ABSTRACT
In this study, the qualitative and quantitative analysis of the absolute (100%) and aqueous (80%) methanolic extract of Carica papaya Linn processed seed flour samples in terms of their antioxidant properties and their phenolic compounds was investigated. The antioxidant properties were determined by 2,2-diphenyl-1-picrylhydrazyl (DPPH), ferric reducing antioxidant power, and metal chelating assay methods. The protein isolate exhibited higher radical scavenging activity power as compared to other samples. Ferulic acid, caffeic acid, p-coumaric acid, kaempferol-3-glucoside, p-hydroxybenzoic acid, and quercetin-3-galactoside were phenolic compounds isolated with ferulic acid as high as 0.62 mg/DWg. Carica papaya seed isolates and concentrate demonstrated potent antioxidant activity and could be of nutraceutical importance in the pharmaceutical and food industry.
Introduction
Pawpaw is the fruit of Carica papaya which belongs to the genus Carica. It is an invaluable plant that is prevalent throughout tropical Africa and Nigeria and has been the third largest producer globally.[Citation1] In recent years, the role and beneficial effects of this plant which includes the fruit, leaves, and latex (with less emphasis on the seeds), both for its nutritional and medicinal values had attracted much attention from food scientists, health care givers as well as the public. The seeds of the papaya fruits account for about 16% of the fresh fruit weight and each seed is made up of a sarcotesta and endosperm.[Citation2] Pawpaw seed extracts had been shown to demonstrate several medicinal as well as nutritional properties and several species of Caricaceae have been used as medication against a variety of diseases in recent times.[Citation3] It had been argued by scientists that all parts of a pawpaw plant, including the seeds, roots, rinds, and fruits, have positive effects on general health and in prevention of diseases.[Citation4] It is worthy of mention that fermented pawpaw seed had been used to produce the analogue product of the locally produced “Daddawa” normally produced from locust beans as reported by Dakare et al.[Citation5]
The growing interest in the substitution of synthetic food antioxidants and antimicrobial agents by natural ones has fostered research on plant sources and the screening of raw materials for identifying new natural food additives with a broad spectrum of antioxidant and antimicrobial activities.[Citation6] Antioxidants act as cooperative networks employing a series of different redox reactions.[Citation7] Antioxidants, such as vitamin C (ascorbic acid), vitamin E, flavonoids, and polyphenols, are compounds which either prevent or mitigate oxidative stress by donating electrons to ROS, thereby neutralizing the ability of ROS to act as free radicals.[Citation8] The waste or by-products from food processing such as seed and peel of some fruits are potential food and antioxidant source. For example; pomegranate peel and grape seed had been shown to possess higher antioxidant activity than their pulp.[Citation9] Converting these papaya waste seeds into an economic viable product will not only help in reducing the cost of disposing this waste, but will also open up a new source of viable business and income to the papaya industry. Processing the seeds into high protein flours such as protein concentrate and isolate with good nutritional and antioxidant properties could serve as a preliminary step toward the utilization of pawpaw seeds as food ingredients which could make them of nutraceutical importance in the pharmaceutical and the food industry, hence the objectives of the study.
Materials and methods
Carica papaya Linn fruits were gathered from pawpaw trees located at various sections of Obafemi Awolowo University, Ile-Ife, Nigeria. The fruits were split opened and the seeds extracted, washed, and de-coated by rubbing briskly with a domestic grater over a plain surface. The seeds obtained were cleaned and oven dried in a Gallenkamp oven (OVB 305, United Kingdom) at 60°C for 12 h. The dried seeds were milled using the Kenwood grinder and sieved through a 300 μm sieve (Endecott’s sieve, United Kingdom). The sieved flour was packaged using an air tight container and stored at room temperature until used.
The following chemicals were used for analysis: gallic acid, Folin–Ciocalteu’s phenol reagent, anhydrous sodium carbonate (Na2CO3), aluminum chloride (Al2Cl3.6H2O), catechin, sodium hydroxide (NaOH), sodium nitrite (NaNO2), sulfuric acid (H2SO4), 2,2-diphenyl-1-picrylhydrazyl (DPPH), vanillin, hydrochloric acid (HCl), methanol, acetate buffer; 2,4,6-tri-(2-pyridyl)-1,3,5-triazine, ascorbic acid, FeCl3.6H2O, FeCl2.4H2O, and ferrozine and were purchased from Sigma-Aldrich Chem. Co. (United Kingdom). Solvents used for chromatography were of HPLC grade. Water was bi-distillated, and HPLC grade water was obtained by a Milli-Q plus water purification system (Millipore Corp., Bedford, MA).
Experimental design
The modified method of Gbadamosi et al.[Citation10] was employed for the production of the full-fat flour and the defatted flour (). The full-fat flour was defatted using the cold (4°C) extraction method with acetone as the solvent (flour to solvent ratio 1:5 w/v) stirred over a magnetic stirrer for 4 h (). The slurry was then filtered through a Whatman No. 1 filter paper and re-extracted twice in a similar fashion. The defatted flour was de-solventized by drying in a fume hood and finely milled in a Kenwood grinder set at high speed to obtain homogenous defatted flours. The defatted flour obtained was stored in an air-tight bottle and kept in a freezer until use.
Carica papaya protein concentrate was prepared by a method described by Chavan et al.[Citation11] as modified by Gbadamosi et al.[Citation10] (). A known weight (100 g) of the defatted flour was dispersed in distilled water (1,000 ml) to give the final flour to water ratio of 1:10. The dispersion was gently stirred on a magnetic stirrer for 10 min after which the pH of the resultant slurry was adjusted with 0.1 M HCl to the point at which the protein was least soluble (pH 4). This was determined from the solubility profile of the defatted flour during preliminary investigation. The precipitation was allowed to proceed with gentle stirring for 4 h keeping the pH constant. Soluble carbohydrates (oligosaccharides) and minerals were removed by centrifugation at 3,500 × g for 30 min using a centrifuge (Bosch, TDL-5, United Kingdom). The precipitate (concentrate) was washed twice with distilled water and the pH adjusted with 0.1 M NaOH to 7.0 and then centrifuged at 3,500 × g for 10 min. The resultant precipitate (concentrate) was collected and dried in an oven at 45ºC for 8 h.
Figure 2. Scheme for the production of protein concentrate. Adapted from Cheftel et al. [Citation47] as modified by Gbadamosi et al.[Citation12]
![Figure 2. Scheme for the production of protein concentrate. Adapted from Cheftel et al. [Citation47] as modified by Gbadamosi et al.[Citation12]](/cms/asset/b456a09c-3e4e-41bb-9916-89d9a7d31b8b/ljfp_a_1230874_f0002_b.gif)
The protein isolate was prepared from the defatted flour by a method described by Chavan et al.[Citation11] (). A known weight (100 g) of the defatted flour was dispersed in distilled water (1,000 ml) to give the final flour to liquid ratio of 1:10. The suspended solution was gently stirred on a magnetic stirrer for 10 min. The pH of the resultant slurry was then adjusted with 0.1 M NaOH to the point at which the protein was most soluble (pH 10). The extraction proceeded with gentle stirring for 4 h keeping the pH constant. Non-solubilized materials were removed by centrifugation at 3500 × g for 10 min. The proteins in the extract were precipitated by drop wise addition of 0.1 M HCl with constant stirring until the pH was adjusted to 4.0. The mixture was centrifuged at 3500 × g for 10 min using a centrifuge (Bosch, TDL-5, United Kingdom) in order to recover the protein. After separation of proteins by centrifugation, the precipitate was washed twice with distilled water. The precipitated protein was re-suspended in distilled water and the pH was adjusted to 7.0 with 0.1 M NaOH, centrifuged, and freeze-dried. The freeze-dried protein was stored in air-tight glass containers at room temperature for further use.
Figure 3. Scheme for the production of protein isolate. Adapted from Chavan et al.[Citation4]
![Figure 3. Scheme for the production of protein isolate. Adapted from Chavan et al.[Citation4]](/cms/asset/c5e75159-483b-4d02-a124-5c02feb9ad88/ljfp_a_1230874_f0003_b.gif)
For the antioxidant assay, extracts for this analysis were prepared by dissolving 300 mg of the samples in 120 ml of absolute methanol. The suspension was stirred on a magnetic stirrer (Cenco, Netherland) for 4 h and afterward filtered using a Whatman No. 1 filter paper. The filtrate was collected in an amber reagent bottle for use in the antioxidant assay. The same procedure was also used in the preparation of the aqueous methanol extract in the ratio of 80:20 for MeOH:H2O solvent. The bulk extraction was used to prepare different concentrations of the extract.
LC–DAD–ESI–MS/MS analysis of phenolic compounds
The method of Rotondi et al.[Citation12] was adopted for the extraction of phenolic fractions for LC–ESI–DAD–MS/MS analysis. Four grams of the flour sample were added to 2 mL of n-hexane and 4 mL of a methanol/water (70/30; v/v) solution in a 10 mL centrifuge tube. This was vigorously mixed using a vortex and centrifuged for 15 min at 5500 rpm. The hydro-alcoholic phase was collected, and the hexane phase was re-extracted twice with 2 mL of methanol/water (70/30; v/v) solution each time. Finally, the hydro-alcoholic fractions were combined, washed with 2 mL of n-hexane to remove the residual samples, concentrated, and evaporated in vacuum at 35°C. The dry extracts were re-suspended in 0.5 mL of a methanol/water (50:50, v/v) solution and filtered through a 0.2 μm nylon filter (Whatman Inc., Clifton, NJ) before being analyzed by LC–ESI–DAD–MS/MS.
The procedure described by Kelebek et al.[Citation13] was used for the determination of the phenolic compounds using an Agilent 1100 HPLC system (Agilent Technologies, Palo Alto, California, USA). The HPLC system operated by the Windows NT-based ChemStation software was utilized and the equipment was used along with a diode array detector (DAD). Phenolic compounds were eluted under the following conditions: 0.5 ml min–Citation1 flow rate with temperature set at 25°C; isocratic conditions from 0 to 5 min with 0% B; gradient conditions from 0% to 5% B in 20 min; from 5% to 15% B in 18 min; from 15% to 25% B in 14 min; from 25% to 50% B in 31 min; from 50% to 100% B in 3 min; followed by washing and reconditioning of the column. The ultra-violet–visible spectra (scanning from 200 nm to 600 nm) were recorded for all peaks. Triplicate analyses were performed for each sample. The identification and assignation of each compound were performed by comparing retention times and UV spectra to authentic standards; and confirmed by an Agilent 6430 LC–MS/MS spectrometer equipped with an electrospray ionization source. The electrospray ionization mass spectrometry detection was performed in a negative ion mode with the following optimized parameters: capillary temperature 400°C, N2 12 L/min; nebulizer pressure, 45 psi (Kelebek et al.[Citation13]). Data gaining was performed using the Multiple Reactions Monitoring (MRM) method that solely monitors specific mass transitions during pre-set retention times. Phenolics were identified by comparing their retention time and UV–vis data with those obtained with reference standards as well as co-chromatography with added standards and using their mass spectra (m/z 200–600). Quantitative data for phenolics were obtained by calibration curves constructed with known standards.
Antioxidant assay
2,2-diphenyl-2-picrylhydrazyl hydrate (DPPH) assay
The radical scavenging ability of the extract was determined using the stable radical DPPH as described by Pownall et al.[Citation14] The reaction of DPPH with an antioxidant compound which can donate hydrogen, leading to its reduction. The change in color from deep violet to light yellow was measured spectrophotometrically at 517 nm. To 1 ml of different concentrations (0.5, 1.0, 1.5, 2.0, and 2.5 mg/ml) of the samples or standard (vitamin C) in a test tube was added 1 ml of 0.3 mM DPPH in methanol. The mixture was mixed and incubated in the dark for 30 min after which the absorbance was read at 517 nm against a DPPH control containing only 1 ml methanol in place of the samples. The percentage of inhibition was calculated in the following way:
where Acontrol is the absorbance of the control reaction (containing all reagents except the test compound) and Asample is the absorbance of the test compound. A standard curve was prepared by plotting inhibition percentage against sample concentration with a linear range of 0.25–2.5 mg/ml.
Determination of ferric reducing antioxidant power (FRAP)
The FRAP assay was estimated based on a method described by Benzie and Strain.[Citation15] The principle of this method is based on the reduction of a colorless ferric-tripyridyltriazine complex to its blue ferrous colored form due to the donation of electrons by antioxidant compounds. A 300 mmol/L acetate buffer of pH 3.6, 10 mmol/L 2,4,6-tri-(2-pyridyl)-1,3,5-triazine and 20 mmol/L FeCl3.6H2O were mixed together in a ratio of 10:1:1, respectively, to give the working FRAP reagent. A 50 μl aliquot of each sample at 1 mg/ml and 50 μl of standard solutions of ascorbic acid (50, 100, 150, 200, 250 µg/ml) were separately added to 1 ml of FRAP reagent. The mixture was well mixed and the absorbance was measured at 593 nm against reagent blank (50 µl of distilled water and 1 ml of FRAP reagent) after allowing reaction to complete at exactly 10 min. The reducing power was expressed as equivalent concentration (EC) which is defined as the concentration of antioxidant that gave a ferric reducing ability equivalent to that of the ascorbic acid standard. A standard curve was prepared with a linear range of 0.5–2.5 mg/ml using ascorbic acid as the standard, to calculate the equivalent concentration based on the absorbance obtained for the samples, and it was expressed as ascorbic acid equivalent per gram of the sample (AAE/g of the sample).
Metal chelating ability assay
The metal-chelating assay was carried out according to the method of Singh and Rajini[Citation16] with some modifications. Solutions of 2 mM FeCl2·4H2O and 5 mM ferrozine were separately diluted 20 times. Briefly, an aliquot of 1 ml of the different concentrations (0.5, 1.0, 1.5, 2.0, and 2.5 μg/ml) of each sample was mixed with 1 ml of diluted FeCl2·4H2O. After 5 min incubation, the reaction was initiated by the addition of 1 ml of diluted ferrozine. The mixture was shaken vigorously and after a further 10 min incubation period the absorbance of the solution was measured spectrophotometrically at 562 nm. The percentage inhibition of ferrozine–Fe+Citation2 complex formation was calculated by using the formula:
where Acontrol = absorbance of control sample (the control contains mixture of FeCl2 and ferrozine) and Asample = absorbance of a tested samples.
Determination of the total phenol content (TPC)
The total phenols were determined with a Folin–Ciocalteu reagent according to the procedure described by Singleton and Rossi.[Citation17] Gallic acid was used as the standard for the calibration curve and results were expressed as gallic acid equivalents (GAE µg/g) of extracts.
Results and discussion
lists the compounds identified according to different families, including the information provided by HPLC–DAD–ESI–MS/MS analysis: retention time, λmax in the ultraviolet region, molecular ions, main fragment ions in MS/MS, and tentative identification. A total of six phenolic compounds were identified and quantified in the WF, DF, PC, and PI. These include p-hydroxybenzoic acid, caffeic acid, p-coumaric acid, ferulic acid, quercetin-3-galactoside, and kaempferol-3-glucoside. Ferulic acid is the major phenolic compound in experimenter samples, followed by caffeic acid and p-coumaric acid as they constitute the large proportion of the total phenolic content (). These three compounds were also reported to be in higher concentration in papaya skin as earlier reported by Sancho et al.[Citation18]. Ferulic acid had a concentration of 0.62, 0.41, 0.38, and 0.26 mg/DWg for its PI, PC, DF, and WF. The least concentration for the identification of phenolic compounds for the PI, PC, DF, and WF is kaempferol-3-glucoside (). Phenolic compounds have been reported to have anticarcinogen, antiradical, and antimutagenic properties and likewise protect plants from UV radiation.[Citation19,Citation20] Barone et al.[Citation21] stated that ferulic acid synthesis occurs from phenylalanine via the shikimate. Kawabata et al.[Citation22] reported that ferulic acid had the anticarcinogenic effect on colon cancer in rats, and this was correlated with the ability to scavenge free radicals and stimulate the cytoprotective effect of various enzymes. Caffeic acid found in many fruits, vegetables, and coffee is commonly found in esterified form with quinic acid, also known as chlorogenic acid.[Citation23] Da Cunha et al.[Citation24] observed that caffeic acid and its derivatives exert anti-inflammatory activity both in vitro and in vivo, and this activity was due in part to the removal of nitric oxide (NO) and its ability to modulate the expression of INOS (inducible nitric oxide synthase). p-Coumaric acid is an intermediate in the synthesis of phenylpropanoids and has been shown to have antioxidant properties, lowers cholesterol, and provides a defense mechanism against atherosclerosis (Sancho et al.[Citation18]). Zang et al.[Citation25] reported that p-coumaric acid oral administration (317 mg/day for 30 days) inhibited the oxidation of Low Density Lipoprotein (LDL), reduced serum cholesterol levels and did not affect High Density Lipoprotein levels (HDL), while also contributing considerably to the antioxidant capacity, which is directly related to the removal of ROS. The health benefits of phenol compounds are associated with their role in the prevention of several disorders, related to the damaging effect of oxygen free radicals and ROS as earlier stated by Valko et al.[Citation26] Liu[Citation27] found that ferulic acid is covalently conjugated to polysaccharides present in the cell wall, lignin, glycoproteins, and insoluble carbohydrate biopolymers. Among the antioxidant and anti-inflammatory properties that have ferulic acid, it has been observed that it has positive effects on Alzheimer’s disease.
Table 1. The individual flavonoids and phenolic compounds in the extracts (mg/DWg) of understudy Carica papaya samples.
Table 2. Phenolic content of understudy Carica papaya samples (mg/DWg sample).
Table 3. Antioxidant activities of Carica papaya full-fat, defatted flour, protein concentrate and protein isolate.
The DPPH radical is a stable organic free radical with an adsorption peak at 517 nm. Adsorption disappears when accepting an electron or a free radical species, which results in a noticeable discoloration from purple to yellow Liu et al.[Citation27] As shown in and , the DPPH radical scavenging activities of all the extracts were influenced by the sample concentration. The DPPH scavenging activity of the extracts followed the following order: PI ˃ PC ˃ DF ˃ WF. At all concentration levels irrespective of the medium of extraction, PI exhibited the highest free radical scavenging activity value (66.23% and 97.57% at 2.5 mg/ml) followed by PC (53.80% and 89.07% at 2.5 mg/ml) and DF (53.47% and 88.92% at 2.5 mg/ml), 100% and 80% methanolic extract, respectively, while the lowest DPPH radical-scavenging activity was obtained with the full-fat flour at the same concentration. The DPPH radical scavenging activity of all samples was also observes to increase in a concentration dependent manner. This observation is in agreement with the report of Delfanian et al.[Citation28] who stated that increased in scavenging free radicals activities were as a result of increased phenolic compounds at higher concentrations of the extracts. With increasing concentrations of phenolic compounds, the number of hydroxyl groups available in the reaction medium also increases, thus the possibility of hydrogen donation to free radicals increased also. The differences in the radical scavenging ability found here might be attributed to the difference in composition and/or nature, type and pattern of distribution of amino acids within protein molecules. The DPPH radical scavenging activity of all the extracts showed antioxidant potency based on IC50 values and a lower IC50 value indicates a higher antioxidant activity while a higher IC50 value indicates a lower antioxidant activity. The IC50 values as shown in for the PI–WF ranges from 0.266 to 0.109 mg/mL. The aqueous methanol extract had lower IC50 value compared with absolute methanol extract (not listed in the table). This implies that aqueous methanol was a better solvent for extraction of antioxidant compounds in all samples. This observation agrees with the findings of Kang et al.[Citation29] and Siddiq et al.[Citation30] who reported that polar extracts often exhibited stronger activity than non-polar extracts. In summary, Carica papaya protein isolates extracts from both aqueous and absolute methanol were observed to have higher antioxidant activities at all concentrations which might be attributed to greater proportions of proteins in the sample and exposure of those amino acids to oxidative reactions like histidine which are capable of scavenging free radicals.
Figure 4. DPPH radical scavenging activities of pawpaw whole seed flour, defatted flour, protein concentrate and protein isolate (a) 100% methanolic extract (b) 80% methanolic extracts.WF: Whole flour of Carica papaya seed, DF: Defatted flour of Carica papaya seed, PC: Protein concentrate of Carica papaya seed, PI: Protein isolate of Carica papaya seed.
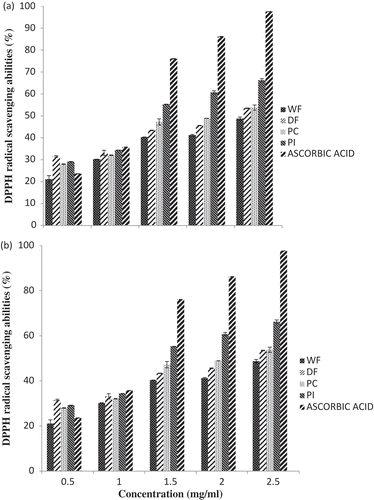
The ferric reducing power is considered a defense mechanism which is related to the ability of the antioxidant agents to transfer electron or hydrogen atom to oxidants or free radicals.[Citation31] This reducing power activity, which may serve as a significant reflection of antioxidant activity, was determined using a modified Fe (III) to Fe (II) reduction assay; the yellow color of the test solution changes to various shades of green and blue depending on the reducing power of the samples. The presence of antioxidants in the samples causes the reduction of the FeCitation2+/ferricyanide complex to the ferrous form. Therefore, FeCitation3+can be monitored by measuring the formation of Perls Prussian blue at 593 nm.[Citation32] The reducing power of ascorbic acid (a well-known antioxidant) was use as the antioxidant equivalent to determine the reducing antioxidant power of the samples. In all tested samples, the reducing power was linearly dependent on the concentration of the samples (). Comparing the 80% methanolic extracts and 100% methanolic extracts, PI exhibited the highest ferric reducing antioxidant power (FRAP) value (1.00 and 0.87 AAE at 2.5 mg/ml) followed by PC (0.90 and 0.68 AAE at 2.5 mg/ml) and DF (0.85 and 0.61 AAE at 2.5 mg/ml) while the lowest FRAP was obtained with the full-fat flour at the same concentration. The difference in their reducing power can be attributed to the difference in their polyphenolic content as reported Wang and Tang[Citation33] on the antioxidant properties of Buckwheat protein isolates. These results suggest that Carica papaya seed products processed into flour with high protein concentration such as its concentrate and isolate content have excellent reducing power, thus exhibiting good potential to be applied as food ingredients with the ability to inhibit FeCitation3+–FeCitation2+ transformation.
Figure 5. Ferric reducing activity (FRAP) of pawpaw whole seed flour, defatted flour, protein concentrate and protein isolate (a) 100% methanolic extract (b) 80% methanolic extracts.WF: Whole flour of Carica papaya seed, DF: Defatted flour of Carica papaya seed, PC: Protein concentrate of Carica papaya seed, PI: Protein isolate of Carica papaya seed.
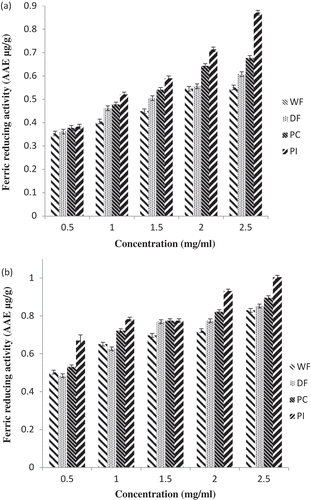
and shows the metal chelating ability of the samples in the range of 0.5–2.5 mg/ml. In this case, ethylene diamine tetra-acetic acid (EDTA), a well-known metal ion chelating agent was applied as the reference. The metal chelating ability of all the samples increased with increasing concentration of the samples. At a concentration of 2.5 mg/ml, extracts of PI exhibited the highest metal ion-chelating ability value for both the 100% and 80% methanolic extracts followed by PC and DF respectively, while the lowest chelating ability was obtained with WF. The high metal ion-chelating ability observed in PI might be due to the molecular and amino acid composition of the proteins in the sample. Some amino acids, such as histidine, tyrosine, methionine, and cysteine, have been reported to show antioxidant activity.[Citation34] In particular, histidine exhibited strong radical scavenging activity due to the decomposition of its imidazole.[Citation34] Disruption of the protein tertiary structure which increased solvent accessibility of amino acid residues capable of scavenging free radicals and chelating pro-oxidative metals might be the reason for this observed increase.[Citation35] The metal ion-chelating (MIC ability) of the extracts revealed antioxidant potency based on the IC50 values in comparison with EDTA. The IC50 value for the protein isolate for the absolute methanol extract was 0.569 mg/ml which was higher than the 0.352 mg/ml value gotten for aqueous methanol extract which implied that the aqueous methanol extracts had better metal chelating ability than the absolute extract. This observation is in agreement with the report of Kang et al.[Citation29] and Siddiq et al.[Citation30] who reported that methanol–water mixture has high polarity and thus greater efficacy toward the extraction of polar phytochemicals such as phenolic and flavonoids. Also, the higher metal chelating ability of the protein isolates may be attributed to greater exposure of effective sites capable of chelating ferrous ions.
Figure 6. Metal chelating abilities of pawpaw whole seed flour, defatted flour, protein concentrate and protein isolate (a) 100% methanolic extract (b) 80% methanolic extracts.WF: Whole flour of Carica papaya seed, DF: Defatted flour of Carica papaya seed, PC: Protein concentrate of Carica papaya seed, PI: Protein isolate of Carica papaya seed.
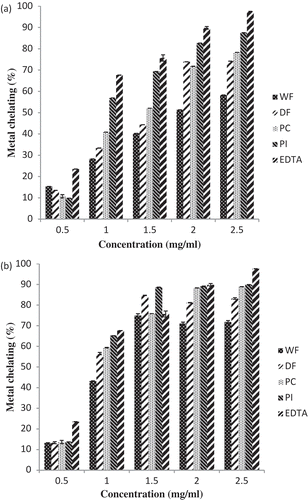
Several findings which involve in vivo and in vitro studies have linked polyphenols of plants to health benefit in humans when consumed. Such benefits includes prevention of certain types of cancer, reduction in the risk of cardiovascular diseases, prevention of obesity and diabetes, and improved functioning of the immune system.[Citation36,Citation37] These health benefits can be said to be as a result of their antioxidant properties which has the ability to neutralize free radicals through their inherent redox activities. Apart from been called radical scavengers, they are also known as metal chelators capable of reducing the rate of Fenton reaction, thereby preventing oxidation caused by highly reactive hydroxyl radicals.[Citation38] To determine the role that the polyphenol present in the protein isolates extracts responsible for the antioxidant activities detected makes to the radical scavenging activities of the sample, a correlation analysis was performed (). The total polyphenol content of extracts obtained under different analytical conditions was found to exhibit a linear relationship to the radical scavenging antioxidant activity (). The presence of such a correlation suggests that high yielding aqueous extraction of polyphenols from Carica papaya protein isolates shows great potential for use in techniques investigating human health benefits. Further studies on the link between the polyphenols of these product and health benefits are therefore highly recommended.
Conclusion
The phenolic screening, TPCs determination and the antioxidants activities studies of Carica papaya protein concentrates and isolates were assessed for the first time. The antioxidant capacity and TPC suggest that the antioxidant activities of the seeds can be mainly ascribed to the phenol compounds. Aside their strong antioxidant activities, these results revealed that the defatted flour, protein isolates, and concentrates make these seeds a promising source of natural antioxidants and other bioactive compounds that could find usefulness in the food and pharmaceutical industries.
References
- Food and Agricultural Organisation (FAO). United Nations Statistics, 2007. http://faostat.fao.org. (accessed on 2nd July 2016)
- Pungasari, S.M.; Abdulkarim; Ghazali, H.M. Properties of Carica papaya L. (Papaya) Seed Oil Following Extraction Using Solvent and Aqueous Enzymatic Methods. Journal of Food Lipids 2005, 12(1), 62–76.
- Mello, V.J.; Gomes, M.T.; Lemos, F.O.; Delfi, J.L.; Andrade, S.P.; Lopes, M.T.; Salas, C.E. The Gastric Ulcer Protective and Healing Role of Cysteine Proteinases from Carica candamarcensis. Phytomedicine 2008, 15, 237–244.
- Seigler, D.S.; Pauli, G.F.; Nahrstedt, A.; Leen, R. Cyanogenic Allosides and Glucosides from Passiflora edulis and (Carica papaya). Phytochemistry 2002, 60(8), 873–882.
- Dakare, M.A.; Ameh, D.A.; Agbaji, A.S. Biochemical Assessment of ‘Daddawa’ Food Seasoning Produced by Fermentation of Pawpaw (Carica papaya) Seeds. Pakistan Journal of Nutrition 2011, 10(3), 220–223.
- Katalinic, V.; Sonja, S.M.; Ivana, G.; Danijela, S.; Ivica, L.; Anja, K. Phenolic Profile, Antioxidant Capacity, and Antimicrobial Activity of Leaf Extracts from Six Vitis vinifera L. Varieties. International Journal of Food Properties 2016, 16(1), 45–60. doi:10.1080/10942912.2010.526274.
- Md. Nazim, U.; Nazi, U.A., Md. Atiar, R.; Rashed, A.; Rehana, A. Antioxidative Potential of the Polyphenolics of Stephania japonica var. Discolor (Blume) Forman: A Chromatographic (High Performance Liquid Chromatography) and Spectrophotometric Measure. International Journal of Food Properties 2016, 19(4), 911–928. doi:10.1080/10942912.2015.1048355.
- Emily M.E.; James K.K. Demonstrating the Minimal Impact of Cultivation Conditions on Antioxidants in Fruits and Vegetables by Differential Pulse Voltammetry. International Journal of Food Properties 2016, 19(4), 826–836. doi:10.1080/10942912.2015.1048354.
- Okonogi, S.; Duangrat, C.; Anuchpreeda, S.; Tachakittirungrod, S.; Chowwanapoonponhn, S. Comparison of Antioxidant Capacities and Cytotoxicities of Certain Fruit Peel. Food Chemistry 2007, 103, 839–846.
- Gbadamosi, S.O.; Abiose, S.H.; Aluko, R.E. Amino Acid Profile, Protein Digestibility, Thermal and Functional Properties of Conophor nut (Tetracarpidium conophorum) Defatted Flour, Protein Concentrate and Isolates. International Journal of Food Science and Technology 2012, 47, 731–739.
- Chavan, U.; Mckenzie, D.; Shahidi, F. Functional Properties of Protein Isolates from Beach Pea (Lathyrus maritimus L.). Food Chemistry 2001, 74, 177–187.
- Rotondi, A.; Bendini, A.; Cerretani, L.; Mari, M.; Lercker, G.; Gallina-Toschi, T. Effect of Olive Ripening Degree on the Oxidative Stability and Organoleptic Properties of Nostrana di Brisighella Extra Virgin Olive Oil. Journal of Agricultural and Food Chemistry 2004, 52, 3649–3654.
- Kelebek, H.; Serkan, S.; Ahmet, S.S.; Songul, K.; Gamze, G.; Osman, K. In LC-DAD/ESI-MS/MS Characterization of Phenolic Compounds of Sunflower Oil. 19th International Sunflower Conference, Edirne, Turkey, International Sunflower Association (ISA), Paris, France, 2016, 1102–1109.
- Pownall, T.L.; Udenigwe, C.C.; Aluko, R.E. Amino Acid Composition and Antioxidant Properties of Pea Seed (Pisum sativum L.) Enzymatic Protein Hydrolysate Fractions. Journal of Agricultural Food Chemistry 2010, 58, 4712–4718.
- Benzie, I.F.F.; Strain, J.J. Ferric Reducing Ability of Plasma (FRAP) as a Measure of Antioxidant Power: The FRAP Assay. Analytical Biochemistry 1999, 239, 70–76.
- Singh, N.; Rajini, P.S. Free Radical Scavenging Activity of an Aqueous Extract of Potato Peel. Food Chemistry 2004, 85, 611.
- Singleton, V.L.; Rossi, J.A. Colorimetry of Total Phenolics with Phosphomolybdic. American Journal of Enology and Viticology 1965, 16(3), 144–158.
- Sancho, G.G.; Laura, E.; Elhadi, M.; Yahia, G.; Adolfo, G.A. Identification and Quantification of Phenols, Carotenoids, and Vitamin C from Papaya (Carica papaya L., cv. Maradol) Fruit Determined by HPLC-DAD-MS/MS-ESI. Food Research International 2011, 44, 1284–1291. doi:10.1016/j.foodres.2010.12.001.
- Cantin, C.M.; Moreno, M.A.; Gogorcena, Y. Evaluation of the Antioxidant Capacity, Phenolic Compounds, and Vitamin c Content of Different Peach and Nectarine [Prunus persica (L.) Batsch] Breeding Progenies. Journal Agricultural of Food Chemistry 2009, 57, 4586−4592.
- Hounsome, N.; Hounsome, B.; Tomos, D.; Edwards-Jones, G. Plant Metabolites and Nutritional Quality of Vegetables. Journal of Food Science 2008, 73, 48−65.
- Barone, E.; Calabrese, V.; Mancuso, C. Ferulic Acid and its Therapeutic Potential as a Hormetin for Age-Related Diseases. Biogerontology 2009, 10, 97−108.
- Kawabata, K.; Yamamoto, T.; Hara, A., Shimizu, M., Yamada, Y., Matsunaga, K. Modifying Effects of Ferulic Acid on Azoxymethane-Induced Colon Carcinogenesis in F344 Rats. Cancer Letters 2000, 157, 15−21.
- Clifford, M.N. Chlorogenic Acids and Other Cinnamates—Nature, Occurrence and Dietary Burden. Journal of the Science of Food and Agriculture 1999, 79, 362−372.
- Da Cunha, F.M.; Duma, D.; Assreuy, J.; Buzzi, F.C.; Niero, R.; Campos, M.M. Caffeic Acid Derivatives: In Vitro and In Vivo Anti-Inflammatory Properties. Free Radical Research 2004, 38, 1241−1253.
- Zang, L.Y.; Cosma, G.; Gardner, H.; Shi, X.; Castranova, V.; Vallyathan, V. Effect of Antioxidant Protection by p-Coumaric Acid on Low-Density Lipoprotein Cholesterol Oxidation. American Journal of Physiology Cell Physiology 2000, 279, 954−960.
- Valko, M.; Leibfritz, D.; Moncol, J.; Cronin, M.T.D.; Mazur, M.; Telser, J. Free Radicals and Antioxidants in Normal Physiological Functions and Human Disease. The International Journal of Biochemistry & Cell Biology 2007, 39, 44−84.
- Liu, R.H. Potential Synergy of Phytochemicals in Cancer Prevention: Mechanism of Action. Journal Nutrition 2004, 134, 3479−3485.
- Mojtaba, D.; Reza, E.K.; Mohammad, A.S. Utilization of Jujube Fruit (Ziziphus mauritiana Lam.) Extracts as Natural Antioxidants in Stability of Frying Oil. International Journal of Food Properties 2016, 19(4), 789–801. doi:10.1080/10942912.2015.1043638.
- Kang, S.C.; Al-Reza, S.M.; Bajpai, V.K. Antioxidant and Antilisterial Effect of Seed Essential Oil and Organic Extracts from Zizyphus jujube. Food and Chemical Toxicology 2009, 47, 2374–2380.
- Siddiq, A.; Anwar, F.; Manzoor, M., Fatima, M. Antioxidant Activity of Different Solvent Extracts of Moringa oleifera Leaves under Accelerated Storage Conditions of Sunflower Oil. Asian Journal of Plant Science 2005, 4, 630–635.
- Ogunmoyole, T.; Rocha, J.B.; Okoronkwo, A.E.; Kade, I.J. Altered pH Homeostasis Modulates the Glutathione Peroxidase Mimics and Other Antioxidant Properties of Diphenyl Diselenide. Chemico-Biological Interactions 2009, 182, 106–111.
- Ferreira, I.C.F.R.; Baptista, P.; Vilas-Boas, M.; Barros, L. Free-Radical Scavenging Capacity and Reducing Power of wild Edible Mushrooms from Northeast Portugal: Individual Cap and Stipe Activity. Food Chemistry 2007, 100, 1511–1516.
- Wang, X.Y.; Tang, C.H. Physicochemical and Antioxidant Properties of Buckwheat Protein Isolates with Different Polyphenolic Content Modified by Limited Hydrolysis with Trypsin. Food Technology and Biotechnology 2012, 50(1), 17–24
- Xie, Z.; Huang, J.; Xu, X.; Jin, Z. Antioxidant Activity of Peptides Isolated from Alfalfa Leaf Protein Hydrolysate. Agriculture 2008, 111, 370–376.
- Elias, R.J.; Kellerby S.S.; Decker E.A. Antioxidant Properties of Proteins and Peptides. Critical Review in Food Science and Nutrition 2008, 48(5), 430–441.
- Visioli, F.; De La Lastra, C.A.; Andres-Lacueva, C.; Aviram, C.M.; M, Calhau C, Cassano A. Polyphenols and Human Health: A Prospectus. Critical Reviews in Food Science and Nutrition 2011, 51, 524–546.
- Vuong, Q.V. Epidemiological Evidence Linking Tea Consumption to Human Health: A Review. Critical Reviews in Food Science and Nutrition 2012. doi:10.1080/2011.594184.
- Tsao, R. Chemistry and Biochemistry of Dietary Polyphenols. Nutrients 2010, 2, 1231–1246.
- Brunner, J.H. Direct Spectrophotometric Determination of Saponin. Analytical Chemistry 1842, 34, 1314–1326.
- Saunders, R.M.; Connor, M.A.; Booth, A.N.; Bickoff, E.N.; Kohier, C.O. Measurements of Digestibility of Alfalfa Protein Concentrate by invitro and invivo Methods. Journal of Nutrition 1973, 103, 530–535.
- Fashakin, J.B.; Ilori, M.O.; Olarenwaju, I. Cost and Quality Optimization of a Complementary Diet from Plant Protein and Corn Flour Using a Computer Aided Linear Programming Model. Nigeria Food Journal 1991, 9, 123–126.
- Gulcin, I.;Oktay, M.; Kireeci, E.; Kufrevioglu O’.I.; Screening of Antioxidant and Antimicrobial Activities of Anise (Pimpinella anisum L.) Seed Extracts’. Journal of Food Chemistry 2003, 83, 371.
- Institute of Medicine. Dietary Reference Intakes for Water, Potassium, Sodium Chloride and Sulfate; 1st ed.; The National Academies Press: Washington, DC, 2005.
- Liu, B.; Du, J.; Zeng, J.; Chen, C.; Niu, S. Characterization and Antioxidant Activity of Dihydromyricetin-Lecithin Complex. European Food Resource and Technology 2009, 230, 325–331.
- Suliman, M.A.; Tinay, A.H.; Elkhalif, A.O.; Babiker, E.E.; Elkhalil, E.A.I. Solubility as Influenced by pH and NaCl Concentration and Functional Properties of Lentil Proteins Isolate. Pakistan Journal of Nutrition 2006, 5, 589–593.
- Choudhary, K.A.; Bandyopadhyay, N.G. Preliminary Studies on the Inorganic Constituents of Some Indigenous Hyperglycaemic Herbs on Oral Glucose Tolerance Test. Journal of Ethnopharmacology 1999, 64, 179–184.
- Cheftel, J.C.; Cuq, J.L.; Lorient, D. Food Chemistry: Amino Acids, Peptides and Proteins. Eds. Fennema, O.R. New York, NY: Marcel Dekker, 1985, pp. 127−228.