ABSTRACT
Mulberry leaf protein fractions were extracted by the ultrasonic cell disrupter-assisted Osborne method. The physicochemical, functional properties, and antioxidant activities of mulberry leaf protein fractions were investigated. Albumin and glutelin were the predominant fractions and contained higher contents of essential amino acids (above 300 g kg−1). The solubility, water holding capacity, and emulsion stability of mulberry leaf protein fractions followed an order of albumin > globulin > glutelin > prolamin. All fractions exhibited significantly higher oil-holding capacity than soy protein isolates (p < 0.05). Furthermore, the radical scavenging activities of albumin were comparable to that of L-glutathione. Therefore, mulberry leaf protein fractions especially albumin showed encouraging functional properties and antioxidant activities, and could be considered as potential food ingredients and antioxidants.
Introduction
In recent years, seeking sources of new plant proteins has attracted increasing interests due to the ever-increasing protein consumption and the growing concerns over the safety of animal-derived products. Leaf proteins, the concentrated products of plant leaves, are the most abundant and renewable proteins in nature and can serve as a better alternative to the expensive animal- and cereal-based proteins. Moreover, leaf proteins have become a major source of dietary protein in many developing countries and could potentially form a major protein source for food applications.[Citation1] Until now, various plant leaves have been used to extract leaf proteins.[Citation2–Citation4]
Mulberry, a Moraceous plant, is cultivated over the world especially in Asia, Africa, and Latin America. All parts of the mulberry, including the roots, bark, fruits, and leaves, are good sources of bioactive compounds and have high economic values.[Citation5–Citation7] Mulberry leaf, the main product of mulberry, is not only the staple food for silkworms, but also a Chinese medicine to treat a fever and protect the liver.[Citation7] Moreover, mulberry leaf is consumed as a functional food for its high nutritional value, such as a mulberry leaf beverage and mulberry leaf tea.[Citation8,Citation9] On the other hand, mulberry leaf is considered as a potential plant protein source for industrial consumptions due to the high protein contents and in vivo digestibility.[Citation10,Citation11] Mulberry leaves are rich in proteins, polysaccharides, alkaloids, polyphenols, and other bioactive compounds. Until now, most studies were focused on the isolation and bioactivity determination of polysaccharides, γ-aminobutyric acid, and phenolic compounds of mulberry leaf.[Citation12–Citation14] However, there is little information about mulberry leaf protein (MLP) and mulberry leaf protein fractions (MLPFs). Several studies have reported that mulberry leaf contained high contents of crude protein (accounting for 17–25% of the dry matter);[Citation15,Citation16] and MLP had a good balance in essential amino acids (EAAs).[Citation17] Meanwhile, Kandylis et al.[Citation10] reported that MLP contained four fractions (albumin, globulin, glutelin, and prolamin), whereas no more information on MLPFs was provided. Considering the easy availability, high quality, and limited investigation of MLP, studies on MLP are necessary. Protein fractions can be extracted by the classical Osborne method, which separated the proteins according to their solubility in different systems. Compared with the traditional Osborne classification procedure, ultrasonic assisted extractions can more effectively break the cells and release the cells’ contents into the extraction medium, thus improving the extraction rate and reducing the extraction time.[Citation18,Citation19]
In general, to understand the nature of a protein, the properties, including microstructure, amino acid composition, and thermal characteristics, should be studied. Meanwhile, to better evaluate the quality and technological usability of food proteins, the functional properties especially the solubility, water holding, and emulsification should be investigated. Until now, physicochemical and functional properties of plant proteins, especially grain proteins, have been studied.[Citation20–Citation24] However, there are few investigations on the physicochemical and functional properties of MLP. Furthermore, to better assess the efficiency of proteins as food additives, especially as antioxidants, the bioactivity especially the antioxidant activity should be analyzed. In recent years, several studies have reported the antioxidant activity of plant proteins[Citation24–Citation26] and mulberry leaf solvent extracts.[Citation27,Citation28] Nevertheless, research on the antioxidant ability of MLP is still rare. Therefore, the objectives of this study were (1) to obtain protein fractions from mulberry leaf by Osborne extraction assisted with ultrasonic cell disrupter; and (2) to study the physicochemical, functional properties, and antioxidant activity of MLPF as indicators of its potential use in the food industry.
Materials and methods
Materials
Mulberry (Morus atropurpurea Roxb.) leaves were supplied by sericulture academy. All leaves were washed and dried in a drum wind-drying oven at 50°C for 48 h. The material was ground and sieved (60-mesh) to get dried mulberry leaf flour (MLF). 1,1-diphenyl-2-picrylhydrazyl (DPPH) and L-glutathione (GSH) were purchased from Sigma-Aldrich Co. (St. Louis, MO, USA). Soy protein isolates (SPI) and other main chemicals were of analytical grade and obtained from Aladdin Co. (Shanghai, China).
Ultrasonic cell disrupter-assisted extraction of protein fractions
The protein fractions were extracted according to the Osborne method, as described by Adebiyi et al.,[Citation29] with some modifications. First, the MLF (100 g) was extracted with 1.5 L distilled water. The mixture was disrupted 15 min with an ultrasonic cell disrupter and blended 1 h at room temperature with magnetic stirring. Then, the extraction was centrifuged (8000 × g, 10 min) and filtered (500-mesh) to get albumin extract (pH 7.49). The residue was successively extracted with 1.2 L NaCl (0.5 M) and 1.2 L NaOH solution (0.05 M). After blending for 1 h with magnetic stirring, it was centrifuged, as previously described, to obtain globulin (pH 7.96) and glutelin extract (pH 10.52), respectively. The precipitated residue was dispersed in 70% ethanol (1.0 L), then blended and centrifuged as previously described. The supernatant was concentrated by vacuum rotary evaporation to remove ethanol and to obtain prolamin extract. Each extract was precipitated with solid ammonium sulfate (for 65% saturation) and centrifuged at 12,000 × g for 20 min. The precipitates were pooled and dialyzed (using dialysis membrane of 8 kDa molecular weight cut-off) against distilled water for 48 h to desalinization. The desalted solution was pooled and freeze-dried to get albumin, globulin, glutelin, and prolamin, respectively. All these four fractions were considered as soluble proteins.
Analysis of chemical compositions
The percentages of moisture, crude protein, lipid, and ash in MLF and MLPFs were determined according to the Association of Official Analytical Chemists (AOAC) procedures.[Citation30] The protein/nitrogen coefficient used was 6.25. The total sugar content was determined by phenol-sulfuric acid method.[Citation31] The total phenolic content (TPC) was measured by the Folin-phenol method described by Ma et al.,[Citation32] results were expressed as grams of gallic acid equivalents (GAE) per kilogram of dry material (g GAE/kg).
Amino acid analysis
Amino acids of MLPFs were performed on an A300 auto amino acid analyzer equipped with a T260N column (4.6 × 100 mm). The results of amino acid composition were expressed as g kg−Citation1 protein. Amino acid score (%) was calculated according to Food and Agriculture Organization (FAO)/World Health Organization (WHO).[Citation33]
Thermal characteristics
Thermal properties of MLPFs were analyzed using a differential scanning calorimeter (DSC-Q200, America) by the method of Adebiyi et al.[Citation29] with a little modification. First, 2.5 mg of MLPF was accurately weighed into an aluminium pan and all samples were hermetically sealed before heating. An empty, sealed aluminium pan was marked as a reference. Then, sample pans were heated from 20 to 150°C at a heating rate of 5°C/min. The denaturation temperature (Td) was computed from each thermogram by the Universal Version 4.5 Analysis Program (TA Instruments). All samples were analyzed in triplicate.
Microstructure analysis
Microstructures of MLPFs were analyzed by scanning electron microscope (SEM). All MLPFs were sputter-coated with gold and examined in a SEM (HITACHI-S-3700N, Japan) at 20 kV.
Determination of functional properties
Functional properties were determined as protein solubility following the method of Adebowale et al.,[Citation34] water-holding capacity (WHC)/oil-holding capacity (OHC) following the method of Ajibola et al.,[Citation21] emulsification activity (EA) and emulsification stability (ES) according to the method of Viuda-Martos et al.,[Citation35] and foaming ability (FA) and foaming stability (FS) following the method of Lamsal et al.,[Citation4] SPI was chosen as a reference.
Determination of radical scavenging activity
The radical scavenging activities including DPPH and hydroxyl radical scavenging capacity were investigated. DPPH scavenging activity was determined using the method of Chen et al.[Citation36] Hydroxyl radical quenching ability was measured by the method of You et al.[Citation37] GSH was chosen as the positive reference. The experiments were carried out in triplicate. The relative half inhibition concentration (IC50) was calculated.
Reducing power
The ferric ion reducing power was assessed according to the method reported by Xie et al.[Citation38] GSH was chosen as the reference.
Statistical analysis
Statistical calculations were performed using SPSS Statistics 17.0 for one-way analysis of variance (ANOVA). The data presented were mean ± standard deviation (SD). Duncan’s multiple range tests were used to identify significant differences (p < 0.05) among treatment means.
Results and discussion
Chemical compositions
The chemical components of MLF and MLPFs are shown in . The contents of ash, lipid, and moisture of MLF were 116.53, 69.80, and 107.20 g kg−Citation1, respectively. The crude protein content of MLF was 243.41 g kg−Citation1, close to the result (251.00 g kg−Citation1) reported by Wang et al.,[Citation16] but higher than that (117.50–237.20 g kg−Citation1) found in many other mulberry species.[Citation10,Citation39] In the Osborne extraction, the major fraction was albumin at 50.54% protein yield and the next was glutelin at 31.62%, followed by globulin (11.89%) and prolamin (5.95%). These results are in disagreement with those of Kandylis et al.,[Citation10] who obtained the protein fractions from mulberry (morus alba) leaf and found that prolamin and glutelin showed the highest and the lowest protein yields, respectively. This difference may be due to the fact that the mulberry leaf species and extraction conditions were different. The protein content of glutelin was the highest (801.42 g kg−Citation1) compared with albumin (703.20 g kg−Citation1), globulin (787.80 g kg−Citation1), and prolamin (632.83 g kg−Citation1). The protein contents of glutelin and globulin are similar with that of MLPs (797.20 g kg−Citation1) extracted by NaCl solution.[Citation40] The lower protein purity of the albumin and prolamin means the higher concentration of non-protein components. The moisture contents of these four fractions ranged from 71.21 to 79.03 g kg−Citation1, with prolamin having the highest moisture content. The sugar content of albumin, globulin, glutelin, and prolamin was 47.90, 46.90, 48.70, and 41.10 g kg−Citation1, respectively. These values were lower than the 164 g kg−Citation1 obtained for the mulberry (Morus alba) leaf.[Citation41] In terms of TPC, prolamin showed the highest amount at 31.21 g GAE/kg, followed by globulin (26.21 g GAE/kg), glutelin (20.92 g GAE/kg), and albumin (17.72 g GAE/kg).
Table 1. Chemical compositions of mulberry leaf flour (MLF) and protein fractionsCitation1.
Amino acids analysis
The amino acids of MLF and MLPF are presented in and . MLF, albumin, globulin, and glutelin showed similar amino acid compositions. In these samples, Glu was the most abundant acid, the next was Asp. This pattern is similar to the amino acid profiles of 10 kinds mulberry leaf established by Zhang et al.[Citation17] and Akebia trifoliata var. australis seed protein fractions reported by Du et al.[Citation22] For prolamin, Arg was the maximum amino acid (154.40 g kg−Citation1) which has been reported to have potential preventative functions against heart disease.[Citation42] Cys, was absent in the glutelin and prolamin (). In terms of the EAAs, albumin, globulin, and glutelin exhibited relatively high amount of Thr, Leu, Val, and Lys, and the ratio of all EAAs contained in these three protein fractions were adequate for adults. Therefore, MLPFs were excellent protein supplements with high nutritive values. The highest content of EAA in prolamin was Phe which was more than five times higher than that of globulin. As a result, prolamin fraction contained the highest amount (134.30 g kg−Citation1) of aromatic amino acids (AAAs) among the four MLPFs, which was higher than that of MLP at 76.00 g kg−Citation1[Citation41] but lower than that of australis seed prolamin at 235.10 g kg−Citation1.[Citation22] Chavan et al.[Citation43] reported that the cultivars containing higher albumin and glutelin fractions would result in improved Met and Cys content in the seeds of pea legumes. In regard to the contents of sulphur amino acids (SAAs), MLF showed significantly higher value (32.50 g kg−Citation1) than the four MLPFs (; p < 0.05) and the freeze-dried mulberry (Morus alba) leaf (29.00 g kg−Citation1).[Citation41] Among these four fractions, glutelin showed the highest amount at 13.60 g kg−Citation1, the next was albumin at 12.80 g kg−Citation1, followed by globulin (10.40 g kg−Citation1) and prolamin (4.00 g kg−Citation1). This pattern is similar to the SAA profiles of beach pea protein fractions.[Citation43] The content of hydrophobic amino acids (HAAs) of glutelin (373.80 g kg−Citation1) and albumin (311.80 g kg−Citation1) was higher than that of globulin (268.1 g kg−Citation1) and prolamin (242.10 g kg−Citation1), which might lead to lower water solubility of glutelin and albumin.
Table 2. Amino acid compositions of mulberry leaf flour (MLF) and protein fractions (g kg −Citation1 of protein).
Table 3. Amino acid scores (%) of mulberry leaf flour (MLF) and protein fractions to the FAO/WHO pattern for (2–5-year-old child).
In respect to the nutritional parameter, SAA and Ile were found to be the first limiting amino acids for these four MLPFs and MLF, respectively (). Lys was the second limiting amino acid for all the five samples. This pattern was very different from that of freeze-dried mulberry (Morus alba) leaf, for which Lys and His were the first and the second limiting amino acids, respectively.[Citation41] While all the amino acid scores were relatively lower than that of australis seed protein fractions except for Thr and His which were the first and the third limiting amino acids in australis seed protein and prolamin, respectively.[Citation22]
Thermal analysis
Heating, the common way of food processing, caused disruptions of various chemical forces and produced endothermic peaks and exothermic process. DSC can be used to offer information about protein heated at different thermal conditions, and the Td can be used as an indicator of the thermal stability of proteins.[Citation4] The thermal characteristics of albumin (7.15% moisture), globulin (7.56% moisture), glutelin (7.12% moisture), and prolamin (7.90% moisture) were shown in . There was a significant difference in Td value for each sample (p < 0.05). Albumin showed the highest Td value at 72.96°C, the next was globulin at 69.92°C, followed by glutelin (65.03°C) and prolamin (60.87°C). This result is opposite to the conclusion that polar proteins possessed lower Td in contrast to less polar types.[Citation44] On the other hand, the Td values of these four fractions were lower than the dried rice bran protein fractions[Citation45] and the freeze-dried seinat seed protein isolate.[Citation46] The types of proteins, the sample preparation methods, and moisture contents are the main factors resulting in these differences.
Table 4. Functional properties and thermal characteristics of protein fractions and soy protein isolates (SPI).
Microstructures of MLPF
As shown in ,
Figure 1. SEM images of mulberry leaf protein fractions. (A) albumins; (B) globulins; (C) glutelins; (D) prolamins.
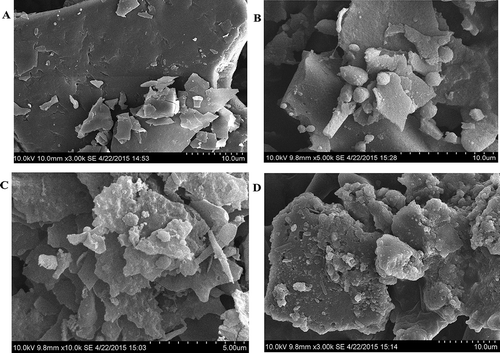
Protein solubility
Protein solubility is probably the most crucial factor for understanding the functional performance of proteins in food systems since it affects other properties, such as foaming, emulsification, and water and fat binding. High solubility is required for proteins used as functional ingredients in many foods. Solubility is the result of surface-active properties of a protein, and is influenced by amino acid composition and distribution, molecular flexibility, shape, and size.[Citation4] The water solubility of MLPFs are shown in . There were remarkable differences (p < 0.05) in solubility for albumin, globulin, glutelin, and prolamin. Albumin and globulin showed higher solubility at 74.13 and 63.15%, respectively, followed by glutelin (55.59%) and prolamin (43.37%). The solubility of MLPFs are higher than that of walnut protein (~40%).[Citation24] Meanwhile, the solubility values of albumin and globulin are comparable to that of rice bran protein hydrolysates (61–73%) at pH 7.[Citation48] The solubility differences between MLPFs may be caused by the differences in amino acid compositions (), microstructures ()
Figure 2. Foaming stability (FS) of soy protein isolate (SPI) and four protein fractions within 120 min.
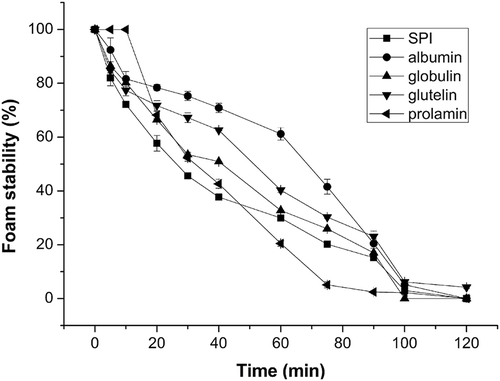
WHC and OHC
WHC and OHC of MLPFs are shown in . Results indicated that all samples except for SPI owned higher OHC than WHC. Compared with SPI, the OHC of MLPFs were significantly higher while the WHC were markedly lower than that of SPI (p < 0.05). Significant differences were found between the four MLPFs (p < 0.05; ). Glutelin showed the highest (4.48 g/g) OHC, followed by prolamin (4.02 g/g), albumin (3.46 g/g), and globulin (2.92 g/g). These results are higher than the values of 2.77 g/g reported for safflower protein isolate,[Citation20] but are lower than the 4.75 g/g for African yam bean seed globulin.[Citation21] OHC is the ability of fat to bind non-polar side chains of proteins, both physical entrapment of oil and mechanisms that involve protein size, charge, and hydrophobicity are contributed to OHC.[Citation21] Therefore, the higher OHC of glutelin is closely related to its microstructure characteristic () and the higher contents of HAA () since the rough surface and irregular networks possess a good physical retention ability with oil. High OHC is desirable for using in cold meat industry since protein can bridge the fat and water in these products.[Citation49]
WHC is a crucial function of protein and is considered critical in viscous foods, such as soups, gravies, and baked products, especially when its values ranging from 1.49 to 4.71 g/g.[Citation50] Albumin exhibited the highest WHC at 2.67 g/g, which is higher than 2.22 g/g reported for safflower protein isolate[Citation20] and 2.10 g/g for pea protein concentration.[Citation51] The WHC of globulin, glutelin and prolamin was 1.69, 1.52, and 1.05 g/g, respectively. The major factors affecting WHC are hydrophilic and hydrophobic balance, protein structure, and unfolding, as well as the presence of non-protein components.[Citation51] According to the present research, WHC might be mainly related to protein solubility since protein fractions with lower solubility showed lower WHC. This conclusion is in agreement with that reported by Chandi et al.,[Citation45] while is opposite to the findings of Ajibola et al.[Citation21]
Emulsification property
The EA and ES of SPI, albumin, globulin, glutelin, and prolamin are shown in . ES refers to the ability of an emulsion to resist changes in its physicochemical properties over time.[Citation21] The four MLPFs showed significantly higher ES than SPI (p < 0.05). Among these MLPFs, albumin showed the highest ES by 75.00%, the next was globulin (68.12%), and glutelin (60.43%), followed by prolamin (45.64%). Thus, the albumin and globulin can be confirmed as a stable emulsifier when compared to the glutelin and globulin. Similar results were reported by Malomo et al.[Citation52] Ajibola et al.[Citation21] reported that the presence of higher levels of non-protein components might have contributed to the better ES. showed that both albumin and prolamin had significantly lower protein purity than glutelin and globulin. However, the ES values of albumin and prolamin were the highest and the lowest among the four MLPFs (). On the other hand, the ES order of MLPFs was consistent with the Td order (). This mean that the high thermal stability of MLPF could contribute to a good ES. Therefore, the better ES of albumin may be due to the combined influence of high non-protein component contents and the good thermal stability. For albumin, globulin, and glutelin, the EA were lower than the ES due to the strengthening of emulsion by heating. This is in agreement with the observation of Lamsal et al.[Citation4] As to prolamin and SPI, the ES was lower than the EA. It might be due to the fact that their protein structures were destroyed by heating at 80°C. It was beneficial to observe that SPI showed lower EA than the MLPFs. Albumin, globulin, and glutelin had better ES than SPI and prolamin, indicating that these three proteins could be used as additives for the stabilization of emulsions in the production of soups and cakes.
Foaming property
As shown in , FA of glutelin was 80.47%, significantly higher (p < 0.05) than that of SPI (31.82%) and other three protein fractions, which was also higher than that of mucuna seed flour[Citation34] and hemp seed globulin.[Citation52] FA of albumin (26.04%) was similar with this of globulin (26.52%) and remarkably higher than that of prolamin (11.28%). Ajibola et al.[Citation21] reported that solubility is a pre-requisite for good foaming properties and the higher contents of AAA and HAA probably decreased the FA value. The lower FA of prolamin was consistent with the lower solubility, suggesting that limited interactions with the aqueous phase reduced the ability of protein molecules to encapsulate air particles. A similar result was reported by Malomo et al.[Citation52] Nevertheless, although albumin and globulin showed significantly higher solubility than glutelin, the FA of glutelin was remarkably higher than these two fractions (p < 0.05). On the other hand, the FA order of these four fractions was consistent with the protein contents order ( and ). It is possible that high protein purity can enhance the interactions of molecules with the aqueous phase. Therefore, the FA of MLPF may be attributed to the combined impact of solubility and protein purity. The mechanisms of emulsification were different from that of foam formation.[Citation21,Citation52] Compared the results of EA and FA (), it was found that prolamin showed the highest EA (61.25%) while having the lowest FA (11.28%). In contrast, glutelin had the lowest EA (55.65) but the highest FA (80.47%). This indicated that a higher emulsion-forming ability did not necessarily translate to a better foaming capacity. A similar conclusion was also reported by Ajibola et al.[Citation21]
The FS is important since the success of whipping agents depends on their ability to maintain whip as long as possible. FS is influenced by the type of protein, pH, net charge on proteins in the interface and the processing methods.[Citation46,Citation52] Decreased electrostatic repulsion caused packing of compact protein molecules into the interface to a greater extent and formed stable films.[Citation4] FS of MLPFs are shown in . Regardless of the protein kinds, FS of SPI and MLPFs gradually decreased with time. The FS of MLPFs within 10 min followed an order of prolamin > albumin > globulin > glutelin, which was opposite to the FA order. FS of SPI, globulin and prolamin declined sharply after 20 min. Moreover, the foam volume of these three proteins practically reached 0 after 120 min. The foam volume of albumin and glutelin was stable at above 50% within 40 min and dropped rapidly after 90 min. In time range of 20–75 min, albumin showed the highest FS (78–42%), the next was glutelin (70–30%) and globulin (65–25%), while SPI and prolamin showed the lowest FS in the time range of 20–40 and 60–75 min, respectively. When the time reached 90 min, the FS followed an order of glutelin > albumin > globulin > prolamin. In the time range of 0–120 min, the albumin showed significant higher FS than globulin (p < 0.05), a similar result was also reported by Lawal et al.[Citation53] In contrast, the albumin fraction of hemp seed was reported to form less stable foams than globulin.[Citation52] In general, the foaming property of glutelin was better than that of SPI and another three protein fractions.
Antioxidant activities
In order to better assess the potential of MLPFs as food additives, the antioxidant activities, including radical scavenging activities ()
Figure 3. Radical scavenging activity of four protein fractions and GSH (0.05- 0.60 g L-1). (A) DPPH radical scavenging activity; (B) Hydroxyl radical scavenging activity. Vertical bars show the standard deviations from three determinations.
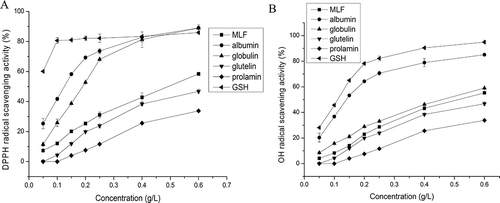
Figure 4. Ferric ion reducing power of four protein fractions and GSH (0.05- 0.60 g L-1). Vertical bars show the standard deviations from three determinations.
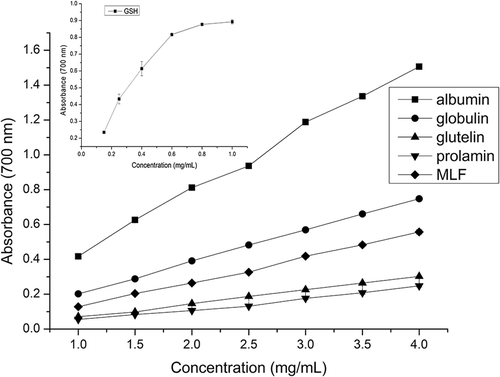
DPPH radical scavenging activity
The DPPH radical in methanol is relatively stable and has been widely used to test the ability of compounds to act as hydrogen donors and free radical scavengers.[Citation36] As shown in Fig. 3a, the DPPH radical inhibition rate of MLF and MLPF increased rapidly when the concentration ranged from 0.05 to 0.40 g L−Citation1. Compared with MLPFs, the DPPH radical scavenging ability of MLF was higher than that of glutelin and prolamin but lower than that of albumin and globulin. Among the four MLPFs, albumin showed the highest DPPH scavenging activity followed by globulin, glutelin, and prolamin. This order was consistent with the solubility order (). Namely, the higher the solubility caused the higher the DPPH quenching ability. Bamdad et al.[Citation54] reported that DPPH scavenging ability of protein hydrolysates is associated with the balance between high levels of hydrophobicity and good diffusivity in reaction medium. In this work, the higher DPPH radical scavenging activity of albumin may be attributed to the higher contents of HAAs and AAAs (), since they can easily scavenge DPPH radicals. Meanwhile, the appropriate solubility of albumin () makes it easier to contact DPPH radicals. Wang et al.[Citation55] reported that some amino acids especially His, Tyr, Val, Pro, Met, and Cys exhibited strong radical scavenging activity. It was found that albumin and globulin exhibited higher contents of His, Tyr, Met, and Cys than glutelin and prolamin (). Therefore, the DPPH quenching ability of MLPF might be the combined consequences of amino acid compositions and solubility. The IC50 values of albumin and globulin were 0.13 and 0.19 g L−Citation1, respectively, which were lower than that of alfalfa leaf peptides (0.80 g L−Citation1).[Citation38] Meanwhile, the DPPH radical inhibition values of MLF, albumin, globulin, glutelin, and prolamin reaching their maximum (58.43, 89.06, 88.97, 46.74, and 33.78%) at the concentration of 0.60 g L−Citation1. The maximum values of albumin and globulin are higher than that of curry leaf protein at 0.11 g L−Citation1 (80%)[Citation2] and walnut protein at 0.125 g L−Citation1 (38%).[Citation24] Besides, the DPPH scavenging activity of albumin at 0.10 g L−Citation1 was 41.69% and was higher than that of mulberry leaf water extracts (30%) at 0.10 g L−Citation1,[Citation28] indicating that proteins in the water extracts might play an important role in its DPPH scavenging activity. MLF and the four MLPFs showed significantly lower DPPH quenching capacity than GSH when the concentration was below 0.25 g L−Citation1. When the concentration reached 0.60 g L−Citation1, the DPPH quenching ability of albumin and globulin was higher than that of GSH. Therefore, both albumin and globulin could be used as potential hydrophobic antioxidants.
Hydroxyl radical scavenging activity
Hydroxyl radicals, as the most reactive oxygen radicals, easily react with bimolecular and induce severe damage to cells.[Citation37] Therefore, the removal of the hydroxyl radical is very important. The .OH inhibition abilities of MLF and MLPFs are shown in Fig. 3b, following the same order with DPPH quenching activity. Namely, albumin > globulin > MLF > glutelin > prolamin. All five of the samples showed lower .OH scavenging abilities than GSH. The .OH inhibition rate of GSH and albumin increased rapidly at the concentration of 0.05–0.20 g L−Citation1 and then continued at a steadily increasing level when the concentration was over 0.20 g L−Citation1. The hydroxyl radical scavenging values of MLF, albumin, globulin, glutelin, and prolamin reaching their maximum (55.3, 85.1, 59.0, 46.7, and 33.8%) at the concentration of 0.6 g L−Citation1. The maxima value of albumin is higher than that of alfalfa leaf peptides (80%; 1.2 g L−Citation1) and loach proteins (78.9%; 2.0 g L−Citation1).[Citation37] Meanwhile, the IC50 value of albumin (1.46 g L−Citation1) was comparable to that of GSH (1.13 g L−Citation1). This means that the albumin can be an effective hydrogen or electron donor even at very low concentrations.
Reducing power
As shown in Fig. 4, the reducing power of albumin was remarkably higher than that of MLF and the other three fractions at their corresponding concentration. Meanwhile, the order of reducing power among MLPFs was in agreement with the ability to scavenge DPPH radicals; similar results were obtained by Wang et al.[Citation24] This mean that the amino acid compositions and solubility of MLPF, were not only beneficial to quench DPPH radicals, but also to reduce ferric ion. At the concentration of 4.00 g L−Citation1, MLF, albumin, globulin, glutelin, and prolamin reached the highest reducing values of 0.557, 1.506, 0.748, 0.302, and 0.249, respectively. Except for albumin, these highest values were significantly lower than GSH at 1.0 g L−Citation1 (0.892, Fig. 4) and curry leaf protein at 0.09 g L−Citation1 (1.420),[Citation2] but higher than walnut protein at 0.02 g L−Citation1 (0.247). [Citation24] When the concentration was 2.0 g L−Citation1, the reducing power of albumin reached 0.812, which was comparable with GSH at 0.6 g L−Citation1 (0.817).
Conclusion
Protein fractionation showed that albumin and glutelin were the prominent fractions. The aromatic and HAAs were higher in the albumin than globulin. Although Cys was absent from glutelin and prolamin, the amino acid compositions in these MLPFs were desirable. In terms of functional properties, albumin showed the highest WHC and ES solubility. The better ES of albumin may be due to the combined influence of high non-protein component contents and the good thermal stability. Prolamin had the highest EA and the lowest FA, glutelin showed the highest OHC and FA, while having the lowest EA. Compared with SPI, MLPF exhibited higher OHC, EA, and FS. Combined with the result of the amino acid profiles, these protein fractions were believed to have high application value in food additive consumptions. Meanwhile, the DPPH and hydroxyl radicals scavenging abilities of albumin were comparable to that of GSH. Therefore, albumin can be used as a low-cost hydrophobic antioxidant. Further research on the isolation and purification of MLP and preparation of antioxidant peptides from mulberry leaf albumin are necessary to better assess the use of this protein as a natural antioxidant additive source in human consumption.
Supplementary_figure.tif
Download TIFF Image (9.2 MB)Additional information
Funding
References
- Tenorio, A.T.; Gieteling, J.; de Jong, G.A.H.; Boom, R.M.; van der Goot, A.J. Recovery of Protein from Green Leaves: Overview of Crucial Steps for Utilisation. Food Chemistry 2016, 203, 402–408.
- Ningappa, M.B.; Srinivas, L. Purification and Characterization of Similar to 35 kDa Antioxidant Protein from Curry Leaves (Murraya Koenigii L.). Toxicology in Vitro 2008, 22, 699–709.
- Teixeira, E.M.B.; Carvalho, M.R.B.; Neves, V.A.; Silva, M.A.; Arantes-Pereira, L. Chemical Characteristics and Fractionation of Proteins from Moringa Oleifera Lam. Leaves. Food Chemistry 2014, 147, 51–54.
- Lamsal, B.P.; Koegel, R.G.; Gunasekaran, S. Some Physicochemical and Functional Properties of Alfalfa Soluble Leaf Proteins. LWT–Food Science and Technology 2007, 40, 1520–1526.
- Raman, S.T.; Ganeshan, A.K.P.G.; Chen, C.; Jin, C.; Li, S.H.; Chen, H.J.; Gui, Z. In Vitro and in Vivo Antioxidant Activity of Flavonoid Extracted from Mulberry Fruit (Morus Alba L.). Pharmacognosy Magazine 2016, 12, 128–133.
- Turgut, N.H.; Mert, D.G.; Kara, H.; Egilmez, H.R.; Arslanbas, E.; Tepe, B.; Gungor, H.; Yilmaz, N.; Tuncel, N.B. Effect of Black Mulberry (Morus Nigra) Extract Treatment on Cognitive Impairment and Oxidative Stress Status of D-Galactose-Induced Aging Mice. Pharmaceutical Biology 2016, 54, 1052–1064.
- Chang, L.W.; Juang, L.J.; Wang, B.S.; Wang, M.Y.; Tai, H.M.; Hung, W.J.; Chen, Y.J.; Huang, M.H. Antioxidant and Antityrosinase Activity of Mulberry (Morus Alba L.) Twigs and Root Bark. Food and Chemical Toxicology 2011, 49, 785–790.
- Phoonan, W.; Deowanish, S.; Chavasiri, W. Food Attractant from Mulberry Leaf Tea and Its Main Volatile Compounds for the Biocontrol of Lasioderma Serricorne F. (Coleoptera: Anobiidae). Journal of Stored Products Research 2014, 59, 299–305.
- Wanyo, P.; Siriamornpun, S.; Meeso, N. Improvement of Quality and Antioxidant Properties of Dried Mulberry Leaves with Combined Far-Infrared Radiation and Air Convection in Thai Tea Process. Food and Bioproducts Processing 2011, 89, 22–30.
- Kandylis, K.; Hadjigeorgiou, I.; Harizanis, P. The Nutritive Value of Mulberry Leaves (Morus Alba) As a Feed Supplement for Sheep. Tropical Animal Health and Production 2009, 41, 17–24.
- Vu, C.C.; Verstegen, M.W.A.; Hendriks, W.H.; Pham, K.C. The Nutritive Value of Mulberry Leaves (Morus Alba) and Partial Replacement of Cotton Seed in Rations on the Performance of Growing Vietnamese Cattle. Asian-Australasian Journal of Animal Sciences 2011, 24, 1233–1242.
- Zhang, D.Y.; Wan, Y.; Xu, J.Y.; Wu, G.H.; Li, L.;Yao, X.H. Ultrasound Extraction of Polysaccharides from Mulberry Leaves and Their Effect on Enhancing Antioxidant Activity. Carbohydrate Polymers 2016, 137, 473–479.
- Sanchez-Salcedo, E.M.; Mena, P.; Garcia-Viguera, C.; Hernandez, F.; Jose Martinez, J. (Poly)phenolic Compounds and Antioxidant Activity of White (Morus Alba) and Black (Morus Nigra) Mulberry Leaves: Their Potential for New Products Rich in Phytochemicals. Journal of Functional Foods 2015, 18, 1039–1046.
- Yang, N.C.; Jhou, K.Y.; Tseng, C.Y. Antihypertensive Effect of Mulberry Leaf Aqueous Extract Containing γ-Aminobutyric Acid in Spontaneously Hypertensive Rats. Food Chemistry 2012, 132, 1796–1801.
- Sun, C.Z.; Wu, W.J.; Min, T.; Liu, Y.; Zhu, J.H.; Lai, F.R.; Wu, H. Functional Properties of Mulberry (Morus Atropurpurea Roxb.) Leaf Proteins Extracted by Different Methods. Modern Food Science and Technology 2015, 31, 235–241.
- Wang, W.X.; Yang, H.J.; Bo, Y.K.; Ding, S.; Cao, B.H. Nutrient Composition, Polyphenolic Contents, and in Situ Protein Degradation Kinetics of Leaves from Three Mulberry Species. Livestock Science 2012, 146, 203–206.
- Zhang, L.L.; Bai, Y.L.; Shu, S.L.; Qian, D.W.; Zhen, O.Y.; Liu, L.; Duan, J.A. Simultaneous Quantitation of Nucleosides, Nucleobases, Amino Acids, and Alkaloids in Mulberry Leaf by Ultra High Performance Liquid Chromatography with Triple Quadrupole Tandem Mass Spectrometry. Journal of Separation Science 2014, 37, 1265–1275.
- Toma, M.; Vinatoru, M.; Paniwnyk, L.; Mason, T.J. Investigation of the Effects of Ultrasound on Vegetal Tissues During Solvent Extraction. Ultrasonics Sonochemistry 2001, 8, 137–142.
- Vilkhu, K.; Mawson, R.; Simons, L.; Bates, D. Applications and Opportunities for Ultrasound Assisted Extraction in the Food Industry—A Review. Innovative Food Science & Emerging Technologies 2008, 9, 161–169.
- Ulloa, J.A.; Rosas-Ulloa, P.; Ulloa-Rangel, B.E. Physicochemical and Functional Properties of a Protein Isolate Produced from Safflower (Carthamus Tinctorius L.) Meal by Ultrafiltration. Journal of the Science of Food and Agriculture 2011, 91, 572–577.
- Ajibola, C.F.; Malomo, S.A.; Fagbemi, T.N.; Aluko, R.E. Polypeptide Composition and Functional Properties of African Yam Bean Seed (Sphenostylis Stenocarpa) Albumin, Globulin and Protein Concentrate. Food Hydrocolloids 2016, 56, 189–200.
- Du, Y.; Jiang, Y.; Zhu, X.; Xiong, H.; Shi, S.; Hu, J.; Peng, H.; Zhou, Q.; Sun, W. Physicochemical and Functional Properties of the Protein Isolate and Major Fractions Prepared from Akebia trifoliata var. Australis Seed. Food Chemistry 2012, 133, 923–929.
- Rezig, L.; Riaublanc, A.; Chouaibi, M.; Guéguen, J.; Hamdi, S. Functional Properties of Protein Fractions Obtained from Pumpkin (Cucurbita Maxima) Seed. International Journal of Food Properties 2016, 19, 172–186.
- Wang, X.; Chen, H.; Li, S.; Zhou, J.; Xu, J. Physico-Chemical Properties, Antioxidant Activities and Antihypertensive Effects of Walnut Protein and Its Hydrolysate. Journal of the Science of Food and Agriculture 2016, 96, 2579–2587.
- Sarkar, M.K.; Kinter, M.; Mazumder, B.; Sil, P.C. Purification and Characterisation of a Novel Antioxidant Protein Molecule from Phyllanthus Niruri. Food Chemistry 2009, 114, 1405–1412.
- Zarena, A.S.; Gopal, S.; Vineeth, R. Antioxidant, Antibacterial, and Cytoprotective Activity of Agathi Leaf Protein. Journal of Analytical Methods in Chemistry 2014, 2014, 989543–989543.
- Andallu, B.; Shankaran, M.; Ullagaddi, R.; Iyer, S. In Vitro Free Radical Scavenging and in Vivo Antioxidant Potential of Mulberry (Morus Indica L.) Leaves. Journal of Herbal Medicine 2014, 4, 10–17.
- Arabshahi-Delouee, S.; Urooj, A. Antioxidant Properties of Various Solvent Extracts of Mulberry (Morus Indica L.) Leaves. Food Chemistry 2007, 102, 1233–1240.
- Adebiyi, A.P.; Adebiyi, A.O.; Hasegawa, Y.; Ogawa, T.; Muramoto, K. Isolation and Characterization of Protein Fractions from Deoiled Rice Bran. European Food Research and Technology 2009, 228, 391–401.
- AOAC. Official Method of Analysis. 15th ed., pp 66–68, Association of Official Analytical Chemists: Washington, DC, USA, 1990.
- Cuesta, G.; Suarez, N.; Bessio, M.I.; Ferreira, F.; Massaldi, H. Quantitative Determination of Pneumococcal Capsular Polysaccharide Serotype 14 Using a Modification of Phenol-Sulfuric Acid Method. Journal of Microbiological Methods 2003, 52, 69–73.
- Ma, Y.; Zhou, M.; Huang, H. Changes of Heat-Treated Soymilks in Bioactive Compounds and Their Antioxidant Activities Under in Vitro Gastrointestinal Digestion. European Food Research and Technology 2014, 239, 637–652.
- FAO/WHO. Protein and Amino Acid Requirements in Human Nutrition. World Health Organization Technical Report Series 2007; 1 p.
- Adebowale, Y.A.; Adeyemi, I.A.; Oshodi, A.A. Functional and Physicochemical Properties of Flours of Six Mucuna Species. African Journal of Biotechnology 2005, 4, 1461–1468.
- Viuda-Martos, M.; Ruiz-Navajas, Y.; Martin-Sanchez, A.; Sanchez-Zapata, E.; Fernandez-Lopez, J.; Sendra, E.; Sayas-Barbera, E.; Navarro, C.; Perez-Alvarez, J.A. Chemical, Physico-Chemical and Functional Properties of Pomegranate (Punica Granatum L.) Bagasses Powder Co-Product. Journal of Food Engineering 2012, 110, 220–224.
- Chen, N.; Zhao, M.; Sun, W. Effect of Protein Oxidation on the in Vitro Digestibility of Soy Protein Isolate. Food Chemistry 2013, 141, 3224–3229.
- You, L.; Zhao, M.; Regenstein, J.M.; Ren, J. Changes in the Antioxidant Activity of Loach (Misgurnus Anguillicaudatus) Protein Hydrolysates During a Simulated Gastrointestinal Digestion. Food Chemistry 2010, 120, 810–816.
- Xie, Z.; Huang, J.; Xu, X.; Jin, Z. Antioxidant Activity of Peptides Isolated from Alfalfa Leaf Protein Hydrolysate. Food Chemistry 2008, 111, 370–376.
- Guven, I. Effect of Species on Nutritive Value of Mulberry Leaves. Kafkas Universitesi Veteriner Fakultesi Dergisi 2012, 18, 865–869.
- Wang, F.; Li, H.; Dong, M. Functional Properties of Proteins from Mulberry Leaves. Food Science (in Chinese) 2010, 31, 81–86.
- Leterme, P.; Londono, A.M.; Estrada, F.; Souffrant, W.B.; Buldgen, A. Chemical Composition, Nutritive Value and Voluntary Intake of Tropical Tree Foliage and Cocoyam in Pigs. Journal of the Science of Food and Agriculture 2005, 85, 1725–1732.
- Pszczola, D.E. Genes and Diet: The Specialized Role Ingredients May Play. Food Technology 2000, 54, 82, 84, 86.
- Chavan, U.D.; McKenzie, D.B.; Shahidi, F. Protein Classification of Beach Pea (Lathyrus Maritimus L.). Food Chemistry 2001, 75, 145–153.
- del Angel, S.S.; Martinez, E.M.; Lopez, M.A.V. Study of Denaturation of Corn Proteins During Storage Using Differential Scanning Calorimetry. Food Chemistry 2003, 83, 531–540.
- Chandi, G.K.; Sogi, D. Functional Properties of Rice Bran Protein Concentrates. Journal of Food Engineering 2007, 79, 592–597.
- Siddeeg, A.; Xu, Y.; Jiang, Q.; Xia, W. Physicochemical and Functional Properties of Flour and Protein Isolates Extracted from Seinat (Cucumis Melo var. Tibish) Seeds. Food Science and Biotechnology 2014, 23, 345–353.
- Zhu, Z.; Yi, J.; Li, J. Fractionation and Structural Characterization of Peach Kernel Proteins. Application of Chemical Engineering 2011, 28, 1974–1977.
- Hamada, J.S. Characterization and Functional Properties of Rice Bran Proteins Modified by Commercial Exoproteases and Endoproteases. Journal of Food Science 2000, 65, 305–310.
- Ogunwolu, S.O.; Henshaw, F.O.; Mock, H.-P.; Santros, A.; Awonorin, S.O. Functional Properties of Protein Concentrates and Isolates Produced from Cashew (Anacardium Occidentale L.) Nut. Food Chemistry 2009, 115, 852–858.
- Aletor, O.; Oshodi, A.A.; Ipinmoroti, K. Chemical Composition of Common Leafy Vegetables and Functional Properties of Their Leaf Protein Concentrates. Food Chemistry 2002, 78, 63–68.
- Toews, R.; Wang, N. Physicochemical and Functional Properties of Protein Concentrates from Pulses. Food Research International 2013, 52, 445–451.
- Malomo, S.A.; Aluko, R.E. A Comparative Study of the Structural and Functional Properties of Isolated Hemp Seed (Cannabis Sativa L.) Albumin and Globulin Fractions. Food Hydrocolloids 2015, 43, 743–752.
- Lawal, O.S.; Adebowale, K.O. Ogunsanwo, B.M.; Sosanwo, O.A.; Bankole, S.A. On the Functional Properties of Globulin and Albumin Protein Fractions and Flours of African Locust Bean (Parkia Biglobossa). Food Chemistry 2005, 92, 681–691.
- Bamdad, F.; Wu, J.; Chen, L. Effects of Enzymatic Hydrolysis on Molecular Structure and Antioxidant Activity of Barley Hordein. Journal of Cereal Science 2011, 54, 20–28.
- Wang, J.S.; Zhao, M.M.; Zhao, Q.Z.; Jiang, Y.M. Antioxidant Properties of Papain Hydrolysates of Wheat Gluten in Different Oxidation Systems. Food Chemistry 2007, 101, 1658–1663.