ABSTRACT
The aim of this study was to test the applicability of bioelectrical measurements and a needle-type multi-electrode array in evaluating salt and moisture content of structurally heterogeneous food samples, such as fish. Impedance measurements were performed at different frequencies in order to find the most appropriate range, where impedance module and/or phase angle are the most sensitive to salt and moisture content. The salt, moisture, and water phase salt (WPS) of the salted rainbow trout samples were successfully evaluated by the impedance module measured at 50 kHz (AdR2 > 0.85). Due to rapid and non-destructive analysis coupled with low cost device design, this method for determination of salt and/or moisture has potential as the quality control technique for the fish industry.
Introduction
Salt is the most commonly used antimicrobial agent in fishery products, added during the production of smoked, marinated, canned, or salted fish. Determination of salt is, particularly, important for the fish industry because the amount of salt compared to the amount of moisture, called water phase salt (WPS), represents the critical limit in the HACCP programme for assuring the microbiological safety of fishery products. Several analytical methods, including drying methods (forced air drying, rapid microwave drying, and drying under vacuum), spectroscopic methods (near infrared transmittance and guided microwave spectrometry), and NMR have been used to determine the moisture content of fish and meat products. Although these methods have advantages for use in laboratory settings, the drying methods are time-consuming and require the sample to be destroyed whereas the spectroscopic methods and NMR often involve expensive equipment, which make these methods not applicable for the routine analysis by the industry.
Regarding the NaCl determination, analytical methods include hydrolysis–titration, X-ray techniques, optical atomic emission methods, ion chromatography, ion selective electrode (ISE), colourimetric methods etc. These analytical methods are either difficult to perform in the industry environment or are time-consuming or expensive, or again require the sample to be destroyed. Therefore, the development of fast, inexpensive, and non-destructive methods for determination of salt, moisture, and WPS for use in the fish industry has been in the focus of many studies.[1–4] One of such methods studied for the determination of salt/moisture in meat and fishery products has been the electrical impedance.[3–5]
Electrical impedance is a frequency dependent property of any material to resist electrical current flow. It can be defined as Z = R + jX or as Z = |Z|exp(jφ), where R is the resistance (real part), X is the reactance (imaginary part), |Z|is the impedance module (magnitude), and φ is the phase. The impedance module is inversely proportional to the conductivity. The conductivity is the proportionality factor between electric current and electric field, and is a measure of the ease with which delocalised charge carriers can move through the material under the influence of an electric field. [6–8] For biological materials, the conductivity arises mainly from the mobility of hydrated ions. The conductivity of biological tissues is ionic (electrolytic), because of Na+ and Cl– ions in the tissue. As the impedance module generally reflects the changes in the conductivity of tissues, by the measurements of the module, the salt content of food could theoretically be determined. Higher salt content due to the salting process increases tissue conductivity as there are more ions to migrate and consequently |Z|decreases. The phase is generally related to the states of cell membranes, but the measurements of the phase have been reported to improve the predictive equations when used together with the impedance module measurements.[3] However, impedance should be adequately measured in order to obtain repeatable results. Electrical impedance is the function of frequency, dielectric properties of the sample (i.e. electrical conductivity and permittivity), and electrode geometry.[9] Although impedance measurements are fast and easy to perform, challenges with the anisotropy of the samples and electrode polarisation should be overcome. Moreover, as salt is not homogeneously distributed through a meat or fish sample at the end of the salting process, the electrode should preferably be able to measure not only the surface, but also the depth of the samples. Impedance is also dependent on the temperature.[10] However, smaller temperature fluctuations do not seem to have a significant influence on the impedance results.[11]
Several authors measured impedance of salted meat or fish at different frequency ranges using different electrodes and reported linear models for prediction of salt with R2> 0.8 based on the impedance measured at two frequencies[3] or in a broader frequency range.[4] However, when cylindrical surface electrodes were used, moisture content was not as successfully predicted[3] and this surface electrode would not be applicable for the measurements of the gradient of salt through the sample. Masot et al.[4] obtained even better models with R2> 0.9 with a single needle coaxial electrode (that can be inserted into the sample) by measurements performed in a broad frequency range from 100 Hz to 1 MHz. However, with the single needle coaxial electrode, the distribution of the electric field is not homogeneous (as it is when parallel electrodes are used), which may have influenced the results. Kaltenecker et al.[5]measured the impedance of pork by a single pair of needle electrodes. However, when a single pair of needle electrodes arranged in a bipolar configuration is used, the current density close to the needles is high in comparison to the bulk, leading to a high focus in the measurements close to the needle. Tetrapolar systems that are traditionally believed to be polarisation-free have disadvantages, such as negative sensitivity, parasitic influence of the potential sensing electrodes etc., to successfully interpret the measured data.[12–14]
The aim of this study was to test if the salt and moisture content could be evaluated by the impedance measurements and another puncture probe – a needle-type multi-electrode array arranged in a bipolar configuration – as the geometry of this electrode could enable more accurate measurements of electrical properties and determination of salt and moisture of heterogeneous solid samples such as fish. The measurements will be performed in a broad frequency range and the most appropriate frequencies for the determination of salt, moisture, and WPS of brine-salted fish will be chosen as a basis for designing a portable industrial device.
Materials and methods
Materials and experimental plan
Sixty samples of rainbow trout (Oncorhynchus mykiss) with the average weight of 305 ± 35 g were purchased from the local fish market, transported to the laboratory on ice and stored in a refrigerator at 2°C. The measurements started on the 3rd day after the purchase, when all the fish were assessed as being in the post-rigor state. Wet salting procedure was used. The brine was prepared by dissolving sea salt in distilled water (w = 25%) and was stored in the refrigerator until it reached a temperature of 8°C.
The epaxial muscle of the fish with skin on was used for the experiment. The samples of approximate same size (~90 g) were placed in the brine (ratio brine/fish (w/w) = 2:1) and were salted for 2, 4, or 6 h at 8°C. Twenty samples were wet salted for 2 h, 20 for 4 h, and 12 for 6 h. Eight pieces were used as control samples. After salting for 2, 4, or 6 h, the samples were removed from the brine, rinsed gently with tap water, wiped off, weighted, wrapped in a plastic bag, and stored for 2 h in a refrigerator at 8°C.
Each sample was then cut into two equal halves. One of them was used for physicochemical measurements i.e. determination of salt, moisture, color, and pH measurements, the other one for electrical measurements. The difference in weight before and after the treatment was calculated according to:
where weight before wet-salting,
weight after wet-salting, and
weight.
WPS was calculated according to Hilderbrand,[15]
Electrical impedance spectroscopy measurements
The Agilent 4294A Precision Impedance Analyser was used to measure the electrical impedance of the samples. The instrument was computer controlled over the TCP/IP interface. A custom-made needle-type multi-electrode array, comprised of two rows of six parallel, electrically connected, gold-plated needles was connected to the Agilent 16047E test fixture making a bipolar measurement setup (). As mentioned in the Introduction, if only two needles had been used, the current density close to the needles is high in comparison to the bulk, leading to a high focus in the measurements close to the needle. With a needle array probe more representative results are obtained, as much larger sample volume contributes to the measured impedance.
Figure 1. Needle-type multi electrode array (12 gold-plated needles arranged in 2 rows of 6 electrically connected needles).
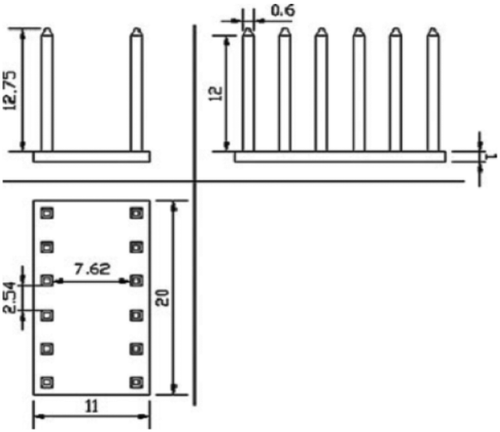
Prior to the impedance measurements on fish samples, open, and short corrections were made to compensate for parasitic impedances. The electrode was inserted in 50 mM and 142 mM NaCl solutions to assess the electrode polarisation that was found to be pronounced below 5 kHz and that diminishes at higher frequencies.[9,16] The electrode array was positioned transversally to the direction of the muscle fibres. The impedance module (|Z|) and phase (φ) were measured at 200 frequencies from 100 Hz to 100 MHz, however, we were focused on the frequencies above 5 kHz where electrode polarisation gradually diminishes. Measurements were performed thrice on each sample in the total length of 6 cm along the epaxial muscle. Temperature influences the impedance module as higher temperatures increase the mobility of ions and therefore the conductivity. Therefore, to control the stability of temperature, the temperature was recorded by two thermometers (Model 26000, Heat⁄Cool Thermometer DeltaTRAK and Model 13307, Thermo-Hygrometer, DeltaTRAK, Pleasanton, CA, USA). All the measurements were performed at T=8 ± 0.3°C.
Chemical composition analysis and colour
The moisture content and sodium chloride were determined according to AOAC methods.[16] In short, for moisture content determination, the samples were weighed and dried, then weighed again according to the standardised procedure. The loss of weight was used to calculate the moisture content of the samples. Mohr’s method, used for sodium chloride determination, determines the chloride ion concentration of a solution by titration with silver nitrate. Two replicates of each sample were analysed and the mean value was used in the data analyses.
The colour of skinned muscles was measured using a colourimeter (HunterLab, Model ColorFlex EZ, Virginia U.S.A) and reported in the CIE system colour profile of L* (lightness), a* (redness/greenness) and b* (yellowness/blueness). The Standard Illuminant D65 and 10° Standard Observers, 45º/0º optical geometry, Port Diameter/View Diameter: 31.8 mm (1.25 in) illuminated/25.4 mm (1 in) were used. Before the analysis, the HunterLab ColorFlex EZ spectrophotometer was standardised at the measurement port with black glass tile and then with white glass tile. For each sample, the colour was measured at three positions.
Statistical analysis
SPSS version 9.0 (Chicago, IL) was used for the statistical evaluation of the results. The data were initially screened for outliers. One observation was removed from the entire dataset (ΔM). The results of the physicochemical analysis were subjected to ANOVA to test the differences among the samples that had been brine salted up to 6 h. Prior to ANOVA, the normal distribution of data and the homogeneity of variances were confirmed.
Linear and multiple regression analyses were calculated to predict the salt and moisture content and WPS of the rainbow trout samples on the basis of their electrical properties i.e. impedance module and phase measured at 150 frequencies, in the frequency range 5 kHz–100 MHz.
The normality of distribution of outcome variables, homoscedasticity, multicollinerarity among predictor variables and linearity between the outcome and predictor variables were checked. As there is a limitation for the number of predictor variables in the multiple regression analysis (1 predictor variable per 15 observations, in total 4 predictor variables per 60 samples in this study), 3 models were tested differing by the predictor variables used.
Model A (Predictor variables were the PCs (or factors) obtained by the PCA of the impedance spectra): The PCA was performed to reduce the number of variables obtained by the impedance measurements recorded at 150 frequencies. The factors obtained by the PCA were used as predictor variables. As the PCA requires a limited number of variables depending on the number of observations, it was performed separately on the impedance module (|Z|) and the phase (φ) spectra.
Model B (Predictor variables were the average values of |Z|and φ obtained from four frequency ranges): A small sample size and a large number of variables in this study may be assessed as violations of the assumptions for performing the PCA (by some authors), so therefore another approach was used to extract as much as information from the impedance recordings i.e. the average values of the impedance module and phase measurements from four frequency ranges were calculated and used as predictors in the multiple regression analysis. This way, we extracted the most of information from both spectra. The four frequency ranges were 5–10 kHz, >10 kHz–1 MHz, >1 MHz–10 MHz, and >10 MHz–30 MHz.
Model C (Predictor variables were |Z|and φ recorded at a single or two frequencies): An industrial device used for the determination of the salt and moisture content of fish should be operable in a cold and humid environment, portable and cost-effective. Multi-frequency devices, especially the ones operating in a higher MHz range, extract much information but are expensive, and the difficulties with the parasitic impedances may be pronounced. Therefore, in this study, linear and multiple regression analyses were performed with a number of impedance module and phase measurements which had been recorded at a single or two frequencies to test if the impedance measurements recorded at one or few frequencies could equally successfully evaluate the salt and/or moisture content as the measurements recorded in a number of frequencies. The models were validated by bootstrapping and expressed as bootstrapped standard error of the predictors.
Results and discussion
The results of the physicochemical analysis of the epaxial muscle of the rainbow trout samples after wet salting up to 6 h are presented in . The wet salting caused changes in all the examined parameters. Larger differences in the average values of ΔM, salt, moisture, and WPS between 4 and 6 h indicate that mass transfer between the osmotic medium and the fish muscle is more pronounced in the latter stage of the process. The initial salt and moisture content of the control samples (n = 8) was 0.23 ± 0.01 and 77.6 ± 0.2 (results are not presented). The salt content of the control samples and the samples salted up to 6 h was in the range of 0.2–8.8 g/100g and the moisture content was in the range of 69.5–77.6 g/100g. The calculated WPS was in the range of 0.3–11.2. Salting also modifies the colour of the fish as a consequence of denaturation of proteins ().
Table 1. Physicochemical analysis of the epaxial muscle of the rainbow trout samples (N = 52) after wet salting up to 6 hours.
Principal components (or factors) obtained by the PCA analysis (), average values calculated from the measurements recorded in four frequency ranges and measurements taken at the selected frequencies were used as the predictor variables in models denoted as A, B, and C for the prediction of WPS () and salt and moisture content (). shows that two principal components (PCs) were extracted from the entire impedance module spectra, and four PCs were extracted from the phase angle spectra (the ‘‘Kaiser criterion” was used to determine the number of PCs). PC1 obtained from the impedance module data accounted for 85.20% of the total variance and was loaded with the IZI measured at frequencies 5 kHz–10 MHz, whereas the PC2 accounted for 14.3% of the total variance and was loaded with the variables measured at frequencies higher than 11 MHz. The results indicate high correlations among the IZI measurements. Phase measurements were less correlated resulting in more (four) PCs extracted. For the multiple regression analysis, four PCs were used – both PCs obtained from the IZI spectra and first two PCs from the φ spectra in order not to violate the assumption regarding the number of predictor variables.
Table 2. Principal component analysis (PCA) of the impedance module (|Z|) and phase (φ) spectra measured at 150 frequencies (5 kHz–100 MHz) on trout samples subjected to wet salting up to 6 hours at 25% (w/w) brine (F1, F2, F3, and F4 are the symbols of the PCs used for the regression models, as the number of the predictors per 60 observations should maximally be four).
Table 3. Linear/multiple regression analysis for prediction of WPS (water phase salt) of the rainbow trout samples based upon their electrical properties.
Table 4. Linear/multiple regression analysis for prediction of moisture and salt of the rainbow trout samples based upon their electrical properties.
The prediction model A1 was statistically significant (4.55) = 90.436, p<0.001, and accounted for approximately 86% of the variance of WPS (R2 = 0,870; adjusted R2 = 0.867) (). With a small number of samples in this study, adjusted R2 (AdR2) is used to describe the models. F3 and F4 were statistically significant predictors, unlike F1 and F2. The Beta weights showed that F3 made a bit larger contribution to the prediction model than F4. The inspection of the coefficients suggests that, with the possible exception of F4 whose correlation with WPS is lower than 0.3, the predictor F3 contributes mostly to the model. The results indicate that IZI measurements recorded in the range 5 kHz–10 MHz contribute mostly to the model, with a weaker contribution of the IZI measurements recorded at frequencies higher than 11 MHz (). Therefore, models A2 and A3 were calculated to examine the possibility of using only impedance module measurements for predicting the WPS. The results show that WPS may be predicted only slightly less well by the model calculated with the IZI measurements from the entire spectra (5 kHz–100 MHz, AdR2 = 0.856). When we excluded higher MHz frequencies (higher than 10 MHz), AdR2 decreased to 0.804, which still makes a model well fit for our data.
The best of the B models is presented in . For this model, one predictor from each spectrum was used (average values of |Z|measured at frequencies 5–10 kHz and average values of φ measured at frequencies 10–30 MHz). The model was statistically significant with the AdR2 of 0.842, and again the impedance module was a much better predictor of the WPS than the φ.
It is clear from the results that IZI is a much better predictor of the WPS than φ. This is expected since the real component (IZI) measurements in an aqueous solution are related to the capability of movement of ions under the influence of an electrical field,[4] which is altered by the salting process. The phase, on the other hand, is more related to the integrity of the membranes and cells, and contributes less to the predictive models. However, φ improved the models. It was reported by Chevalier et al.[3]
In the C models, measurements of the impedance module (|Z|) and phase (φ) recorded at a single or two frequencies were used as the predictor variables for linear and multiple regression analyses. A device that would operate at one or few frequencies, ideally in a lower frequency range, could be portable, inexpensive and easy to use. The results with the measurements taken from the entire impedance spectra described above indicated that the prediction models may be calculated with the impedance variables measured at just (preferably lower) few frequencies. The impedance modules measured at 20, 50 and 500 kHz, 1 and 15 MHz were used as the predictive variables in linear regression models and the best results were obtained with the |Z|measured at 50 kHz (). The impedance module measured at 50 kHz predicted the WPS with the AdR2 of 0.864; however when the model was calculated with the impedance module measured at the additional frequency of 30 MHz, the AdR2 was slightly higher (0.866, results not presented). Models with φ as the predictor variable recorded at different single frequencies had, as expected, much lower predictive strength (AdR2 ≅ 0.3–0.6, results are not presented). Different combinations of |Z|and φ were also used as the predictor variables in the multiple regression analysis (measured at one or two frequencies), and the best model was with the |Z|measured at 10 MHz and φ at 30 MHz (Model C2, AdR2 = 0.878, ). However, as mentioned earlier, for the development of an industrial device, such high MHz frequencies have drawbacks regarding the cost as well as accuracy of the results because of the challenges with the parasitic impedance of the electrode and the cables.
The variables used in models C1 and C2 presented in were also used for the assessment of the moisture and salt content of the samples. The results are shown in . The models calculated from the impedance module measured with the needle-type multi-electrode array at a single frequency of 50 kHz and the models calculated from the impedance module and phase measured with the same electrode at 2 different frequencies in a MHz range, are statistically significant, and accounted for more than 85% of the variance of the salt and moisture. A slightly better prediction was with the models calculated with the impedance variables measured at 2 different frequencies in a MHz range. However, as AdR2 is not much improved when the models were calculated with the variables measured at these high frequencies, the proposed frequency of measurements for the industrial device design would be a single frequency of 50 kHz. The equation for the determination of WPS is 10.9 −0.106·|Z|50kHz.
Although with the cylindrical surface electrodes used by Chevalier et al.[3] the salt content and WPS were better predicted by the variables measured at 1 MHz (R2 = 0.926 – salt; R2 = 0.890 – WPS), the moisture content was not so well predicted (R2 = 0.757) and, as mentioned in the Introduction, the surface electrodes cannot measure the salt content very precisely due to differences in the salt content through the fish or meat tissue immediately after salting. Masot et al.[4] obtained R2 > 0.93 when the impedance data in the range 100 Hz–1 MHz were used for prediction of the salt content by a single coaxial needle electrode. However, by coaxial needle electrodes only contact impedance at one point of the sample can be measured. The fish or meat tissue is homogeneous in structure with anisotropic properties, and these may affect the results if the measurements are performed on a small surface. The needle-type multi-electrode array measures impedance module on a larger surface, giving more average results.
The impedance changes with the alternations of the chemical composition among the samples (water/lipid content), during the post-mortem period[9] or as a consequence of freezing.[10,17,18] The samples in this study were obtained from the fish market and their history was not strictly controlled. The fish were of similar size, but the exact date of catching was not confirmed. We assume that the fish were not in the same post-mortem condition which may have influenced the electric measurements and therefore, a slightly lower AdR2 of the models in this study was obtained, compared to other studies.[3,4] Therefore, the predictive models should be calculated with variables recorded under controlled conditions, i.e. for a batch of fish with similar chemical composition, degree of freshness and tissue structure. For farmed fish it is achievable, but for wild fish salting, this method would not be robust enough.
With regard to other fast methods that have been investigated for salt and moisture determination, a short-wavelength near infrared (SW-NIR) reflectance spectroscopy also showed potential in non-destructive determination of salt, moisture and WPS in smoked salmon samples.[1] The salt was slightly less well predicted and the moisture slightly better predicted than in this study. Uddin et al.[19] used the same method for prediction of water and proteins in surimi, and obtained excellent regression coefficients (higher than 0.98) The practical use of NIR spectroscopy may be however limited by the requirement for laborious calibration of the equipment.[20] Another technique with potential application in salt and moisture determination of food is computed tomography.[21,22] Although this method could be valuable in scientific research, its’ drawback is cost, which makes it not very practicable for industry purposes.
Conclusion
The impedance module measured at 50 kHz with the needle-type multi-electrode array showed a potential in determining the WPS, salt, and moisture of the salted farmed fish. The needle-type multi-electrode array could be used for fast and non destructive evaluating the levels of salt and moisture of solid heterogeneous and anisotropic food samples within homogeneous salt distribution. The measurements can be performed at only one frequency and, therefore, the portable device could be designed cost efficiently. However, as the measurements should be performed under controlled conditions, the presented model can be used for quality control of the salting process of fish of known origin while for other applications, model calibrations should be performed.
References
- Huang, Y.; Cavinato, A.G.; Mayes, D.M.; Bledsoe, G.E.; Rasco, B.A. Nondestructive Prediction of Moisture and Sodium Chloride in Cold Smoked Atlantic salmon (Salmo salar). Journal of Food Science 2002, 67(7), 2543–2547.
- Lin, M.; Cavinato, A.G.; Huang, Y.; Rasco, B.A. Predicting Sodium Chloride Content in Commercial King (Oncorhynchus tshawytscha) and Chum (O. keta) Hot Smoked Salmon Fillet Portions by Short-Wavelength Near-Infrared (SW-NIR) Spectroscopy. Food Research International 2003, 36(8), 761–766.
- Chevalier, D.; Ossart, F.; Ghommidh, C. Development of a Non-Destructive Salt and Moisture Measurement Method in Salmon (Salmo salar) Fillets Using Impedance Technology. Food Control 2006, 17, 342–347.
- Masot, R.; Alcañiz, M.; Fuentes, A.; Schmidt, F.C.; Barat, J.M.; Gil, L.; Baigts, D.; Martínez-Máñez, R.; Soto, J. Design of a Low-Cost Non-Destructive System for Punctual Measurements of Salt Levels in Food Products Using Impedance Spectroscopy. Sensors and Actuators A 2010, 158, 217–223.
- Kaltenecker, P.; Szöllősi, D.; Friedrich, L.; Vozáry, E. Determination of Salt Content in Various Depth of Pork Chop by Electrical Impedance Spectroscopy. Journal of Physics: Conference Series 2013, 434.
- Grimnes, S.; Martinsen, Ø. G. Bioimpedance and Bioelectricity Basics. Academic Press: San Diego, CA, 2000.
- Barsoukov, E.; Ross Macdonald J. Impedance Spectroscopy: Theory, Experiment, and Applications, 2nd Ed; John Wiley & Sons Inc.: New Jersey, 2005.
- Pething R. Electrical Properties of Biological Tissue in Modern Bioelectricity, Marcel Dekker: New York, 1988; 125–179.
- Zavadlav, S.; Janči, T.; Lacković, I.; Karlović, S.; Rogulj, I.; Vidaček, S. Assessment of Storage Shelf Life of European Squid (Cephalopod: Loliginidae, Loligo vulgaris) by Bioelectrical Impedance Measurements. Journal of Food Engineering 2016, 184, 44–52.
- Vidaček, S.; Janči, T.; Brdek, Z.; Udovičić, D.; Marušić, N.; Medić, H.: Petrak, T.; Lacković, I. Differencing Sea Bass (Dicentrarchus labrax) Fillets Frozen in Different Conditions by Impedance Measurements. International Journal of Food Science & Technology 2012, 47(8), 1757–1764.
- Duck, F.A. Physical Properties of Tissue: A Comprehensive Reference Book; Academic Press: London, United Kingdom, 1990; 173 pp.
- Ishai, P.B.; Talary, M.S.; Caduff, A.; Levy, E.; Feldman, Y. Electrode Polarization in Dielectric Measurements: A Review. Measurement Science and Technology 2013, 24(10), 1–21.
- Grimnes, S.; Martinsen, Ø.G. Sources of Error in Tetrapolar Impedance Measurements on Biomaterials and Other Ionic Conductors. Journal of Physics D Applied Physics 2007, 40, 9–14.
- Mazzeo, B.A.; Flewitt, A.J. Two- and Four-Electrode, Wide-Bandwidth, Dielectric Spectrometer for Conductive Liquids: Theory, Limitations, and Experiment. Journal of Applied Physics 2007, 102(10), 6–11.
- Hilderbrand, H.S. Quick determination of Water Phase Salt Content of Smoked Fish, Microwave Oven Procedure. Oregon State University: Extension Sea Grant, 2000.
- AOAC Official Methods of Analysis. Association of Official Analytical Chemists: Washington, DC, 1984.
- Vidaček, S.; Medić, H.; Botka-Petrak, K.; Nežak, J.; Petrak, T. Bioelectrical Impedance Analysis of Frozen Sea Bass (Dicentrarchus labrax). Journal of Food Engineering 2008, 88, 263–271.
- Vidaček, S.; Medić, H.; Marušić, N.; Tonković, S.; Petrak, T. Influence of Different Freezing Regimes on Bioelectrical Properties of Atlantic Chub Mackerel (Scomber colias). Journal of Food Process Engineering 2012, 35(5), 735–741.
- Uddin, M.; Okazaki, E.; Fukushima, H.; Turza, S.; Yumiko, Y.; Fukuda, Y. Nondestructive Determination of Water and Protein in Surimi by Near-Infrared Spectroscopy. Food Chemistry 2006, 96, 491–495.
- Sun, J. Infrared Spectroscopy for Food Quality Analysis and Control. Academic Press: New York, USA, 2009.
- Vestergaard, C.; Risum, J.; Adler-Nissen, J. Quantification of Salt Concentrations in Cured Pork by Computed Tomography. Meat Science 2004, 68, 107–113.
- Fulladosa, E.; Santos-Garcés, E.: Picouet, P.; Gou, P. Prediction of Salt and Water Content in Dry-Cured Hams by Computed Tomography. Journal of Food Engineering 2010, 96, 80–85.