ABSTRACT
This paper dealt with the physicochemical, functional and antioxidative properties of Yak skin gelatins with various molecular weights. The gelatin was extracted by alkaline process, and different molecular weight gelatin was achieved by ultrafiltration with different molecular weight cut membrane. The SDS-PAGE showed the molecular weight of the yak skin gelatin. The UV-Vis absorption turned out that the high molecular weight (HMW) of yak skin gelatin had a higher maximum absorption peaks. The FT-IR indicated the change of gelatin structures with the change of the molecular weight. No matter the HMW or the low molecular weight (LMW), the Yak skin gelatin had a high denaturation temperature (Td), and it was higher than most of the other mammals and marine biological gelatin. The HMW gelatin had higher imino acids contents and lower oxidation resistance, foamability and emulsibility compared to the LMW gelatin. These findings showed that a certain molecular weight range of Yak skin gelatin has great potential for application as an alternative to commercial gelatin due to its good antioxidative, foamability emulsibility and thermotolerance.
Introduction
Gelatin is a poly-ampholytic biopolymer which has been widely used in the food industry, pharmaceuticals, photography and other technical applications.[Citation1] Recently, the outbreaks of bovine spongiform encephalopathy (BSE) and foot-and-mouth disease (FMD) have created a demand for unpolluted resource of gelatin for food and medicine applications.[Citation2] Therefore, people are eager to find safer sources of collagen including marine animals (fish, jellyfish, marine sponge and squid) and those unpolluted animals.[Citation3–Citation5] The Yak (bos grunniens), as a year-round grazing animal, is one kind of the unique livestock in the world, mainly living in the Qinghai-Tibetan Plateau. It was famous with its high altitude, unpolluted natural environment and frequent seasonal variation.[Citation6,Citation7] That is why Yak is regarded as the unpolluted animal, its meat is considered as green food, and its skin is believed as a new source of collagen. Zhang et al.[Citation8] proved Yak skin glue contains 18 kinds, about 75.41% of amino acids (including seven kinds of essential amino acids), and two kinds of trace elements. Besides these, there was little study about the physicochemical and functional properties of gelatin polypeptide from Yak skin.
The quality of the gelatin preparation depends on its physicochemical properties, which are influenced not only by the severity of the pretreatment and extraction process, but also by the molecular weight and amino acid composition. Amino acid composition depends on the source of the gelatin, while the molecular weight mainly depends on extraction process. It is well known that molecular weight of macromolecule has a close relationship with its physical and functional properties. According to Johnston-Banks,[Citation9] the sum of intact α and β fractions together with their peptides is proportional to the gel strength, while the viscosity, setting rate and melting point are increased with the amount of the HMW (greater than g) fraction increase. According to Tavernier,[Citation10] high incidence of LMW peptides is associated with low viscosity, melting point, setting point and high setting time. The Ag+ in photographic gelatin is only combined with the α protein component rather than the γ or the β components.[Citation11] A component (peptides <5 kDa) that is hydrolyzed by alcalase protease from fish scales gelatin has a strongest inhibitory effect of ACE-inhibitory activity, which plays an important physiological role in regulating blood pressure in the human body.[Citation12] Therefore, the molecular weight of gelatin is an important physical property index.
The scope of the present paper was to obtain a series of different molecular weight of Yak skin gelatin by alkaline process, then to study their physicochemical, function and antioxidative properties, including the yield, molecular weight distirbution, UV-Vis absorption spectra, fourier transform infrared spectroscopy (FT-IR), denaturation temperature (TD), amino acid composition, foamability, emulsibility and oxidation resistance. All these were studied to give the foundation of future food and pharmacology function of Yak skin gelatin.
Materials and methods
Raw materials
Fresh Yak skin was supplied by BaiDe Foods Factory (QingHai, China). Pepsin had a declared activity of 3000–3500 units/mg (Pangbo Biotech. Company, Guangxi, China). Molecular weight standard (17–180 kDa) was obtained from Sigma-Aldrich, Inc. (Dublin, Ireland). All reagents used were of analytical grade.
Pretreatment
The whole clean Yak skin was divided into a size of 30 × 30 cm block and immersed in the 2.0% NaOH water solution for 48 h to depilation. Then, the depilated skin was soaked with water for many times until the soaking water changed into neutral.
Extraction of Yak skin gelatin
The Yak skin after pretreatment was heated in distilled water with a skin/solution ratio of 1:10 (w/v) for 6 h at 80°C with continuous stirring to extract the gelatin from the skin. After the first heat, the solution was filtered with four layers of gauze. Then, the filter residue was heated in distilled water with a residue/solution ratio of 1:10 (w/v) for 6 h at 80°C again. The solution was filtered with four layers of gauze again. The mixtures of first and second filtrate were the required gelatin solution. Then, the ultrafiltration membranes with different molecular weight cut (0.1 μm, 150 kDa and 10 kDa) were used to obtain a series of different molecular weight of Yak skin gelatin. Six samples after ultrafiltration were obtained, and then, the gelatin solution were freeze-dried and stored at 4°C for further use. The powder obtained, designated as A1, A2, A3, A4, A5, A6, respectively. The molecular weight of each sample was A1 > 0.1 μm, A2 < 0.1 μm, 0.1 μm > A3 > 150 kDa, A4 < 150 kDa, 150 kDa > A5 > 10 kDa, A6 < 10 kDa.
Physicochemical properties of yak skin gelatin
Yield of gelatin
The yield of gelatin was calculated based on the wet weight of fresh skin as follows:
Electrophoretic analysis
SDS-PAGE pattern of gelatin was determined according to the method described by Laemmli[Citation13] using 6% resolving gel and 5% stacking gel. After electrophoresis, the gel was stained with 1 g/l Coomassie brilliant blue R-250, dissolved in water, methanol and trichloroacetic acid (5:4:1), and de-stained using a solution containing methanol, distilled water, and acetic acid at a ratio of 5:4:1. Type I collagen of calf skin (Sigma, St. Louis, MO, USA) and the standard protein molecular weight markers (Sigma, St. Louis, MO, USA) were used as marker for α-chain and β-component mobilities and obtained the molecular weight bands of protein.
UV-Vis absorption spectra
The UV spectra of Yak skin gelatin were determined according to the method described by Lee and Kim[Citation14] and used an UV-Vis spectrophotometer (Varian Cary 300, America). Samples were dissolved in distilled water, respectively, and every sample was 1 mg/ml. The solution samples were tested separately, and distilled water was the reference. The range of the absorbance curve was from 190 to 400 nm, and the resolution was 0.5 nm.
Determination of denaturation temperature
DSC was employed to determine the thermal properties of the gelatin. A power compensation DSC (Mettler Toledo DSC2 STARe System), equipped with a mechanical cooling accessory (Julabo FT 100), was used for thermal analysis. Temperature calibration was done using n-decane and indium (melting points at −29.66 and 156.60°C, respectively). Heat flow calibration was done using indium (heat of fusion, 28.45 J/g). All experiments were performed under nitrogen with a flow rate of 50 ml/min. Samples of 5–7 mg (±0.0005 mg) were weighed by a Mettler Toledo MS105 Ultra-microbalance and placed into aluminum pans (40 μl) without small piercings, and an empty pan was used as a reference. A heating rate of 10 K/min and the temperature range of 25–250°C were employed for the measurements.
FT-IR spectroscopy
The samples were subjected to attenuated total reflectance-Fourier transform infrared spectroscopy (ATR-FTIR). Fourier Transform Infrared Spectra were recorded using a ThermoFisher Nicolet FT-IR spectrometer. A resolution of 4 cm−Citation1 and the average of 64 automated scans from 400 to 4000 cm−Citation1 were used to obtain the FT-IR spectra.
Amino acid composition
The amino acid of gelatin was determined according to the method described by Bildlingmeyer et al.[Citation15] Briefly, dry gelatin (10–20 mg) was mixed with 6 mol/l HCl (1 ml) containing 1% phenol (v/v). The mixture was evacuated, blown with Ar, and sealed before hydrolysis at 110°C for 24 h, and then, the samples were cooled and made up to 5 ml with deionized water. A portion (25 ml) was then dried and derivatized. Derivatization involved addition of 10 ml of a mixture of methanol, water and trimethylamine (2:2:1) was mixed and dried for 5 min, which was followed by 20 ml mixture of methanol, water, trimethylamine and phenylisothiocyanate (7:1:1:1). The sample was held for 20 min at room temperature (20–25°C), dried under vacuum and then dissolved in 200 ml of pH 7.4 phosphate buffer and filtered with a 0.45 mm filter. Portions (7 μl) of the filtered samples were injected using an automatic loader G1329B (Agilent, USA) into the C18 column (Shiseido Capcell Pak C18, 4.6 mm × 250 mm, 5 µm), for amino acid analysis.
Functional properties of Yak skin gelatin
Emulsifying properties
Emulsion activity index (EAI) and emulsion stability index (ESI) of gelatin samples were determined according to the method of Zarai el at.[Citation16] with a slight modification. Peanut oil (2 ml) and gelatin solution (3%, w/v, 6 ml) were homogenized (D-106, Dragon Laboratory Instruments Limited, Beijing, China) at a speed of 20,000 rpm for 1 min. Emulsion was pipetted out at 0 and 10 min and 100-fold diluted with 0.1% SDS. The mixture was mixed thoroughly for 10 s using a vortex mixer (VORTEX-5, Qilinbeier Instruments Limited, Beijing, China). A500 of the resulting dispersion was measured using a spectrophotometer (Varian Cary 300, America). EAI and ESI were calculated as follows:
where A is A500, DF is dilution factor (100), l is path length of cuvette (m), ø is oil volume fraction, and C is protein concentration in aqueous phase (g/ml). A0 and A10 are absorbance at 500 nm measured at time 0 and 10 min after emulsification, ΔA is A0-A10, and Δt is 10 min.
Foaming properties
Foam expansion (FE) and foam stability (FS) of gelatin solutions were determined as described by Shahidi et al.[Citation17] with a slight modification. Gelatin solution (3%, w/v) was transferred into 100 ml cylinders and homogenized using a homogenizer (D-106, Dragon Laboratory Instruments Limited, Beijing, China) at 13,400 rpm for 1 min at room temperature. The sample was held for 0, 30 and 60 min. FE and FS were then calculated using the following equations.
where VT is total volume after whipping, V0 is the original volume before whipping, and Vt is total volume after leaving at room temperature for different times (30 and 60 min).
Antioxidant properties of Yak skin gelatin
DPPH radical–scavenging assay
DPPH radical–scavenging activity was determined as described by Bersuder et al.[Citation18] A volume of 500 μl 3 mg/ml of each sample was mixed with 500 μl of 99.5% ethanol and 125 μl of 0.02% DPPH in 99.5% ethanol. The mixtures were shaken and incubated in the dark for 60 min at room temperature. The reduction of DPPH radical was measured at 517 nm using a multimode plate reader (Perkin-Elmer, Inc., Waltham MA, U.S.). The DPPH radical–scavenging activity was calculated as follows:
DPPH radical−scavenging activity (%) = (1−A517 of sample/A517 of control)×100
The control was conducted in the same manner, except that distilled water was used instead of sample. A lower absorbance of the reaction mixture indicated a higher DPPH radical–scavenging activity.
FRAP assay
The ferric reducing ability of plasma (FRAP) method described by Benzie and Strain[Citation19] was used to measure the ferric ion reducing capacity of the Yak skin gelatin. It is based on the increase in absorbance at 595 nm due to the formation of the complex tripiridiltriazine (TPTZ)–Fe (II) in the presence of reducing agents at 37°C. Both gelatin samples were dissolved in distilled water. Absorbance was read at 30 min using a multimode plate reader (Perkin-Elmer, Inc., Waltham MA, U.S.). A standard curve of FeSO4 · 7H2O, which relates the concentration of FeSO4 · 7H2O (lM) to the absorbance at 595 nm, was performed. Results were expressed as mmol FeSO4 · 7H2O equivalents/g sample. At least three replicates were carried out for each sample.
ABTS assay
The ABTS radical (2, 20ʹ-azinobis-(3-ethylbenzothiazoline-6-sulfonic acid)) scavenging capacity of the Yak skin gelatin was determined according to a modified version of the method of Re et al.[Citation20] The stock solution of ABTS radical consisted of 7 mM ABTS in potassium persulfato 2.45 mM, kept in the dark at room temperature for 16 h. An aliquot of stock solution was diluted with distilled water in order to prepare the working solution of ABTS radical with absorbance at 734 nm of 0.70 ± 0.02. Both gelatin samples were dissolved in distilled water. A 20 μl aliquot of sample (dissolved hydrolyzate) or distilled water (in case of the control) was mixed with 980 μl of ABTS radical working solution, and the reduction of absorbance at 734 nm was measured after incubation at 37°C for 10 min in the dark. Sample was replaced by Trolox (6-hydroxy-2,5,7,8-tetramethychroman-2-carboxylic acid), a water-soluble vitamin E analog, for comparative purpose. Antioxidant capacity was expressed as mmol antioxidant activity (percentage inhibition of radical cation absorbance) equivalents/g sample. All determinations were performed at least in triplicate.
Statistical analysis
Analysis of variance (ANOVA) was performed, and means comparison was done using Duncan’s multiple range tests for amino acid comparison between scales and gelatin data. For comparison, the Duncan’s Multiple Range Test was used. Analysis was performed using SPSS 20.0 for Windows (SPSS Inc., Chicago, IL, USA).
Results and discussion
Yield of gelatin
The yields of different molecular weight gelatin extracted from Yak skin are shown in . A1 was the hard particles of solid, while A6 was soft crystallizing solid. The yield of A6 was only 4.32%, while it increased with increasing molecular weight. The yield of A1 was approximately sixfold higher than that of A6.
Table 1. Yield (% of wet weight) of different molecular weight gelatin extracted from the Yak skin.
Molecular weight distribution of gelatin
The SDS-PAGE pattern of gelatin showed major bands in the molecular weight range of 17–180 kDa (). These bands represented α-chain and β-component, respectively. In addition to major bands, the range of molecular weight of the sample was pretty wide, but the average molecular weight of each sample was gradually smaller. The SDS-PAGE pattern showed that the molecular weight of A1 and A2 was higher than 55 kDa, A3-A6 was relatively dispersed, and A6 was obviously below 26 kDa.
UV-Vis absorption spectra of gelatin
The UV-Vis absorption spectra of different molecular weight gelatin extracted from Yak skin are depicted in . The results showed that the six samples all had the higher absorption recorded in the wavelength region of 190–240 nm, which indicated the presence of peptide bonds in the polypeptide chains of gelatin. The absorption peaks of A1-A6 were 221 nm, 219 nm, 217 nm, 216 nm, 213 nm and 219 nm, respectively. It indicated that the maximum absorption peaks moved to longer wavelengths with the increasing molecular weight, and the HMW gelatin had a higher maximum absorption peaks except A6. Gelatin samples revealed end absorption by UV scan at the beginning of hydrolysis. It was probably caused by the amide bond generated gradually with the further hydrolysis. The gelatin samples had strong absorption peak at 209 nm and 221 nm, which is mainly C = O of n→π* transition contributed by a peptide bond. There was a little absorption in the region of 270–280 nm in all the gelatin samples, which may be due to none aromatic residues like phenylalanine, tyrosine and tryptophan.[Citation21]
Denaturation temperature of gelatin
Thermal denaturation profile of Yak skin gelatin had provided useful clues to the thermal stability of collagen in relation to the environment and the change of helix-coil structure. The denaturation temperature (TD) and denaturation enthalpy (ΔHD) of Yak skin gelatin were calculated from the thermal denaturation curve. As can be seen in , TD of Yak skin gelatin calculated by the DSC thermogram was 103.76–177.14°C. The TD of A1 was higher than A6, and the TD of A2–A5 was irregular. But the denaturation enthalpy (ΔHD) was almost increased with the increasing molecular weight. The ΔHD represented the extent of the ordered structure of a protein.[Citation22] Thus, the data suggested that the extent of the ordered structure of Yak solution was gradually increased with the molecular weight. It was suggested that the endothermic peak of the gelatin in the DSC profile was mainly caused by the protein denaturation or the disruption of hydrogen bonds between protein and water.[Citation23]
Table 2. Denaturation temperature (TD) and denaturation enthalpy (ΔHD) of the different molecular weight gelatin extracted from Yak skin.
The TD of Yak skin was much higher than the pig skin collagen (36.3°C),[Citation24] the collagen of bovine limed split wastes (37.5°C),[Citation25] the collagen of chick tendon (23.5–37.9°C),[Citation26] and the sponge collagen (70°C).[Citation27] Literature search indicated that there was no published investigation on the TD of Yak skin, except that Liu et al.[Citation28] studied the denaturation temperature (133.01–148.76°C) of yak milk caseins. The results showed that the Yak skin gelatin had excellent thermal stability. One of the possible reasons may be that Yak lives in high elevation and low temperature local. So the Yak skin fiber had more compact structure. Obviously, Yak skin gelatin was suitable proteins for use in products in which higher thermal stability was required. For example, it could serve as heat resistant agents to protect the pharmaceuticals with biological activity like live vaccine stabilizer, and applied to the biological materials like heat-resisting coating stability.
FT-IR spectra of gelatin
FT-IR spectra of different molecular weight gelatin extracted from Yak skin are depicted in . FT-IR spectroscopy has been used to study the changes in functional groups and secondary structure of gelatin. All gelatin samples had the major peaks in amide region, but showed slight differences in the spectra. FT-IR spectra of Yak skin gelatin had the amide I band at 1600–1700 cm−Citation1, which was similar to the report by Muyonga et al.[Citation29] The amide I vibration mode was primarily a C = O stretching vibration coupled to contributions from the—CN stretch,—CCN deformation and in-plane—NH bending modes.[Citation30,Citation31] The absorption peak at amide I was characteristic for the coil structure of gelatin.[Citation32] Compared with other gelatins, the amide I peak of A1 showed the higher amplitudes and wide peak, which was probably caused by more complete triple helix structure than other LMW gelatin.
Besides these, the samples also had the peaks at 1560–1500 cm−Citation1 and 1234–1237 cm−Citation1, which were the amide II band and the amide III band. The amide II band was generally considered to be much more sensitive to hydration than to secondary structure change,[Citation33] and Amide II vibration mode of gelatins at 1560–1500 cm−Citation1 was reported by Yakimets et al.[Citation32] It is attributed to an out-of-phase combination of—CN stretch and in-plane—NH deformation modes of the peptide group.[Citation30,Citation31] Small amide III bands of all gelatins observed at 1234–1237 cm−Citation1, which indicated the disorder in gelatin molecules, were associated with loss of triple helix state.[Citation34] Amide II band and amide III band in the samples all became smaller with the decreasing molecular weight, even the amide III of A6 disappeared. It can be predicted that the LMW had a little complete triple helix structure.
Furthermore, amide A peak (3169–3366 cm−Citation1) was found at samples, which represented NH–stretching coupled with hydrogen bonding. A free NH-stretching vibration normally occurs in the range of 3400–3440 cm−Citation1, while the position is shifted to lower frequencies. A1, A2, A3 and A4 showed higher amplitude than A5 and A6, which indicated the higher free amino groups caused by this molecular weight. This was in agreement with the marked degradation of α-and β-chains (). Amide B peak was found at 3128–3190 cm−Citation1, represented—CH stretching and—NH2.
Amino acid composition of gelatin
Amino acid compositions of different molecular weight Yak skin gelatin samples are showed in . It showed a similar amino acid profile in each samples. Like other gelatin, all samples were rich in glycine, proline, hydroxyproline, glutamic, and alanine acid, but low in methionine, tyrosine and histidine. And the result indicated that high melocular weight Yak skin gelatin had a higher amino acid component especially the component of imino acid content.
Table 3. Amino acid composition of different molecular weight gelatin extracted from Yak skin.
With the increasing molecular weight, the amino acid component content increased. And the amino acid content of A5 (17–26 kDa) and A6 (below 17 kDa) was the lowest. The amino acid composition of Yak skin gelatins was different from those reported for other species. Hydroxyproline and proline are believed to play a singular role in the stabilization of the triple-stranded collagen helix due to its hydrogen bonding ability through its—OH group.[Citation35] Imino acid content of Yak skin gelatin (19–23%) was approximated with Nile perch gelatin (21.5%)[Citation36] and Yak bone gelatin (20.19%).[Citation37]
Emulsifying and foaming properties of gelatin
Gelatin was used as a foaming and emulsifying agent in food, pharmaceutical, medical, and technical applications due to its surface-active properties. Although gelatin was generally a weaker emulsifier than other surface-active substances such as globular proteins and gum arabic, it could play a special role at a lot of aspects, for example, it could be used in conjunction with anionic surfactants to improve its effectiveness as an emulsifier.[Citation38]
Emulsion activity index (EAI) and emulsion stability index (ESI) of different molecular weight Yak skin gelatin are presented in . EAI of gelatin increased with the decreasing molecular weight in general (p < 0.05), while ESI of Yak skin gelatin was just opposite. That was presumed that small peptides could stabilize the emulsifying ability more effectively. According to Dickinson,[Citation39] the relatively HMW of modified polymers had poorer emulsifying performance. This analysis is consistent with my result.
Table 4. Emulsifying properties of different molecular weight gelatin extracted from Yak skin.
Foam formation ability is another important property of gelatin and commonly used in food products. Foam expansion (FE) and foam stability (FS) of different molecular weight Yak skin gelatin are presented in . In general, FE and FS of gelatin increased with the decrease of molecular weight (p < 0.05). We can see that foams of LMW gelatin were denser and more stable. The foaming ability of proteins is related to their film-forming ability at the air-water interface, while the stability of foams depends on various parameters, such as the rate of attaining equilibrium surface tension, bulk and surface viscosities, steric stabilization, and electrical repulsion between the two sides of the foam lamella.[Citation40] It is presumed that the LMW Yak skin gelatin has higher surface viscosities and the lower steric stabilization. And it could serve like emulsifier and for the in food industry.
Antioxidant properties of gelatin
The antioxidant activity of protein hydrolyzates may not be attributed to a single mechanism. Therefore, different assays (DPPH, FRAP and ABTS) were performed to study the antioxidant properties of the gelatin peptides. DPPH radical scavenging is an important property of an antioxidant and can be determined by the inhibition of lipid peroxidation and the ability to reduce the DPPH radical to the more stable DPPH form.[Citation41] The DPPH radical–scavenging activity of the different molecular weight gelatin from Yak skin is shown in . As can be seen, DPPH radical−scavenging activity increased with decreasing molecular weight of Yak skin gelatin. The DPPH radical−scavenging activity of A1 was only 8.28%, it had a little DPPH radical activity. And the DPPH radical−scavenging activities of A2-A6 were all above 50%, especially, A6 was 68.41%. It indicated that both A2-A6 may contain certain peptides that are electron donors and can react with free radicals to terminate the radical chain reaction.[Citation42]
Figure 4. The DPPH radical−scavenging activity of the different molecular weight gelatin from Yak skin. Different letters indicate significant differences (p < 0.05).
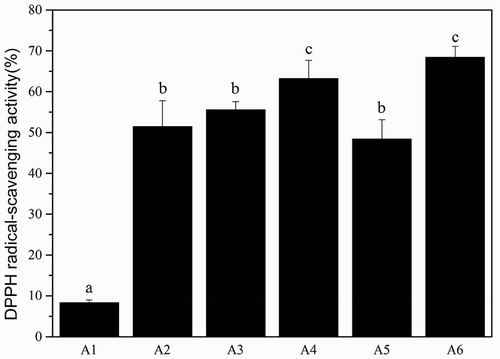
The reducing capacity of a given compound may serve as a significant indicator of its potential antioxidant activity. An electron donating reducing agent is able to donate an electron to a free radical. As a result, the radical is neutralized and the reduced species subsequently acquires a proton from solution.[Citation43] In the FRAP assay, the presence of reducing agents in the tested samples results in the formation of the complex tripiridiltriazine (TPTZ)–Fe(II), which is monitored at 595 nm. As shown in , A6 showed approximately a ninefold higher ferric iron reducing ability than A1–A5 (p < 0.05). The hydrolysis of gelatin chains into peptides may give rise to both the release of sequences with antioxidant properties and the exposition of previously hidden amino acid residues and side chains with antioxidant activity.[Citation44] showed the ABTS total antioxidant capacity of different molecular weight gelatin from Yak skin. A6 also showed approximately a sixfold higher ABTS radical scavenging ability than A1-A5 (p < 0.05).
Figure 5. The FRAP total antioxidant capacity of the different molecular weight gelatin from Yak skin. Different letters indicate significant differences (p < 0.05).
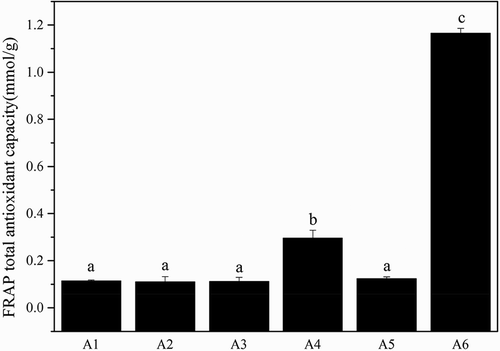
Figure 6. The ABTS total antioxidant capacity of the different molecular weight gelatin from Yak skin. Different letters indicate significant differences (p < 0.05).
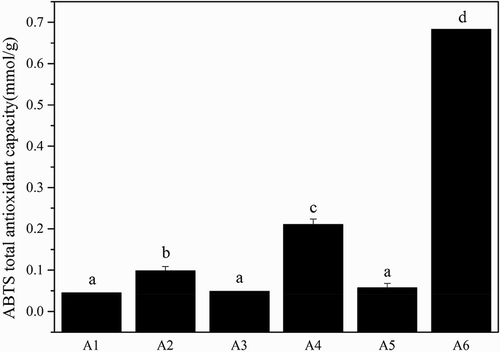
According to the findings described above, A6 exhibited highest antioxidant ability among the gelatin samples. One possible explanation may be that the molecular weight of Yak skin gelatin at 17-26 kDa (A6) possibly contains amino acids or peptides which act as electron donors and can react with free radicals to give rise to more stable products and terminate radical chain reactions. Since antioxidant activity of protein hydrolyzates is highly dependent on their sequence and the amino acid composition,[Citation45] the LMW Yak skin gelatin could serve as antioxidant healthcare products.
Conclusion
There are considerable differences between HMW and LMW gelatin from Yak skin in the aspect of oxidation resistance, thermal stability, foamability, emulsibility and other physicochemical properties. These data presented in this study show that HMW gelatin has higher imino acid content, while the LMW gelatin has better oxidation resistance, foamability and emulsibility. And both the HMW and LMW Yak skin gelatin have great thermal stability. It is worthnoting that Yak skin gelatin has excellent thermostability compared with other mammals and sea creatures. So the Yak skin has great potential application in the field of food and medicine. However, the Yak skin has been always used as leather and overlooked the other applications with high added value. It is important and significant to develop and utilize this valuable resource. This study is only a preliminary report of work for exploiting gelatin from underutilized resources. Further researches are recommended to focus on how to develop and use the thermotolerance and antioxidant of Yak skin gelatin, investigate the correlation between physicochemical and functional properties and different pretreatment, extraction process and the system of antioxidant.
Funding
This study was supported by the Natural science foundation of Qinghai Province (Qinghai, China; 2015-ZJ-924Q); CAS “Light of West China” program.
Additional information
Funding
References
- Haddar, A.; Sellimi, S.; Ghannouchi, R.; Alvarez, O.M.; Nasri, M.; Bougatef, A. Functional, Antioxidant and Film-Forming Properties of Tuna-Skin Gelatin with a Brown Algae Extract. International Journal of Biological Macromolecules 2012, 51, 477–483.
- Muyonga, J.H.; Cole, C.G.B.; Duodu, K.G. Extraction and Physico-Chemical Characterisation of Nile Perch (Lates niloticus) Skin and Bone Gelatin. Food Hydrocolloids 2004, 18, 581–592.
- Lin, C.C.; Chiou, T.K.; Sung, W.C. Characteristics of Gelatin from Giant Grouper (Epinephelus lanceolatus) Skin. International Journal of Food Properties 2015, 11, 2339–2348.
- Sadowska, M.; Kołodziejska, I.; Niecikowska, C. Isolation of Collagen from the Skins of Baltic Cod (Gadus morhua). Food Chemistry 2003, 81, 257–262.
- Chandra, M.V.; Shamasundar, B.A. Texture Profile Analysis and Functional Properties of Gelatin from the Skin of Three Species of Fresh Water Fish. International Journal of Food Properties 2015, 3, 572–584.
- Long, R.J.; Dong, S.K.; Wei, X.H.; Pu, X.P. The Effect of Supplementary Feeds on the Bodyweight of Yaks in Cold Season. Livestock Production Science 2005, 93, 197–204.
- Long, R.J.; Dong, S.K.; Hu, Z.Z.; Shi, J.J.; Dong, Q.M.; Han, X.T. Digestibility, Nutrient Balance and Urinary Purine Derivative Excretion in Dry Yak Cows Fed Oat Hay at Different Levels of Intake. Livestock Production Science 2004, 88, 27–32.
- Zhang, C.Q.; Xing, Y.X.; Hu, F.Z. Analysis and Evaluation of Amino Acids and Mineral Composition in Skin Glue of Yak. Modern Scientific Instruments 2006, 3, 012.
- Johnston-Banks, F.A. In Food Gels; Springer Netherlands: Heidelberg, 1990; 233–289 pp.
- Tavernier, B.H. Molecular Mass Distribution of Gelatin and Physical Properties. Photographic Gelatin Proceedings 1989, 1, 217–228.
- Dong, Y.P.; Huang, B.X.; Yue, J. The Distribution of Ag+ in Gelatin with respect to Molecular Weight. Chinese Journal of Chemical Physics 2003, 16, 75–78.
- Hu, S.Q.; Zhang, T.T.; Hu, J.; Guo, S.S.; Li, L. Preparation and Properties of ACE-Inhibitory Peptides from Fish Scale Gelatin. Modern Food Science and Technology 2012, 11, 017.
- Laemmli, U.K.;. Cleavage of Structural Proteins during the Assembly of the Head of Bacteriophage T4. Nature 1970, 227, 680–685.
- Lee, J.H.; Kim, J.C. UV-Absorbing and Emulsifying Property of Cinnamic Acid-Conjugated Gelatin. Journal of Dispersion Science and Technology 2015, 36, 1000–1008.
- Bidlingmeyer, B.A.; Cohen, S.A.; Tarvin, T.L. Rapid Analysis of Amino Acids Using Pre-Column Derivatization. Journal of Chromatography B: Biomedical Sciences and Applications 1984, 336, 93–104.
- Zarai, Z.; Balti, R.; Sila, A.; Ali, Y.B.; Gargouri, Y. Helix Aspersa Gelatin as an Emulsifier and Emulsion Stabilizer: Functional Properties and Effects on Pancreatic Lipolysis. Food & Function 2016, 7, 326–336.
- Shahidi, F.; Han, X.Q.; Synowiecki, J. Production and Characteristics of Protein Hydrolysates from Capelin (Mallotus villosus). Food Chemistry 1995, 53, 285–293.
- Bersuder, P.; Hole, M.; Smith, G. Antioxidants from a Heated Histidine-Glucose Model System. I: Investigation of the Antioxidant Role of Histidine and Isolation of Antioxidants by High-Performance Liquid Chromatography. Journal of the American Oil Chemists’ Society 1998, 75, 181–187.
- Benzie, I.F.F.; Strain, J.J. The Ferric Reducing Ability of Plasma (FRAP) as a Measure of “Antioxidant Power”: The FRAP Assay. Analytical Biochemistry 1996, 239, 70–76.
- Re, R.; Pellegrini, N.; Proteggente, A.; Pannala, A.; Yang, M.; Rice-Evans, C. Antioxidant Activity Applying an Improved ABTS Radical Cation Decolorization Assay. Free Radical Biology and Medicine 1999, 26, 1231–1237.
- Sarbon, N.M.; Badii, F.; Howell, N.K. Preparation and Characterisation of Chicken Skin Gelatin as an Alternative to Mammalian Gelatin. Food Hydrocolloids 2013, 30, 143–151.
- Arntfield, S.D.; Murray, E.D. The Influence of Processing Parameters on Food Protein Functionality I. Differential Scanning Calorimetry as an Indicator of Protein Denaturation. Canadian Institute of Food Science and Technology Journal 1981, 14, 289–294.
- Wang, X.S.; Tang, C.H.; Li, B.S.; Yang, X.Q.; Li, L.; Ma, C.Y. Effects of High-Pressure Treatment on Some Physicochemical and Functional Properties of Soy Protein Isolates. Food Hydrocolloids 2008, 22, 560–567.
- Nagai, T.; Araki, Y.; Suzuki, N. Collagen of the Skin of Ocellate Puffer Fish (Takifugu rubripes). Food Chemistry 2002, 78, 173–177.
- Zhang, Z.; Li, G.; Shi, B.I. Physicochemical Properties of Collagen, Gelatin and Collagen Hydrolysate Derived from Bovine Limed Split Wastes. Journal of the Society of Leather Technologists and Chemists 2006, 90, 23.
- Rosenbloom, J.; Harsch, M.; Jimenez, S. Hydroxyproline Content Determines the Denaturation Temperature of Chick Tendon Collagen. Archives of Biochemistry and Biophysics 1973, 158, 478–484.
- Sudharsan, S.; Seedevi, P.; Saravanan, R.; Ramasamy, P.; Kumar, S.V.; Vairamani, S.; Shanmugam, A. Isolation, Characterization and Molecular Weight Determination of Collagen from Marine Sponge Spirastrella Inconstans (Dendy). African Journal of Biotechnology 2013, 12, 504–511.
- Liu, H.N.; Zhang, C.; Zhang, H.; Guo, H.Y.; Wang, P.J.; Zhu, Y.B.; Ren, F.Z. Ph Treatment as an Effective Tool to Select the Functional and Structural Properties of Yak Milk Caseins. Journal of Dairy Science 2013, 96, 5494–5500.
- Muyonga, J.H.; Cole, C.G.B.; Duodu, K.G. Characterisation of Acid Soluble Collagen from Skins of Young and Adult Nile Perch (Lates niloticus). Food Chemistry 2004, 85, 81–89.
- Bandekar, J.;. Amide Modes and Protein Conformation. Biochimica Et Biophysica Acta (Bba)-Protein Structure and Molecular Enzymology 1992, 1120, 123–143.
- Lavialle, F.; Adams, R.G.; Levin, I.W. Infrared Spectroscopic Study of the Secondary Structure of Melittin in Water, 2-Chloroethanol, and Phospholipid Bilayer Dispersions. Biochemistry 1982, 21, 2305–2312.
- Yakimets, I.; Wellner, N.; Smith, A.C.; Wilson, R.H.; Farhat, I.; Mitchell, J. Mechanical Properties with respect to Water Content of Gelatin Films in Glassy State. Polymer 2005, 46, 12577–12585.
- Wellner, N.; Belton, P.S.; Tatham, A.S. Fourier Transform IR Spectroscopic Study of Hydration-Induced Structure Changes in the Solid State of Ω-Gliadins. Biochemical Journal 1996, 319, 741–747.
- Friess, W.; Lee, G. Basic Thermoanalytical Studies of Insoluble Collagen Matrices. Biomaterials 1996, 17, 2289–2294.
- Burjanadze, T.V.;. Hydroxyproline Content and Location in Relation to Collagen Thermal Stability. Biopolymers 1979, 18, 931–938.
- Muyonga, J.H.; Cole, C.G.B.; Duodu, K.G. Extraction and Physico-Chemical Characterisation of Nile Perch (Lates niloticus) Skin and Bone Gelatin. Food Hydrocolloids 2004, 18, 581–592.
- Li, F.; Jia, D.; Yao, K. Amino Acid Composition and Functional Properties of Collagen Polypeptide from Yak (Bos grunniens) Bone. LWT-Food Science and Technology 2009, 42, 945–949.
- Olijve, J.; Mori, F.; Toda, Y. Influence of the Molecular-Weight Distribution of Gelatin on Emulsion Stability. Journal of Colloid and Interface Science 2001, 243, 476–482.
- Dickinson, E.;. Hydrocolloids at Interfaces and the Influence on the Properties of Dispersed Systems. Food Hydrocolloids 2003, 17, 25–39.
- Liu, J.; Xu, G.; Yuan, S.; Jiang, P. The Effect of Macromolecules on Foam Stability in Sodium Dodecyl Sulfate/Cetylpyridinium Bromide Mixtures. Journal of Dispersion Science and Technology 2003, 24, 779–787.
- Blokhina, O.; Virolainen, E.; Fagerstedt, K.V. Antioxidants, Oxidative Damage and Oxygen Deprivation Stress: A Review. Annals of Botany 2003, 91, 179–194.
- Yang, J.I.; Ho, H.Y.; Chu, Y.J.; Chow, C.J. Characteristic and Antioxidant Activity of Retorted Gelatin Hydrolysates from Cobia (Rachycentron canadum) Skin. Food Chemistry 2008, 110, 128–136.
- Wang, H.; Gao, X.D.; Zhou, G.C.; Cai, L.; Yao, W.B. In Vitro and in Vivo Antioxidant Activity of Aqueous Extract from Choerospondias axillaris Fruit. Food Chemistry 2008, 106, 888–895.
- Giménez, B.; Alemán, A.; Montero, P.; Gómez-Guillén, M.C. Antioxidant and Functional Properties of Gelatin Hydrolysates Obtained from Skin of Sole and Squid. Food Chemistry 2009, 114, 976–983.
- Chen, H.M.; Muramoto, K.; Yamauchi, F.; Fujimoto, K.; Nokihara, K. Antioxidative Properties of Histidine-Containing Peptides Designed from Peptide Fragments Found in the Digests of a Soybean Protein. Journal of Agricultural and Food Chemistry 1998, 46, 49–53.