ABSTRACT
Defatted walnut residues (DWR) were hydrolysed using Bacillus subtilis to produce angiotensin-I-converting enzyme (ACE) inhibitory peptides. Fermented walnut residue extract (FWRE) was fractionated using ultrafiltration methods to identify the ACE-inhibitory peptides. The fraction with a molecular weight of <1 kDa (WPs-I) presented the greatest ACE-inhibitory activity. Reverse-phase high-performance liquid chromatography was used to separate WPs-I from the most active fractions, and the amino acid sequence of WPs-I was confirmed as VQTL (459.351 Da) and LGYEN (594.276 Da) by UPLC-ESI-MS/MS.
Introduction
Hypertension or high blood pressure is increasing annually in populations worldwide. Approximately 13.5% of premature deaths worldwide are attributed to high blood pressure.[Citation1] Angiotensin-I-converting enzyme (ACE, EC 3.4.15.1) is a dipeptidyl carboxypeptidase associated with blood pressure regulation and cardiovascular function.[Citation2] Consequently, ACE-inhibitory substances are used to decrease the blood pressure of hypertensive patients.[Citation3] Potent synthetic ACE inhibitors, such as perindopril, captopril, enalapril, alacepril, lisinopril, and ramipril, are widely used to clinically treat hypertension in humans. However, these synthetic drugs exert several side effects, including cough, skin rashes, hypotension, hyperkalemia, headache, dizziness, and renal impairment.[Citation4,Citation5] Food-derived ACE inhibitors are safer than synthetic compounds.[Citation6] To date, many ACE-inhibitory peptides have been isolated from food proteins, such as egg yolk protein, Agaricus bisporus, whey protein, and gluten.[Citation7–Citation10] Some of these peptides have been found effective as antihypertensive agents in vivo without causing side effects in spontaneously hypertensive rats (SHRs) and hypertensive humans.[Citation11]
Walnut (Juglans regia L.) is the most widespread and oldest tree nut in the world.[Citation12] Walnut is a nutrient-dense food, mainly owing to its fat content, as well as protein, vitamin, and mineral profiles. Several biological properties of walnut such as antiatherogenic, anti-inflammatory, antimutagenic, and antioxidant activities have been reported.[Citation13] Studies have shown that bioactive peptides demonstrate functions better than those of other proteins, as a result of the enzymatic hydrolysis of nut proteins.[Citation14] However, the biological activity of fermented walnut products has not been investigated. Therefore, the current study aims to isolate and characterize potential ACE-inhibitory peptides from fermented walnut residue extract (FWRE).
Materials and methods
Materials
Defatted walnut residues (DWR), processed by Yunnan Huizhiyuan Food Company (Lincang City, China) in 2012, were ground to powder by a muller (mesh concentration 60 mm) with a protein content of 43.5%. Angiotensin converting enzyme (ACE) and hippuryl-L-histidyl-L-leucine (HHL) were purchased from Sigma-Aldrich Co. (St. Louis, MO, USA). Other chemicals (analytical grade) were obtained from Beijing Chemical Co., Ltd. (Kunming, China). B. subtilis GIM 1.135 was purchased from the Microbial Culture Collection Center of Guangdong (GIMCC), China.
Preparation of FWRE
Bacillus subtilis was stored at 4°C on agar slants and cultured for 16 h at 37°C prior to use for solid-state fermentation (SSF). After incubation for 16 h, part of the nutrient broth culture was used as an inoculum. Serial dilution by using sterile distilled water with glucose (0.2 g/100 mL) and KH2PO4 (0.26 g/100 mL) was performed to adjust the bacterial concentration to 108 CFU/mL. SSF was conducted in 500 mL Erlenmeyer flasks containing 100 g of DWRs, followed by autoclaving at 121°C for 20 min, cooling at room temperature, and use for inoculation. The fermentation conditions included a fermentation time of 82 h, temperature of 37°C, inoculum concentration of 10.40%, and water content of 1.50 mL/g.[Citation15]
FWRE was mixed with distilled water (1:25, g/mL) by shaking on a rotary shaker (180 rpm, 30 min, and 37°C) to prepare walnut peptides (WPs). The mixture was centrifuged (Allegra X-30R; Beckman Coulter, Brea, CA, Inc., USA) at 10,000 ×g for 10 min (4°C), and supernatants were lyophilized by a freeze dryer (LGJ-10F; Beijing Huaxing Technology Development Co., Ltd., Beijing, China).
ACE-inhibitory activity assay
ACE-inhibitory activity was measured by a spectrophotometric method[Citation16] with minor modifications. The reaction mixture contained 80 µL of 5 mmol/L HHL as a substrate, 10 µL of ACE powder (0.1 unit) in 100 mmol/L sodium borate buffer (pH 8.3), and 10 µL of the sample solution. The reaction was performed at 37°C for 30 min and terminated by adding 200 µL of 1 mol/L HCl and 1.2 mL of ethyl acetate (−20°C). After centrifugation, 1 mL of the supernatant was transferred into a test tube and evaporated at room temperature for 2 h in a vacuum. Hippuric acid was redissolved in 1 mL of distilled water, and the absorbance was measured at 228 nm. IC50 was defined as the concentration of the peptide inhibiting 50% of the ACE activity. IC50 was determined from the ACE-inhibitory activity and peptide contents of each sample after regression analysis.
Isolation of ACE-inhibitory peptides
Ultrafiltration
WPs were fractionated by ultrafiltration (UF) with 1000, 5000, and 10,000 molecular weight cut-off UF membranes (Amicon Co., Beverly, MA, USA). Four fractions with molecular weights of <1, 1–5, 5–10 kDa, and >10 kDa were obtained and lyophilized. The fractions were pooled, concentrated, and lyophilized for ACE-inhibitory activity assay.
Yield and content of the peptides isolated by ultrafiltration
The peptide content was determined in accordance with the national standard of China (GB/T 5009.124-2003). Walnut protein hydrolysate (1 g) was dispersed into 50 mL of trichloroacetic acid solution (15% TCA, w/w) and stirred magnetically for 30 min. The solution was allowed to stand for 5 min following filtration using a filter paper. The contents of the filtrated protein and free amino acid were determined by the Kjeldahl method and an automatic amino acid analyser (Hitachi L-8900, Tokyo, Japan), respectively.[Citation17] The peptide content and the yield of peptide were then calculated according to Eqs. (1) and (2).
ACE-inhibition pattern determination of the purified peptides
The fraction that exhibited the greatest ACE-inhibitory activity after ultrafiltration and purification was determined (ACE-inhibition pattern). The inhibition pattern was determined by spectrophotometry.[Citation16] ACE kinetics in the presence of the inhibitor were analysed by Lineweaver–Burk plots[Citation17] at three peptide concentrations (0, 0.2, and 0.4 mg/mL), in which the reciprocal of HHL concentration was used as an independent variable (X-axis), and the reciprocal of the HA production rate was used as a dependent variable (Y-axis). The Lineweaver–Burk plot, showing correlations between 1/V and 1/[S], was used to determine the X-axis intercepts of −1/Km (without inhibitor) and −1/{Km(1 + [I]/Ki)} (with inhibitor), which were used to calculate the inhibitory constant K i.[Citation18] All the experiments were conducted in triplicate.
Purification and identification of ACE inhibitory peptide amino acid sequences (UPLC-ESI-MS/MS)
The fraction that exhibited the greatest ACE-inhibitory activity after ultrafiltration and purification was dissolved in distilled water and loaded into a Waters Acquity Ultra Performance Liquid Chromatography (UPLC) system with a reversed-phase column (Zorbax StableBond SB C18, 5 µm, 4.6 mm × 250 mm, Agilent, USA). The flow rate was set at 0.3 mL/min, and the column temperature was 30°C. Mobile phase B was 100% acetonitrile. For the analysis of samples, the solvent gradient used was 0.1% formic acid in water. The mobile phases were 5–70% B (0–20 min) and 70% B (20–25 min).[Citation19]
Mass spectrometry was performed on a Waters Xevo TQ-S (Waters, USA) with an electrospray ionization (ESI) source. Ionization was conducted in positive mode with a capillary voltage of 3500 V. Nitrogen was maintained at 40 psi for nebulization and 9 L/min at 350°C for evaporation temperature. Spectra were recorded over the mass/charge (m/z) range of 100–1000. Data acquisition and processing were performed using MassLynx 4.1. Peptide sequences were manually calculated using a 477A gas-phase automated sequencer (Applied Biosystems, Inc., Foster, CA USA).
Statistical analysis
All experiments were performed in at least three independent trials, and the results were reported as means ± standard deviations. Results were subjected to analysis of variance using SPSS, and the statistical significance of differences (p < 0.05) was evaluated.
Results and discussion
Isolation of ACE inhibitory peptides and its ACE inhibitory properties
The hydrolysate obtained by the digestion of walnut protein with B. subtilis showed higher ACE-inhibitory activity than that demonstrated by untreated walnut protein (). This finding indicated that the hydrolysis of walnut protein probably released a significant number of ACE-inhibitory peptides. The walnut protein hydrolysate was fractionated into four tiers by ultrafiltration. The peptide yield, peptide content, and ACE-inhibitory activities are listed in . WPs-I exhibited the highest ACE-inhibitory activity (IC50 value of 0.098 ± 0.005 mg/mg) and peptide yield (62.20 ± 2.65%), whereas WPs-IV showed the lowest activity (IC50 value of 0.982 ± 0.086 mg/mg) and peptide yield (1.20 ± 0.32%). WPs-II presented the highest peptide content, but it displayed a lower ACE-inhibitory activity (IC50 value of 0.264 ± 0.027 mg/mg) than that of WPs-I. The molecular weight of walnut protein ranged from 12000 Da to 70000 Da,[Citation20] whereas most fractions of the WPs were smaller than 10 kDa. This result confirmed that B. subtilis digested the walnut protein into small peptide fragments. The peptide yield of WPs-I was higher than that of the three other fractions. The ACE-inhibitory activity of WPs-I was significantly higher than that of the enzymatic hydrolysis products of walnut protein (0.97–1.52 mg/mL).[Citation21] Pihlanto-Leppälä et al.[Citation22] found that the ACE-inhibitory activity of <1 kDa fraction in whey peptides was higher than that of the other tested fractions. Both results suggest the use of ultrafiltration to enrich the ACE-inhibitory peptides derived from walnut protein.
Table 1. Peptide yields, peptide contents, and IC50 values of fractions isolated by ultrafiltration.
The inhibition pattern of the purified peptides against ACE
Lineweaver–Burk plots were constructed using three concentrations of WPs-I (0, 0.2, and 0.4 mg/mL), as shown in . The X-axis intercepts for the different concentrations of WPs-I are identical, indicating that WPs-I showed non-competitive inhibition. Accordingly, Ki was calculated to be 1.23 ± 0.15 mg/mL for this fraction. The Lineweaver–Burk plots indicated that WPs-I appeared non-competitive with HHL for the binding sites of ACE.
Purification and identification of ACE inhibitory peptide amino acid sequences by LC-ESI-MS/MS analysis
WPs-I was subjected to RP-HPLC coupled online to an ion trap mass spectrometry to identify active peptides. shows the RP-HPLC-UV chromatographic profile corresponding to the analyses of Fra.I and Fra.II. The resulting chromatograms contained two major peaks (retaining time = 11.78 min, 16.64 min), which are the target peptides.
Figure 2. Purification of the peptides from the WPs-I fraction obtained from ultrafiltration using reversed-phase high-performance liquid chromatography on Zorbax SB-C18 column with a linear gradient of acetonitrile containing 0.1% formic acid (TFA) at a flow rate of 0.3 mL/min and column temperature 30°C.
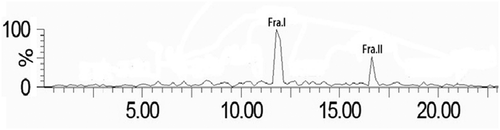
Fraction WPs-I with relatively high ACE-inhibitory activity as previously indicated was subjected to individual peptide separation and sequence identification. Full-scan UPLC/MS data were mined post-acquisition for the calculated m/z values of the different multiple-charged Fra.I and Fra.II forms. These data were analysed by ESI-MS for molecular mass determination and then examined by ESI-MS/MS for the characterization of peptides. The MS spectrum of Fra.I is shown in , and the MS/MS spectrum of a single charged ion with m/z at 459.351 Da is displayed in . The MS spectrum of Fra.II is shown in , and the MS/MS spectrum of a single charged ion with m/z at 594.276 Da is shown in . Peptides were manually calculated. The results provided two deduced amino acid sequences: Val-Gln-Thr-Leu (VQTL, 459.351 Da) and Leu-Gly-Tyr-Glu-Asn (LGYEN, 594.276 Da). The results further confirmed the general finding, indicating that short peptides with 2–10 amino acids exhibit greater ACE-inhibitory activity,[Citation23] and bioactive peptides usually contain less than 20 amino acid residues per molecule.[Citation22] Moreover, peptides with lower molecular weights present a better chance of crossing the intestinal barrier and exerting biological effects.[Citation24] Majority of the related studies suggested that most ACE-inhibitory peptides derived from food sources are approximately 150–800 Da in terms of molecular weight.[Citation25] These findings agree with observations from other studies, thereby supporting the fact that the functional properties of ACE-inhibitory peptides are highly influenced by molecular mass. This report implies that active peptides can be selected to a degree by ultrafiltration, resulting in the production of a permeate stream with higher ACE inhibition than that of the original hydrolysate.[Citation26] Given the low molecular mass coupled with low allergenicity,[Citation27] the potential of the hydrolysate for use in functional foods to mitigate hypertension would seem to merit further clinical studies in humans. Synthetic drugs will always demonstrate greater activity than natural products such as hydrolysates or peptide fractions. However, hydrolysates not only exhibit biological activity but also improve the nutritional contribution.[Citation28] Peptide fractions are constituted by sets of peptides with similar molecular weights, which may synergistically act to increase their effects on an organism.[Citation29]
Figure 3. (A) The MS spectrum of Fra.I collected after RP-HPLC. (B) The MS/MS spectrum of a single charged ion (459.351 Da).
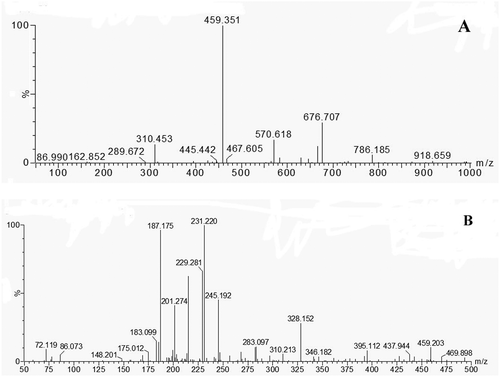
Figure 4. (A) The MS spectrum of Fra.II collected after RP-HPLC. (B) The MS/MS spectrum of a single charged ion (594.276 Da).
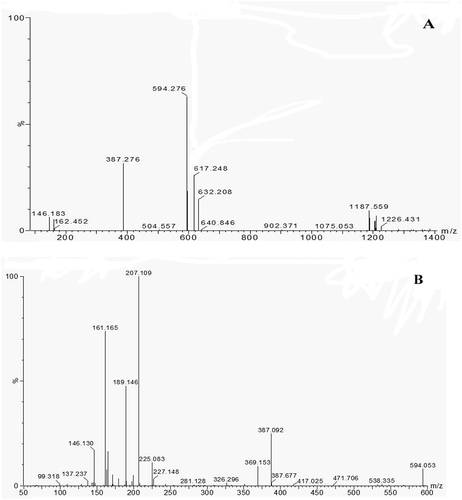
In addition to peptide size, different amino acid residues and peptide sequences may play an important role in determining ACE-inhibitory activities. Some studies suggested that most naturally occurring ACE-inhibitory peptides contain Pro, Lys, or aromatic amino acid residues.[Citation30–Citation32] Peptides bound to ACE are strongly influenced by the C-terminal sequence, which would be more active with a hydrophobic amino acid at the C-terminal.[Citation33] In the current study, the C-terminal of VQTL is leucine, which is a hydrophobic amino acid. However, further research on the relationship between the structure and function of ACE-inhibitory peptides is needed because establishing the relationship between their structure and activity is a valuable research goal to further understand the underlying mechanisms.
Conclusion
In this study, fermented walnut extract (FWE) was proven to demonstrate extraordinary ACE-inhibitory activity. Two ACE-inhibitory peptides (VQTL and LGYEN) were identified by UPLC-ESI-MS/MS from the fraction (WPs-I) isolated from FWRE by ultrafiltration and RP-HPLC. Therefore, Fra.I and Fra.II are potentially suitable for extraction from related foods as natural ACE inhibitors for preventing hypertension. Moreover, the structural analysis laid a solid foundation for the artificial synthesis of ACE-inhibitory peptides. FWE or its isolated peptides can serve as a promising ACE-inhibitor source for functional food ingredients and pharmaceuticals.
Funding
The authors would like to acknowledge the financial support of the National Natural Science Foundation of China (No. 51376200).
Additional information
Funding
References
- Lawes, C.M.; Hoom, S.V.; Rodgers, A. Global Burden of Blood-Pressure Related Disease, 2001. The Lancet 2008, 371, 1513–1518.
- Wang, H.; Meng, F.; Yin, L.; et al. Changes of Composition and Angiotensin I-Converting Enzyme (ACE)-inhibitory activity during Douchi fermentation. International Journal of Food Properties 2016, 19(11), 2408–2416.
- Li, F.; Liu, W.; Yamaki, K.; et al. Angiotensin I-Converting Enzyme Inhibitory Effect of Chinese Soypaste along Fermentation and Ripening: Contribution of Early Soybean Protein Borne Peptides and Late Maillard Reaction Products. International Journal of Food Properties 2016, 19(12), 2805–2816.
- Brugts, J.J.; Hisatomi, A.; Remme, W.; Brugts, J.J.; et al. The Incidence and Clinical Predictors of ACE-inhibitor Induced Dry Cough by Perindopril in 27,492 Patients with Vascular Disease. International Journal of Cardiology 2014, http://dx.doi.org/10.1016/j.ijcard. 2014.07.108.
- Barbosa-Filho, J.M.; Martins, V.K.M.; Rabelo, L.A.; Moura, M.D.; Silva, M.S.; Cunha, E.V.L.; et al. Natural Products Inhibitors of the Angiotensin Converting Enzyme (ACE). A review between 1980-2000. Revista Brasileira De Farmacognosia-brazilian Journal of Pharmacognosy 2006, 16, 421–446.
- Atkinson, A.B.; Robertson, J.I.S. Captopril in the Treatment of Clinical Hypertension and Cardiac Failure. The Lancet 1979, 2, 836–839.
- Ewelina, E.; Aleksandra, Z.; Marta, P.; Bartosz, S. Egg-yolk Protein By-product as a Source of ACE-inhibitory Peptides Obtained with using Unconventional Proteinase from Asian Pumpkin (Cucurbita fi cifolia). Journal of Proteomics 2014, 110, 107–116.
- Ching, C.L.; Noorlidah, A.; Adawiyah, S.S.; Norhaniza, A. Novel Angiotensin I-converting Enzyme Inhibitory Peptides Derived from Edible Mushroom Agaricus bisporus (J.E. Lange) Imbach Identified by LC-MS/MS. Food Chemistry 2014, 148, 396–401.
- Wang, L.; Jiang, Z.; Tian, B.; et al. Effects of Galactose Concentration on Characteristics of Angiotensin-I-converting Enzyme Inhibitory Peptides Derived from Bovine Casein in Maillard Reaction. International Journal of Food Properties 2016, 19(10), 2238–2250.
- Ahmad, A.; Leyla, H.; Jamashidkhan, C.; Mohamad, A.A.; Zahra, M.; Milad, L. Potential Angiotensin I-converting Enzyme Inhibitory Peptides from Gluten Hydrolysate: Biochemical Characterization and Molecular Docking Study. Journal of Cereal Science 2014, 60, 92–98.
- Li, G.H.; Le, G.W.; Shi, Y.H.; Shrestha, S. Angiotensin I-converting Enzyme Inhibitory Peptides Derived from Food Proteins and their Physiological and Pharmacological Effects. Nutrition Research 2004, 27, 469–486.
- Yi, J.; Sun, Y.; Zhu, Z.; et al. Near-infrared Spectroscopy (NIRS) for the Prediction of Chemical Composition in Walnut Kernel. International Journal of Food Properties, 2016, 20(7), 1633–1642.
- Martínez, M.L.; Labuckas, D.O.; Lamarque, A.L.; Maestri, D.M. Walnut (Juglans regia L.): Genetic Resources, Chemistry, By-Products. Journal of The Science of Food And Agriculture 2010, 90, 1959–1967.
- Jamdar, S.N.; Rajalakshmi, V. Influence of Degree of Hydrolysis on Functional Properties, Antioxidant Activity and ACE Inhibitory Activity of Peanut Protein Hydrolysate. Food Chemistry 2010, 121, 178–184.
- Wu, W.; Zhao, S.; Chen. C.; et al. Optimization of Production Conditions for Antioxidant Peptides from Walnut Protein Meal using Solid-state fermentation. Food Science and Biotechnology 2014, 23(6):1941–1949.
- Cushman, D.W.; Cheung, H.S. Spectrophotmetric Assay and Properties of the Angiotensin-converting Enzyme of Rabbit Lung. Biochemical Pharmacology 1971, 20, 1637–1648.
- Chen, N.; Yang, H.G.; Sun, Y.; Niu, J.; Liu, S.Y. Purification and Identification of Antioxidant Peptides from Walnut (Juglans regia L.) Protein Hydrolysates. Peptides 2012, 38, 344–349.
- Fennema, O.R.; Whitaker, J.R. Food chemistry. CRC Press Taylor & Francis Group, 1996; 480–481 pp.
- Chen, J.W.; Liu, S.S.; Ye, R.; Cai, G.G.; Ji, B.; Wu, Y.G. Angiotensin-I Converting Enzyme (ACE) Inhibitory Tripeptides from Rice Protein Hydrolysate: Purification and Characterization. Journal of Functional Foods 2013, 5, 1684–1692.
- Sze-Tao, K.W.C.; Sathe, S.K. Walnuts (Juglans regia L): Proximate Composition, Protein Solubility, Protein Amino Acid Composition and Protein in Vitro Digestibility. Journal of the Science of Food and Agriculture 2000, 80(9), 1393–1401.
- Xu, H.J.; Hao, Y.B.; Qi, J.X.; Fan, G.S.; Wang, K.J. Study on Antioxidant Activity and AntihypeIrtensive Activity of Enzymatic Hydrolysis Products of Walnut Protein. Innovational Edition of Farm Products Processing 2009, 10, 38–42.
- Pihlanto-Leppälä, A.; Koskinen, P.; Piilola, K.; Tupasela, T.; Korhonen, H. Angiotensin I-converting Enzyme Inhibitory Properties of Whey Protein Digest: Concentration and Characterization of Active Peptides. Journal of Dairy Research 2000, 67, 53–64.
- Megias, C.; Yust, M.D.; Pedrohe, J.; Lquari, H.; Giron-Calle, J.; Alaiz, M. Purification of an ACE Inhibitory Peptide after Hydrolysis of Sunflower (Helianthus annuus L.) Protein Isolates. Journal of Agriculture and Food Chemistry 2004, 52, 1928–1932.
- Sampath, K.N.S.; Nazeer, R.A.; Jaiganesh, R. Purification and Identification of Antioxidant Peptides from the Skin Protein Hydrolysate of Two Marine Fishes, Horse Mackerel (Magalaspis cordyla) and Croaker (Otolithesruber). Amino Acids 2012, 42, 1641–1649.
- Zhang, F.; Wang, Z.; Xu, S. Macroporous Resin Purification of Grass Carp Fish (Ctenopharyngodon idella) Scale Peptides with in Vitro Angiotensin-I Converting Enzyme (ACE) Inhibitory Ability. Food Chemistry 2009, 117, 387–392.
- Sun, J.; He, H.; Xie, B.J. Novel Antioxidant Peptides from Fermented Mushroom Ganoderma Lucidum. Journal of Agricultural And Food Chemistry 2004, 52, 6646–6652.
- Pan, D.; Cao, J.; Guo, H.; et al. Studies on Purification and the Molecular Mechanism of a Novel ACE Inhibitory Peptide from Whey Protein Hydrolysate. Food Chemistry 2012, 130(1): 121–126.
- Guan, H.L.; Ming, R.Q.; Ju, Z.W.; Jin, M.Y. Antihypertensive Effect of Rice Protein Hydrolysate with in Vitro Angiotensin I-converting Enzyme Inhibitory Activity in Spontaneously Hypertensive Rats. Asia Pacific Journal of Clinical Nutrition 2007, 16(1), 275–280.
- Fujita, H.; Yoshikawa, M. LKPNM: A Prodrug-Type ACE-inhibitory Peptide Derivedfrom Fish Protein. Immunopharmacology 1999, 44(1–2), 123–127.
- Cheung, H.S.; Wang, F.L.; Ondetti, M.A.; Sabo, E.F.; Cushman, D.W. Binding of Peptide Substrates and Inhibitors of Angiotensin-Converting Enzyme. The Journal of Biological Chemistry 1980, 255(2), 401–407.
- Kawakami, A.; Kayahara, H. Synthesis of Leu-Lys-Tyr Derivatives and their Interaction with Angiotensin Converting Enzyme. Journal of the Japanese Society of Nutrition and Food Science 1993, 46(5), 425–428.
- Suetsuna, K.; Nakano, T. Identification of an Antihypertensive Peptide from Peptic Digest of Wakame (Undaria pinnatifida). The Journal of Nutritional Biochemistry 2000, 11(9), 450–454.
- Rohrbach, M.S.; Williams, E.B. Jr.; Rolstad, R.A. Purification and Substrate Specificity of Bovine Angiotensin-Converting Enzyme. Journal of Biological Chemistry 1981, 256(1), 225–230.