as potato and tapioca) participate in gelatinization more easily and generally exhibit more functional characteristics.[Citation13]
Many of the starch-based or relatively high starch-content food and feed products are produced using extrusion technology at the commercial scale. Aquaculture is a relatively new industry that utilizes extrusion technology.[Citation17] Water stability, durability, buoyancy, and floatability of extrudates are directly related to the extent of extrudate expansion, which is influenced by the degree of starch gelatinization, transformation of other ingredients (like protein denaturation), and consequently their contribution in texturizing of the product in the die section.[Citation18] They postulated that gelatinization during extrusion processing can excel the mechanical strength, due to increasing the cohesion, and can improve digestibility of the extrudates nutrients. This is caused by the absorption of a large quantity of water, that enhances the enzymatic conversion of starch in to a soluble carbohydrate, which is also essential for monogastric animal feeds.
To improve the economic viability of aquaculture production, a considerable amount of research has been conducted to investigate the inclusion of plant-based proteins as fish meal replacements.[Citation19–Citation22] However, substitution of these proteins may result in inferior quality products in terms of the physical and/or biological viability of the feed. Physically, plant-protein based aquafeeds may be less cohesive and less floatable because of their low starch and high fiber contents.[Citation23,Citation24] Attempts have been made to modify plant protein-based feed formulas by adding either a suitable binding agent,[Citation24] or controlling the extrusion processing parameters to achieve a quality feed.[Citation10,Citation24–Citation29] The minimum required starch to produce a floating aquafeed is 18–22%, while that of the sinking feed is 10%.[Citation30] However, this amount is also influenced by the level of inclusion and the functionality of the other ingredients in the feed formula (such as protein). Starch components for aquatic diets come from a variety of starch sources in which wheat is the most readily available and economic source.[Citation13] However, there are also some other sources (such as corn, potato, and cassava), each of which has different characteristics and may contribute differently in the creation of a cohesive structure, floating or sinking extrudates.
Knowledge about the relationships among the extrusion variables and the behavior of the starch will allow us to use starch more effectively in the production of non-fish based aquadiets. This can also help control the complexity of the cooking which occurs during extrusion and produce tailor-made extrudates, while interweaving the extruding non-fish ingredients. Hence, specific objectives of this study were to: (1) characterize the behavior of three different starch sources (corn, cassava, and potato) under various single-screw extrusion processing conditions and (2) investigate the interrelationships among starch property changes during extrusion processing. It is expected that this information will enable us to understand the starches’ structural changes, and ultimately improve plant protein-based extrudates properties to suit various aquadiet products. Some of these properties can vary for different fish species.
MATERIALS AND METHODS
Materials and Sample Preparation
Potato starch (amylose/amylopectin: 0.26) and Cassava (amylose/amylopectin: 0.2) were provided by Penford Food Ingredients, Centennial, CO, whereas corn starch (amylose/amylopectin: 0.38) was provided by Cargill Dry Ingredients, Paris, IL, USA. All chemicals used in this study were purchased from Sigma-Aldrich (St. Louis, MO). The starch samples were conditioned to the desired MCs. To avoid clumping, the calculated amount of water was sprayed onto the starch while mixing continuously at a medium speed in a mixer (Model 600, Hobart Corporation, Troy, OH); the mist and starch were mixed for <10 min. The moistened starch samples were then stored at 25°C in sealed plastic containers for 24 h in order to attain uniform hydration and minimize the variability as much as possible.
Experimental Design and Extrusion Processing
Extrusion cooking of the selected starch sources was conducted in a single-screw, laboratory-scale extruder (Model PL 2000 Brabender Plasti-Corder, South Hackensack, NJ) with a short barrel of 31.75 cm and L/D ratio of 20:1 consisting of three independently heating zones equipped with compressed-air cooling system. A factorial design with a replicated central composite points (3×2×2×2) for the three starch was conducted (). The feeding zone temperature, die dimension, and screw compression ratio were kept constant at 80°C, L/D ratio of 3.3, and 3:1, respectively. All in all 27 extrusion trials, each trial was replicated only once except the one with the central point which was repeated three times each time with a different starch source. The extrudates were collected at steady state condition when the screw was rotated at a constant speed in response to the input torque (approximately). Considerable operation difficulty in extruding low moisture starch samples was observed, specifically for corn starch which led to clogging and inconsistent flow rate, several times. To preserve the structure of the extrudates exiting the die, the starch extrudates were air dried for 48 h. Since the MC of the starch extrudates were low (~<7%), they were set their structure in <1 h. Half of the dried extrudates were ground using the laboratory grinder, sieved to pass through an 80 mesh screen (177 µm) and stored at –20°C until the time of sample analysis. The rest of the extrudates were used for expansion ratio (ER) measurement. MC of the extrudates powders were also determined according to AACC method 44-19 (2000).
TABLE 1 Independent variables and correspondent levels used in this study†
Measurement of Structural Properties of Extrudates
Alkaline viscosity (ηa) and ER
A suspension of 0.2 g starch powder, both raw and extrudates, with 0.5 mL ethanol, and 4 mL of potassium hydroxide (KOH) (5 M) was stirred overnight to obtain a clear solution. The solution was then diluted to 40 mL with double-distilled water.[Citation31] The η of the solution was measured by Viscoanalyzer (ATS Instrument, Bordentown Township, NJ) using concentric cylinder attachment over an increasing shear rate from 1 to 100 s–1, at room temperature, after 10 min equilibration time. The apparent η was recorded at a shear rate of 10 s–1 or when the Newtonian behavior is lost. All measurements were carried out in triplicate. The radial expansion of the extrudates (ER) was determined following Anderson and Conway.[Citation32] Each value was the mean of 10 measurements.
Measurement of Functional Properties
Degree of starch transformation
Peak and final viscosities of the extrudates were determined on a Rapid Visco Analyzer(RVA-4, New Port Scientific, Pty. Ltd., Warriewood, Australia) according to Ozcan and Jackson,[Citation33] with some modification. For raw and extruded starches, 5 ± 0.001 g of a dry sample was added to 25 ± 0.1 mL of distilled water, which had previously been placed in the RVA canister. To attain a consistent comparison and to bring the extrudate samples into a measurable range, relatively high equal sample sizes were chosen. The RVA canister paddle blade was vigorously jogged up and down five times through the sample and within 20 second the slurry was subjected to a RVA heating and cooling profile (hold at 30°C for 1 min, heat to 95°C at 6.5°C/min, hold at 95°C for 5.5 min, cool to 50°C at 6°C/min, and hold at 50°C for 2 min). The paddle speed was set at 960 rpm for the first 5 s and then 160 rpm for the remainder of the analysis. Peak viscosity (ηp) and final viscosity (ηf) were calculated using Thermocline version 2.2 software. The degree of starch transformation due to extrusion processing was manifested as the difference between ηp and ηf.[Citation34] All RVA experiments were run in duplicate.
Water absorption indices (WAI) and water solubility indices (WSI)
These two physical/functional characteristics were determined following Anderson et al.[Citation35] The details for this method were explained elsewhere.[Citation28] Sample from each treatment combination was analyzed in triplicate.
Thermal transition
The thermal transitions of the three starch sources were quantified using differential scanning calorimeter (Model Q 200, TA Instruments, New Castle, DE). All samples were moisture balanced at ~5% db. An amount of 2.0 ± 0.001 mg sample was weighed in an aluminum pan and 4 μL of distilled water was added using a micro-pipette. The sample pan was hermetically sealed and allowed to equilibrate overnight at room temperature. An empty, sealed aluminum pan was also used as a reference. Differential scanning calorimetry (DSC) was calibrated with indium. The starch samples were subjected to a heat scan with the temperature range of –10 to 200°C, and heating rate of 5°C/min. Nitrogen at a flow rate of 50 mL/min was used as a carrier and cooling gas. Duplicate measurements were used for thermal analysis. Onset temperature (To), glass transition temperature (Tg), and enthalpy change due to gelatinization transition (∆HT) were determined using the Universal TA instrument software (V 4.5A).
Statistical Analysis
All collected data were analyzed with Microsoft Excel v.2010 and SAS v.9 (SAS Institute, Cary, NC). The Proc General Linear Model (GLM) procedure was used to identify the main/individual effects due to each independent variable, treatment/simultaneous combination effects, and interactions using a Type I error rate (α) of 0.05. To evaluate the possible dominant effect of starch source as an independent variable on the extrudates’ properties, the main and simultaneous effects of the independent variables on response variables were analyzed in two ways. First, an overall analysis was performed where the starch was considered as one of the individual independent variables among the other variables (i.e., independent variable: ST, T, SS, and MC). The source of starch (ST) was found to be a statistically significant factor influencing most of the responses (α = 0.05). Therefore, ST was considered a blocking factor accounting for treatment variability among the three starch sources being different in nature. This reduces sources of variability, and thus leads to greater precision. Post-hoc least squares mean (LSD) tests were used to determine where the specific differences occurred. The individual effects of extrusion processing parameters (T, SS, and MC) on starch properties due to overall analysis are presented in . Also, the individual effects of these variables (T, SS, and MC) due to blocking analysis for each type of starch (cassava, corn, and potato) are presented in –, respectively.
TABLE 2 Main effects due to starch source (ST), barrel temperature (T), screw speed (SS), and moisture content (MC) on extrudate functional properties (Overall analysis)†
TABLE 3 Main effects of barrel temperature (T), screw speed (SS), and moisture content (MC) on cassava extrudate functional properties†
TABLE 4 Main effects of barrel temperature (T), screw speed (SS), and moisture content (MC) on corn extrudate functional properties†
TABLE 5 Main effects of barrel temperature (T), screw speed (SS), and moisture content (MC) on potato extrudate functional properties†
RESULTS AND DISCUSSION
Structural and Functional Properties
To indirectly estimate of starch degradation profile during extrusion processing, examination of physical, functional, and thermal properties of the extruded starch were used.
ηp and ηf
The individual as well as the simultaneous effects of the independent variables on extrudates ηp and ηf are presented in –. Among the raw starches, potato had the highest ηp and ηf of 14843.3 (cP) and 10144 (cP), followed by 10415 (cP) and 6203.3 (cP) for cassava, and then 7662.5 (cP) and 1594.5 (cP) for corn starches. Mishra and Rai[Citation36] reported the ηp and ηf values of these starches as the following: 4927 and 2227 (cP) for potato; 1761 and 2451 (cP) for cassava; 2604 and 2550 (cP) for corn. The differences among the pasting characteristics of the starches obviously arose due to the variation in the starch botanical source.[Citation36] They also suggested that potato and cassava starches gelatinized faster and in lower temperatures compared to corn starch. This may be due to the more organized crystalline structure of corn starch. Additionally, the different methods used for starch isolation might affect the pasting properties of the starches.
TABLE 6 Treatment combination effects on starch extrudate functional properties blocked according to starch sources†
Macromolecular structural changes occurring during extrusion processing affect the resistance of the extruding materials against the flow, which in turn influences the η of the blend. It has been reported that η changes during extrusion processing play a key role in controlling the expansion of the extrudates.[Citation5,Citation8] The difference between the ηp and ηf of the starch extrudates in the RVA pasting profile reflects the degree of starch transformation during extrusion processing which can indirectly quantify the extent of starch degradation.[Citation37]
Temperature had the most effective influence on the extrudates ηp and ηf ( and ). Increasing T from 80 to 140°C curvilinearly decreased the ηp values by ~25, ~49, and 40% for cassava, corn, and potato, respectively. However, in terms of the cassava and potato starches, ηp slightly increased with raising T from 80 to 110°C. For corn starch, raising the SS from 80 to 150°C and MC from 15 to 22.5% sharply reduced the ηp by 57.5 and 55%, respectively (). But further increasing these variables to the highest point of SS, to 220 rpm, and 30% did not have any significant effect on the ηp. With regard to potato extrudates, increasing SS and MC from the lowest point of 80 rpm and 15% to the highest points of 220 rpm and 30% reduced the ηp by more than 30 and 18%, respectively ().
TABLE 7 Interaction effects due to source of starch (ST), temperature (T), screw speed (SS), and moisture content (MC) on extrudate properties (p-values; overall analysis)†
TABLE 8 Interaction effects due to temperature (T), screw speed (SS), and moisture content (MC) on extrudates properties (p-values)†
ηp of corn extrudates was affected by changing levels of SS and MC in a larger order of magnitude compare to that of the cassava and potato starches. Also, the interaction effect of T×SS×MC was significant on corn extrudates (). In general the lowest RVA profile was observed for corn starch extrudates with the lowest ηp and ηf of 168 and 83.5 (cP) with a treatment combination effect of 140°C–80 rpm–15%, respectively (). Although speculation, it is reasonable to guess that the low values of ηa, ER, ηp, and ηf of the corn extrudates indicate more degradation of corn extrudate at this condition. Due to the low MC, the resistance against the flow could increase and generate more shearing forces. Thus, the starch molecules could be depolymerized due to the effect of mechanical shearing forces along with degradative effect of extrudate temperature (T) and longer residence time.[Citation37] It has been well documented that lower η values for extruded starch are influenced by molecular degradation which occurs during extrusion.[Citation33]
As presented in , RVA viscosity parameters of extruded and raw starches were significantly different (α = 0.05), as expected. illustrates a typical RVA graph for raw and extruded starches. An initial increase in cold η of the starch extrudates was observed (data is not reported). This can be due to the fact that the disintegrated structure of the extrudates can be easily hydrated at low temperature.[Citation37] This is supported by the higher ηa values of the extruded starches. In general with the severity of the processing condition, ηf of all the extrudates decreased which can be due to the smaller molecular size.[Citation38] Microscopic images of the extrudates produced from the most severe conditions of the experimental design are presented in .
FIGURE 2 Microscopic images of extruded starches, and affiliated processing conditions (MC-SS-T); A: Corn, A-a: 15%—220 rpm—140°C, A-b: 15%—80 rpm—140°C, A-c: 30%—80 rpm—140°C, A-d: 30%—220 rpm—140°C; B: Cassava, B-a: 15%—220 rpm—140°C, B-b: 15%—80 rpm—140°C, B-c: 30%—80 rpm—140°C, B-d: 30%—220 rpm—140°C; C: Potato, C-a: 15%—220 rpm—140°C, C-b: 30%—80rpm—140°C, C-c: 15%—80 rpm—140°C, C-d: 30%—220 rpm—140°C.
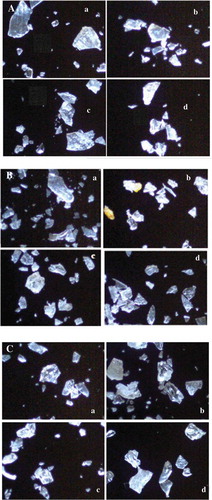
An increase in ηf of the potato extrudate at the treatment combination of 140°C–80 rpm–30% was observed. It can be due to the fact that the potato polysaccharides were becoming disassociated, but did not a have great loss of molecular weight at this condition. In general, as the severity of the extrusion conditions increased, corn extrudates exhibited more loss of η compared to the raw corn starch indicating that the corn starch molecules were breaking down. A similar behavior for twin-screw extruded wheat starch was reported.[Citation38] Earlier research[Citation39] revealed that the pasting properties of starch reflect the rigidity of the starch granules. Therefore, the starch robustness, or ability to survive the extrusion processing in this study, can be ranked as follows: potato ≈ cassava > corn.
ηa
ηa of the extrudates can provide information on the state of starch transformation due to extrusion processing.[Citation31] In other words, it can quantify the degree of macrostructural degradation of the starch.[Citation40] indicates that the effect of starch source as an independent variable on ηa of the starch extrudates is dominant compare to the effects of the other independent variables—T, SS, and MC. Significant differences were observed between the ηa values of the raw and extruded starches (α = 0.05), as expected. Raising T from 80 to 140°C significantly decreased the ηa values of cassava, corn, and potato extrudates by 12.4% (), 9.5% (), and 13.8% (), respectively. However, increasing T from 80 to the 110°C did not show any significant effect on the ηa of cassava and corn extrudates. This indicates that utilized starches behaved in a same manner at extrusion T of 80 and 110°C for this experimental design condition. Variation of SS and MC at all levels did not have any considerable impact on the ηa of these starch extrudates (α = 0.05). Overall, T has greater effect on the ηa of the products. The lowest ηa values of 1.46 (mPa.s) and 2.00 (mPa.s) were observed for cassava and potato extrudates with treatment combination effects of 140°C–80 rpm–15%; while the lowest ηa value of 1.47 (mPa.s) was obtained with treatment combination effects of 140°C–220 rpm–30% for corn extrudates. Because, polysaccharides like starch are solubilized in KOH solution, the extent of ηa reduction for the starch extrudates can indicate the actual molecular size reduction of the starch samples during extrusion processing.[Citation40] In this study, the highest degree of reduction in ηa was observed for cassava followed by corn, and then potato extrudates, 68, 62, and 61%, respectively, compared to those of their raw samples.
ER
The ER was found to depend on all treatment variables, and these relationships were significant (α = 0.05; and ). For cassava extrudates, increasing T from 80 to 110°C decreased the ER values from 1.82 to 1.72; further increasing T to 140°C increased the ER by 49%. The opposite trend was observed for potato starch extrudates. Increasing T from 80 to 110°C, raised the ER from 1.83 to 2.70, and with increasing T to 140°C, ER decreased by 33%. In terms of corn extrudates, increasing T from 80 to 140°C did not have significant influence on ER while raising T to the mid-point of 110°C resulted in an 86% increase in ER. This behavior of the starch extrudates as a function of T was in agreement with what Thymi et al.[Citation41] observed for corn starch extrudates. It was reported[Citation42] that temperatures above 160°C resulted in a significant decrease in ER of extrudates. In another study conducted by Grossmann et al.,[Citation43] the greatest expansion for cassava starch occurred at 170°C.
Increasing SS from the lowest point of 80 rpm to the highest point of 220 rpm led to a curvilinear increase in ER values of cassava and potato extrudates. Where increasing SS from 80 to 150 rpm reduced ER of cassava by 6%, it increased that of the potato extrudate by nearly 60%. Increasing MC from 15 to 22.5% caused a 18% decrease in ER value of cassava extrudates, and further increasing of MC to 30% resulted in a 15% increase in ER values, respectively. A similar trend in changing ER with increasing MC was reported for corn extrudates.[Citation44,Citation45] With respect to corn extrudates, the ER ratio increased and decreased by 62 and 40% with an increased MC from 15 to 22.5% and 22.5 to 30%, respectively. The reduction in ER as a function of MC can be due to the reduction of starch melt elasticity inside the extruder barrel as a function of plasticizing effect of water and thus less molecular degradation of the starch.[Citation44] As the MC decreases, the resistance to flow increases which results in a melt with higher η. Therefore, shearing forces within the extruder barrel increases leading to the degradation of the starch molecules.[Citation45] But, high MC can influence the amylopectin macromolecular structure, reduce the melt elasticity and thus result in production of less expanded product.[Citation46] In this study, increasing ER as a result of increased MC (from 15 to 30%) in ER values of the cassava and potato starches were in contrast to expectations. In fact, the expansion phenomena is governed by the starch gelatinization due to the interactions among water content, pressure, shear stresses and strains during extrusion cooking,[Citation47] and starch molecular transformation during the evaporation process.[Citation48,Citation49] In other words, the degree of expansion is related to the extent of starch macromolecules structural disruption.[Citation47] Treatment combination effect of independent variables is presented in . While the highest ER of 3.68 and 3.07 were observed at treatment combination effects of 140°C–220 rpm–30% for cassava and potato starch extrudates, respectively, that of the corn starch extrudate was 2.71 which occurred at the treatment combination of 110°C–150 rpm–22.5%. On the other hand, the lowest ER values of 1.43 and 1.17 were related to the treatment combination of 80°C–80 rpm–30%, for cassava and corn starches, respectively. In terms of potato starch, treatment combination of 140°C–80 rpm–15% resulted in the lowest ER of 1.33. The ER of the corn starch extrudates was lower than those of cassava and potato. In general tuber starches like potato and cassava contribute more in starch gelatinization and thus provide more expanded products. However, in this study the higher ER of the cassava extrudates can be due to its higher amylopectin content compare to that of the potato starch. Other researchers[Citation50] suggested that there was no simple linear relationship between the ER and macromolecular degradation of the starches during extrusion processing.
(WAI and WSI)
Our results indicated that WAI and WSI of all the starch extrudates decreased and increased with the severity of the extrusion conditions, as expected (–). The highest WSI index of 87.1 % was related for corn extrudates followed by 76.2% for cassava extrudates obtained at the treatment combination of 140°C–220 rpm–30%, followed by 48.8% for potato extrudates at 140°C–80 rpm–15% (). Among the extrudates, the smallest WAI of 0.94 was related to corn starch extrudate which was observed at the highest levels of T and SS either at the low or high MC () Several researchers suggested that the magnitudes of WAI and WSI can be reasonable measures for starch degradation as they are greatly affected by the macromolecular breakdown of starch.[Citation8,Citation45]
Increasing T from 80 to 140°C drastically reduced WAI by 34 and 37.5% for cassava and corn extrudates, respectively. A similar trend was exhibited by potato starch extrudates as a function of changing the level of T from 80 to 140°C and resulted in a 26% reduction in WAI. The decreased WAI in high extrusion T could be due to an increase in starch degradation.[Citation51] These researchers observed that the WAI decreased at T of higher than 150°C. Increasing the level of SS from 80 to 220 rpm increased WAI of cassava extrudates by 21%, while this increase did not influence WAI values of corn extrudates, significantly (α = 0.05). But it resulted in a decrease of 21% in WAI values of potato extrudates (–).
As to the effect of MC, varying levels of MC did not affect the WAI values of corn and potato starch extrudates, significantly (α = 0.05). But raising MC to 30% led to a considerable decrease of 36.5% for cassava extrudates. The decrease in WAI of the cassava extrudates as a result of increased MC was in contrast to what was reported by several researchers.[Citation32,Citation50,Citation52] They also reported that WAI decreased with increased T, which is in good agreement with our results in this study. However, no significant correlation between WAI and the degree of starch macromolecular degradation was found in their extrusion study.[Citation50]
WSI increased as the T increased for all three types of starch extrudates (–). The increasing behavior of WSI as a function of T is supported by decreasing ηa as a result of the T increase. The elevating trend of WSI was similar to the results reported by Anderson[Citation32] and Tang and Ding[Citation50] For corn extrudates, increasing the level of SS from 80 to 150 rpm led to a greater increase of 19.5%; while further increasing of SS to 220 rpm did not have significant impact on WSI. Increasing MC, did not exhibit significant change in WSI of cassava extrudates. However, increasing MC from 15 to 22.55% increased WSI by nearly 14% for corn extrudates. These results were also in good agreement with what the above mentioned researchers observed.
In contrast, potato extrudates exhibited different behavior for WSI in response to the variation levels of SS and MC. Raising SS from 80 to 220 rpm, did not affect the WSI (α = 0.05). A similar trend was observed with variation levels of MC. Taken together, WSI was affected by interaction effect T×SS×MC for all types of the strach extrudates, and the interaction effects of T×SS and T×MC were more siginificant for corn extrudates ().
Thermal properties: Enthalpy of transition (∆HT), TO
As shown, increased T resulted in a significant decrease in ∆HT values of the starch extrudates, as expected. The highest and the lowest ∆HT for the cassava extrudates obtained when all independent variables were at their lowest and the highest values, respectively. The low value of ∆HT for the extrudates obtained from the more severe extrusion condition can be related to the more structural disruption of the strach. The high shear forces along with high T at this condition, could destroy the macromolecular structure of the starch and reduce the crystallinity of the molecule. Thus, resulted in extrudates with less ordered structure and consequently less thermal stability.[Citation53] For corn and potato extrudates, the lowest values of ∆HT were 3.21 (J/g) and 2.65 (J/g) which were observed at 140°C–80 rpm–15% (). The low MC along with the high T and longer residense time could lead to production of extrudate with a higher amount of less ordered residue structure. In regards to the main effect of independent variables, it can be concluded that for cassava and potato strach extrudates, the degree of ∆HT reduction was higher when T increased from 80 to 140°C. While the corn extrudates had the highest ∆HT reduction with increasing T from 80 to 110°C, further incresaing of T to 140°C slightly increased the ∆HT compared to that of centerpoint. Interestingly, at 80°C, ∆HT values of the corn and potato extruadtes were higher than those of their raw starch ( and ). No clear explanation for this observation can be concluded.
The required energy of transition for corn extrudates decreased by 52% with increasing SS from 80 to 150 rpm, while further increasing of SS from 150 to 220 rpm increased the ∆HT by 89%. Varying levels of SS did not change ∆HT value for cassava extrudates, significantly at α = 0.05 (). Increasing MC did not have significant effect on ∆HT values of the cassava and potato extrudates. Whereas ∆HT decreased for corn extrudates with increasing MC from 15 to 22.5% and 15 to 30% by 65 and 24%, respectively. In general, the larger ∆HT changes reflects a higher percentage of crystalinity in starch structure which indicates larger amount of energy are required to gelatinize the crystallites.[Citation54]
In addition, raising extruder T increased TO values for cassava and potato extrudates, progressively. For corn extrudate, increasing the TO of corn extrudates exhibited a curvilinear trend as a function of T. Raising T from 80 to 110°C led to a significant increase in TO from 15.2 to 27.8°C and futher increasing T to 140°C reduced TO to 22.4°C. As the screw rotation increased to 220 rpm, the TO values of all three starch extrudates also increased, though it was not statistically significant. Increasing MC did not have a significant effect on the TO value of the starch extrudates, except for corn extrudates ( to 5), where a significant increase in the onset of transition temperature was observed from 20.1 to 27.8°C, with increasing MC from 15 to 22.5%. The lowest ∆HT of 2.69 (J/g) observed at treatment effect of 140°C–220 rpm–30% was supported by relatively the lowest ηa value of 1.57 (mPa.s) and the highest ER of 3.68 for cassava extrudates, indicating that more strach gelatinization or transformation resulted in lower ηa, higher ER, and lower ∆HT. A similar trend was observed for potato extrudates ()
In terms of Tg, as extruder T increased from 80 to 140°C, Tg increased by 66% for cassava extrudates. Likewise, this temperature rise resulted in a 48 and 83% increase in Tg values of corn and potato extrudates, respectively ( and ). Variation levels of SS did not influence Tg of the starch extrudates (α = 0.05). Changing level of MC only affected Tg of corn extrudates. Increasing MC from 15.5 to 30% led to a 26% decrease in Tg of corn extrudates (), mainly due to the plasticizing effect of water.[Citation55] As shown in , Tg increased with the severity of the extrusion conditions, and the more degraded the starch, the higher the Tg. These observations were in contrast to our expectations. It can be concluded that the endotherm peaks which where observed for the extrudates might be related to the melting transition of the extrudates. It is possible that the starch in the extrudates was fully gelatinized, and thus the observed ∆HT values could be related to the enthalpy of melting. The DSC graph generated for heat flow as a function of T is shown in and for raw and extruded starches, respectively. The different transition trends for raw starch sources are depicted in . These trends clearly indicate a lower TO and Tg for cassava starches compared to corn and potato starches. As shown, increasing Tg and peak T (TP) for the starch extrudates are evident. A similar trend in increasing ∆HT and TP of the transition of starch extrudates were reported by Zhan et al.[Citation56] Some other researchers could not detect the thermal properties of the starch extrudates, implying that the starch was fully gelatinized during their extrusion processing.[Citation33,Citation57,Citation58]
CONCLUSIONS
Extrusion cooking processes can modify starch granular structure at all levels, affecting functional characteristics of the starch and thus physical, chemical, and nutritional properties of the starch-based extrudates. Understanding the extent of starch degradation in such a complex system lightens the path for developing starchy food/feed products. A good agreement with increasing water solubility index, decreasing ηa, increasing ER, and decreasing thermal stability was observed. The lowest RVA profile of corn extrudates indicated that corn starch experienced a higher degree of structural change during the extrusion processing in this study. A remarkable decrease in enthalpy of starch transition with increasing the severity of the extrusion processing was observed. The increased Tg with increasing the severity of extrusion condition needs to be further investigated. Overall, it is expected that cassava and potato starches can contribute better in the formation of a cohesive structure and the generation of more expanded extrudates, specifically in the production of aquafeed. The higher enthalpy transition of corn extrudates indicates the higher thermal stability of corn starch which can lead in production of more durable aquafeed extrudates.
ACKNOWLEDGMENT
The assistance and advice of Dr. Karunanithy Chinnandurai during the extrusion processing is greatly appreciated.
FUNDING
The authors would like to thank the Agricultural Experiment Station, South Dakota State University for the funding, facilities, equipment and supplies.
Additional information
Funding
References
- Whistler, R.L.; Paschall, E.F. Starch: Chemistry and Technology, Vol. 1 and 2, Academic: New York, NY, 1965.
- Giannini, A.N.; Krokida, M.K.; Bisharat, G.I. Structural Properties of Corn-Based Extrudates Enriched with Plant Fibers. International Journal of Food Properties 2013, 16(3), 667–683.
- Ritika, B.Y.; Khatkar, B.S.; Yadav, B.S. Physicochemical, Morphological, Thermal, and Pasting Properties of Starches Isolated from Rice Cultivars Grown in India. International Journal of Food Properties 2010, 13, 1339–1335.
- French, D. Organization of Starch Granules. In Starch: Chemistry and Technology; Whistler, R.; Bemiller, J.N.; Paschall, E.F.; Eds.; Academic Press: Orlando, FL, 1984; 183–256 pp.
- Bowen, S.E.; Gray, D.A.; Giraud, C.; Majzoobi, M.; Millan Testa, C.E.; Bello Pérez, L.A.; Hill, S.E. Lipid Oxidation and Amylopectin Molecular Weight Changes Occurring During Storage of Extruded Starch Samples. Journal of Cereal Science 2006, 43, 275–283.
- Arisaka, M.; Yoshii, Y. Properties of High Amylose Starch Paste. Journal of Applied Glycoscience 1999, 41(1), 1–7.
- Davidson, V.J.; Paton, D.; Diosady, L.L.; Larocque, G.J. Degradation of Wheat Starch in a Single Screw Extruder: Characteristics of Extruded Starch Polymers. Journal of Food Science 1984, 49, 453–458. DOI:10.1111/j.1365 2621.1984.tb12441.x
- Mercier, C.; Linko, P.; Harper, J.M. Extrusion Cooking, AACC International, St. Paul, MN, 1989.
- Lai, L.S.; Kokini, J.L. Physicochemical Changes and Rheological Properties of Starch during Extrusion (A Review.) Biotechnology Progress 1991, 7, 251–206.
- Chevanan, N.; Muthukumarappan, K.; Rosentrater, K.A.; Julson, J.L. Effect of Die Dimensions on Extrusion Processing Parameters and Properties of DDGS-Based Aquaculture Feeds. Cereal Chemistry 2007a, 84, 389–398.
- Aguilera, J.M.; Stanley, D.W. Simultaneous Heat and Mass Transfer: Dehydration. In Microstructural Principles of Food Processing and Engineering, 2nd Ed., Aspen Publishers, Inc.: Gaithersburg, MA, 1999; 373–411 pp.
- Nwabueze, T.U.; Iwe, M.O. Residence Time Distribution (RTD) in a Single Screw Extrusion of African Breadfruit Mixtures. Food and Bioprocess Technology 2008, 3, 135–145. DOI:10.1007/s11947-008-0056-z
- Riaz, M.N.; Aldrich, G. Extruders and Expanders in Pet Food, Aquatic, and Livestock Feeds; Riaz, M.N.; Ed.; Agrimedia: Clenze, Germany, 2007.
- Wen, L.F.; Rodis, P.; Wasserman, B.P. Starch Fragmentation and Protein Insolubilization During Twin-Screw Extrusion of Corn Meal. Cereal Chemistry 1990, 67(3), 268–275.
- Brümmer, T.; Meuser, F.; van Lengerich, B.; Niemann, C. Effect of Extrusion Cooking on Molecular Parameters of Corn Starch. Starch/Stärke 2002, 54, 1–8.
- Gomez, M.H.; Aguilera, J.M. A Physicochemical Model of Corn Starch. Journal of Food Science 1984, 49, 40–43.
- Chang, Y.K.; Wang, S.S. Advances in Extrusion Technology: Aquaculture/Animal Feeds and Foods; Technomic Publishing Co.: Lancaster, PA, 1998.
- Thomas, M.; Paul, T.H.J.H.; Ton, V.V.; Dick, J.V.Z.; Antonius, F.B.V.P. Effect of Process Conditions During Expander Processing and Pelleting on Starch Modification and Pellet Quality of Tapioca. Journal of the Science of Food and Agriculture 1999, 79, 1481–1494.
- El-Saidy, D.M.S.; Gaber, M.M.A. Complete Replacement of Fish Meal by Soybean Meal with Dietary L-Lysine Supplementation for Nile Tilapia Oreochromis Niloticus (L.) Fingerlings. Journal of the World Aquaculture Society 2002, 33, 1119–1127.
- Ali, A.; Al-Asgah, N.A.; Al-Ogaily, S.M.; Ali, S. Effect of Feeding Different Levels of Alfalfa Meal on the Growth Performance and Body Composition of Nile Tilapia (Oreochromis Niloticus) Fingerlings. Asian Fisheries Science 2003, 16, 59–67.
- Khan, M.A.; Jafri, A.K.; Chadha, N.K.; Usmani, N. Growth and Body Composition of Rohu (Labeo Rohita) Fed Diets Containing Oilseed Meals: Partial or Total Replacement of Fish Meal with Soybean Meal. Aquaculture Nutrition 2003, 9, 391–396.
- Amaya, E.A.; Davis, D.A.; Rouse, D.B. Plant Proteins Effectively Replace Fishmeal in Shrimp Feed Trial. Global Aquaculture Advocate 2006, 50–51. http://pdf.gaalliance.org/pdf/GAA-Amaya-Nov06.pdf (accessed June 20, 2012).
- Chin, H.K.; Joseph, A.M.; Jeffery, T.M. Properties of Extruded Dried Distillers Grains (DDG) and Flour Blends. Journal of Food Processing and Preservation 1989, 13, 219–231.
- Chevanan, N.; Muthukumarappan, K.; Rosentrater, K.A. Extrusion Studies of Aquaculture Feed Using Distillers Dried Grains with Solubles and Whey. Food Bioprocess Technology 2009, 2, 177–185.
- Chevanan, N.; Rosentrater, K.A.; Muthukumarappan, K. Effect of DDGS, Moisture Content, and Screw Speed on Physical Properties of Extrudates in Single-Screw Extrusion. Cereal Chemistry 2008, 85, 132–139.
- Chevanan, N.; Rosentrater, K.A.; Muthukumarappan, K. Effects of Processing Conditions on Single Screw Extrusion of Feed Ingredients Containing DDGS. Food Bioprocess Technology 2010, 3, 111–120.
- Fallahi, P.; Muthukumarappan, K.; Rosentrater, K.A. Twin-Screw Extrusion Processing of Vegetable-Based Protein Feeds for Yellow Perch (Perca flavescens) Containing Distillers Dried Grains, Soy Protein Concentrate, and Fermented High Protein Soybean Meal. Journal of Food Research 2012, 1, 3. DOI:org/10.5539/jfr.v1n3p230
- Fallahi, P.; Rosentrater, K.A.; Muthukumarappan, K.; Tulbek, M.C. Effects of Steam, Moisture, and Screw Speed on Physical Properties of DDGS-Based Extrudates. Cereal Chemistry 2013, 90(3), 186–197.
- Chevanan, N.; Rosentrater, K.A.; Muthukumarappan, K. Twins-Screw Extrusion Processing of Feed Blends Containing Distillers Dried Grains with Solubles (DDGS). Cereal Chemistry 2007b, 84, 428–436.
- Riaz, M.N. Using Extrusion to Make Floating and Sinking Fish Feed: Controlling the Water Stability of Feed. Feed Management 1997, 48(1), 21–24.
- Sriburi, P.; Hill, S.E.; Mitchell, J.R. Effects of L-Ascorbic Acid on the Conversion of Cassava Starch. Food Hydrocolloids 1999, 13, 177–183.
- Anderson, R.A.; Conway, H.F.; Peplinski, A.J. Gelatinization of Corn Grits by Roll-Cooking, Extrusion-Cooking, and Steaming. Staerke/Strach 1970, 22, 130–134.
- Ozcan, S.; Jackson, D.S. Functionality Behavior of Raw and Extruded Corn Starch Mixtures. Cereal Chemistry 2005, 82(2), 223–227.
- Lawton, B.T.; Henderson, G.A.; DerLatska, E.J. The Effects of Extruder Variables on the Gelatinization of Corn Starch. The Canadian Journal of Chemical Engineering 1972, 50(4), 168.
- Anderson, R.A.; Conway, H.F.; Pfeifer, V.F.; Griffin, L.E. Gelatinization of Corn Grits by Roll and Extrusion Cooking. Cereal Science Today 1969, 14, 4–7.
- Mishra, S.; Rai, T. Morphology and Functional Properties of Corn, Potato, and Tapioca Starches. Food Hydrocolloids 2006, 20, 557–566.
- Nelles, E.M.; Dewar, J.; van der Merwe, C.F.; Taylor, J.R.N. Granule Integrity and Starch Solubility During Slow, Extended Pasting of Maize Starch—The Second Viscosity Peak. Starch/Stärke 2003, 55, 72–79.
- Majzoobi, M.; Farahnaky, A. Comparison of the Effects of Extrusion Cooking on Some Cereal Starches. International Journal of Food Engineering 2010, 6(3), Article 2. DOI:10.2202/1556-3758.1456
- Sandhya Rani, M.R.; Bhattacharya, K.R. Rheology of Rice-Flour Paste: Effect of Variety, Concentration, and Temperature and Time of Cooking. Journal of Texture Studies 1989, 20, 127–137.
- Paterson, L.A.; Mitchell, J.R.; Hill, S.E.; Blanshard, J.M.V. Evidence for Sulfite Induced Oxidative Reductive Depolymerisation of Starch Polysaccharide. Carbohydrate Research 1996, 292, 143–151.
- Thymi, S.; Krokida, M.K.; Pappa, A.; Maroulis, Z.B. Structural Properties of Extruded Corn Starch. Journal of Food Engineering 2005, 68, 519–526.
- Miranda, M.Z.; Grossmann, M.V.E.; Nabeshima, E.H. Utilization of Brewer’s Spent Grain for the Production of Snacks with Fiber. 1. Physicochemical Characteristics. Arquivos de Biologiae Tecnologia 1994, 37, 483–493.
- Grossmann, M.V.E.; EL-Dash, A.A.; Tavares, D.Q. Effect of extrusion variables on the physical properties of manioc starch. Archives of Biology and Technology 1989, 32, 793–802.
- Chinnaswamy, R.; Hanna, M.A. Macromolecular and Functional Properties of Native and Extrusion-Cooked Corn Starch. Cereal Chemistry 1990, 67(5), 490–499.
- Vergnes, B.; Villemaire, J.P.; Colonna, P.; Tayeb, J. Interrelationships Between Thermomechanical Treatment and Macromolecular Degradation of Maize Starch in a Novel Rheometer with Preshearing. Journal of Cereal Science 1987, 5, 189–202.
- Launay, B.; Lisch, L.M. Twin Screw Extrusion Cooking of Starches: Flow Behavior of Starch Pastes, Expansion, and Mechanical Properties of Extrudates. Journal of Food Engineering 1983, 2, 259–280.
- Stanley, D.W. Chemical and Structural Determinants of Texture of Fabricated Foods. Food Technology 1986, 40, 65–76.
- Friesen, K.G.; Nelssen, J.L.; Behnke, K.C.; Goodband, R.D. Ingredient Processing: Improving Pig Feed by Extrusion. Feed Management 1992, 43(5), 33–38.
- Miller, R.C. Low Moisture Extrusion: Effects of Cooking Moisture on Product Characteristics. Journal of Food Science 1985, 50(1), 249–253.
- Tang, J.; Ding, X.-L. Relationship Between Functional Properties and Macromolecular Modifications of Extruded Corn Starch. Cereal Chemistry 1994, 71(4), 364–369.
- Badrie, N.; Mellowes, W.A. Effect of Extrusion Variables on Cassava Extrudates. Journal of Food Science 1991, 56, 1334–1337.
- Hoseney, R.C.; Mason, W.R.; Lai, C.S.; Guetzlaff, J. Factor Affecting the Viscosity and Structure of Extrusion-Cooked Wheat Starch. In Extrusion Science and Technology; Kokini, J.L.; Ho, C.T.; Karwe, M.V.; Eds.; Marcel Decker Inc.: New York, NY, 1992; 277–305 pp.
- Miyoshi, E. Effects of Heat-Moisture Treatment and Lipids on Gelatinization and Retrogradation of Maize and Potato Starches. Cereal Chemistry 2002, 79, 72–77.
- Jane, J.; Chen, Y.Y.; Lee, L.F.; McPherson, A.E.; Wong, K.S.; Radosavljevic, M.; Kasemsuwan, T. Effects of Amylopectin Branch Chain Length and Amylose Content on the Gelatinization and Pasting Properties of Starch. Cereal Chemistry 1999, 76(5), 629. Retrieved from http://dx.doi.org/10.1094/CCHEM.1999.76.5.629
- Bisharat, G.I.; Eleni, P.N.; Panagiotou, N.M.; Krokida, M.K.; Maroulis, Z.B. Thermal, Textural, and Physicochemical Analysis of Corn Extrudates Enriched with Broccoli or Olive Paste. International Journal of Food Properties 2014, 17(9), 2100–2116.
- Zhana, X.; Wanga, D.; Beanb, S.R.; Moc, X.; Sunc, X.S.; Boyled, D. Ethanol Production from Supercritical-Fluid-Extrusion Cooked Sorghum. Industrial Crops and Products 2006, 23, 304–310.
- Becker, A.; Hill, S.E.; Mitchell, J.R. Milling—A Further Parameter Affecting the Rapid Visco Analyser (RVA) Profile. Cereal Chemistry 2001, 78(2), 166–172. Retrieved from http://dx.doi.org/10.1094/CCHEM.2001.78.2.166
- Cheyne, A.; Barnes, J.; Gedney, S.; Wilson, D.I. Extrusion Behaviour of Cohesive Potato Starch Pastes: II. Microstructure-Process Interactions. Journal of Food Engineering 2005, 66(1), 13–24.