ABSTRACT
Mango fruits from different varieties vary in aroma and taste. In this study, methanol extracts of fruit pulps obtained from seven Indian varieties of Mangifera indica (Amrapali, Fazli, Golapkhas, Gopalbhog, Himsagar, Langra, and Mohanbhog) were profiled using a metabolomics approach. Chemometric methods were used to understand the contribution of metabolites to varietal differences. The extracts were also assayed for pancreatic lipase inhibitory activities in vitro. All the pulp extracts were analyzed by GC-MS and HPLC to detect their metabolites. In sum, 63 metabolites were identified. The varieties were distinctly different chemically in terms of the identified metabolites, as revealed by principal component analysis (PCA) and partial least squares – discriminant analysis (PLS-DA). From the biplot and VIP scores, it was observed that in addition to some organic acids, amino acids, sugars, and phenolic constituents (e.g., benzene-1,2,4-triol, mangiferin, and pyrogallol) contributed to this variation. All the varieties also inhibited pancreatic lipase in a dose-dependent manner. Gallic acid (IC50 value 0.47 nM) and 4-hydroxy benzoic acid (IC50 value 1.15 nM) showed high anti-lipase activity. Three amino acids (L-proline (IC50 value 0.01 µM), L-alanine (IC50 value 0.14 µM) and aspartic acid (IC50 value 0.02 µM)), and the inorganic acid phosphoric acid (0.02 µM) also showed activity against pancreatic lipase.
Introduction
Treatment of obesity, a lipid metabolism disorder, has involved the inhibition of pancreatic lipase (PL).[Citation1] PL is the most important acid-stable enzyme involved in the digestion of fat in the body. This enzyme hydrolyses fat into fatty acids and glycerol. This hydrolysis process makes the fat absorbable. Extra fat cannot be absorbed if fat hydrolysis is prevented by inhibitors of this enzyme. Therefore, PL inhibition can be an appreciable way to prevent diseases caused by fat deposition.[Citation2] One of the approved options for the treatment of obesity and long-term weight management is the use of a lipase inhibitor.[Citation3] Mango (Mangifera indica L.) fruits are appreciated for their taste globally. The taste and aroma of mangos vary widely depending on the variety as well as the stage of ripening. Mango fruits are reported to contain carotenoids[Citation4–Citation6]; tocopherols[Citation5]; phenolics, including gallic acid, methyl gallate, methyl galloylgallate and digallic acid, mangiferin and isomers, and flavonoids such as quercetin and derivatives, kaempferol, and epicatechin-3-O-gallate[Citation7–Citation9]; ascorbic acid[Citation8]; different sugars; organic acids[Citation10]; a number of amino acids; shikimic acid; trigonelline[Citation11]; and aromatic volatile oils.[Citation12–Citation15] However, to the best of our knowledge, detailed analyses of different varieties of mango fruit pulps in terms of different sugars, polyols, organic acids, amino acids, fatty acids, etc., are lacking.
Mango fruits are also sources of bioactive compounds with potential health benefits. Mango extracts have been found to have activity in human breast cancer cells in vitro.[Citation16] Mango fruit extract promoted endothelial cell migration beneficial in diseases related to the impaired formation of blood vessels.[Citation17] The fruits have also exhibited antioxidant potential in reducing triglyceride levels (very low density lipoprotein, VLDL).[Citation18] Incorporating freeze-dried mango pulp in diets reduced adiposity and lipid profiles in mice fed a high fat diet.[Citation19] Moreover, the regular consumption of freeze-dried mango was shown to reduce fasting glucose levels in obese individuals and to possibly be able to prevent an increase in body fat.[Citation20]
The objectives of the study were (1) to explore the metabolite profiles of Indian mango varieties to identify the metabolites contributing to varietal differences and (2) to assess the lipase inhibitory property of mango fruit pulps.
Materials and methods
Plant material
Seven different varieties of M. indica fruits were collected from the agricultural field station of the University of Calcutta, Baruipur, Kolkata, India. The varieties were Amrapali, Fazli, Golapkhas, Gopalbhog, Himsagar, Langra, and Mohanbhog. Fully mature mangoes were collected during peak fruiting season (May–June, 2014). Amrapali is a hybrid variety.[Citation21] The surfaces of the fruits were cleaned with distilled water just after harvesting. The fruits were peeled, and the pulp portions were used for the experiments.
Sample preparation
The pulp portion of each variety was homogenized with liquid nitrogen and extracted with methanol for 1 hour by reflux at 80°C in a water bath. The extract obtained after filtration was evaporated to dryness under reduced pressure with a rotary evaporator at room temperature (35–40°C). The dried extract was preserved at −20°C for further study. The process was repeated to obtain biological replicas. Six biological replicas were prepared for each fruit type.
GC-MS analysis
Crude fruit extract (10 ± 5 mg) and adonitol (internal standard 20 µl of 0.2 mg/ml solution) dissolved in MeOH: H2O (1:1) was distributed into microfuge tubes and evaporated to dryness. The residue was dissolved in 10 µl of methoxyamine hydrochloride (20 mg/ml in pyridine) and subsequently shaken for 90 min at 30°C. N-Methyl-N-trimethylsilyltrifluoroacetamide (MSTFA) (90 µl) was added and shaken at 37°C for 30 min for trimethylsilylation at acidic proton positions. A mixture of fatty acid methyl ester (FAME) markers (2 µl) was added.[Citation22] GC-MS analysis (Agilent 7890 A GC with software driver version 4.019054) equipped with Agilent 5975C inert MSD with triple axix detector was performed following a previously mentioned method.[Citation23] In brief, an HP-5MS capillary column (Agilent J&W GC columns, USA) (length 30 m plus DuraGuard 10 m, diameter 0.25 mm narrow bore, film 0.25 μm) was used. The analysis was performed under the following oven temperature program: oven ramp 60°C (1 min hold) to 325°C at 10°C/min, 10 min hold before cool down, 37.5 min run time. The injection temperature was set at 250°C, the MS transfer line at 290°C, and the ion source at 230°C. Helium was used as the carrier gas at a constant flow rate of 0.723 ml/min (carrier linear velocity 31.141 cm/s). Each sample (1 µl) was injected via the split mode (split ratio 1:5) onto the GC column. The metabolites were identified by comparing their mass spectral fragmentation patterns, retention times (RTs) and retention indices (RIs) with those present in the Agilent Fiehn Metabolomics Library using the Agilent retention time locking (RTL) method. The automated mass spectral deconvolution and identification system (AMDIS) was used to deconvolute GC-MS results and to identify the chromatographic peaks. Many of the metabolites were also further confirmed by matching their RTs with those of authentic compounds.
HPLC analysis
Crude methanol extract of mango fruit pulp was dissolved in 1 ml of a 1:1 mixture of water and methanol. The solution was centrifuged at 10,000 rpm for 15 min at ambient temperature. The supernatant was used for mangiferin content analysis by HPLC. The analysis was performed with the modified method of Schieber et al.[Citation24] The HPLC system consisted of an Agilent 1260 Infinity with a diode array detector and a C18 Eclipse Plus analytical column (3.5 µm x 4.6 mm x 100 mm). The detection wavelengths were 258, 317, and 366 nm. The gradient elution was performed with the mobile phases as follows: 0–2 min, 5% B; 2–10 min, 5–25% B; 10–40 min, 25–55% B; 40–45 min, 55–90% B; 45–50 min, 90–55% B; 50–55 min, 55–5% B; 55–60 min, 5% B [2% acetic acid (A) and acetonitrile: 0.5% acetic acid (1:1 v/v) (B)]. The flow rate of the mobile phase was 0.6 ml/min. The injection volume was 10 µl. Mangiferin was identified by comparing its RT and absorbance spectrum with those of the authentic sample.
Pancreatic lipase inhibition assay
Lipase inhibitory activity was assayed by modifying the method of Mc Dougal et al.[Citation25] Each reaction mixture consisted of 400 µl of tris buffer, 150 µl of lipase enzyme (ex-porcine pancreas), 50 µl of fruit extracts as inhibitor, and 450 µl of the substrate p-nitrophenyl laurate. The reaction mixture was incubated for 2 hours at 37°C and centrifuged for 1 min at 16,000 rpm. The spectrophotometric absorbance was measured at 400 nm. The percentage inhibition of enzyme activity was calculated as [(A0 – AC)/A0] X 100 (A0 = absorbance without extract, AC = absorbance with extract). A regression equation was calculated from the concentrations of the extracts, and the percentage inhibition. IC50 value (concentration of sample required to inhibit the enzyme activity by 50%) was calculated from the regression equation. A lower IC50 value indicates a higher enzyme inhibitory activity.
Determination of total phenol content
Total phenol content (TPC) was determined by using the Folin–Ciocalteau reagent in alkaline medium[Citation26] and was expressed as gallic acid equivalents (GAE) (equivalent to µg gallic acid/g fruit extract). To a 3 ml aliquot of fruit extract, 0.5 ml of Folin–Ciocalteau reagent was added. After 3 min, 2 ml of 20% Na2CO3 was added. The reaction mixture was kept in a boiling water bath for 1 min. The absorbance of the mixture was measured at 650 nm against a reagent blank.
Determination of total flavonoid content
Total flavonoid content (TFC) was determined following Kim et al.[Citation27] and was expressed as catechin equivalent (CE) (equivalent to µg of catechin/g fruit extract). To a 1 ml aliquot of fruit extract, 4 ml of double distilled water and 0.3 ml of 5% NaNO2 were added. After 5 min, 0.3 ml of 10% AlCl3 was added. After 1 min, 2 ml of 1 (M) NaOH was added, and the reaction mixture was immediately diluted to 10 ml with the addition of 2.4 ml of double distilled water. The absorbance of the light pink mixture was measured at 510 nm.
Statistical analysis
The relative response ratio of each metabolite was calculated by normalizing the peak area of the metabolites by sample fresh weight and by the peak area of the internal standard. Statistical analysis was performed using MetaboAnalyst 3.0 and SIMCA 13.0.2. The relative response ratios of different fruit metabolites were further normalized after log transformation for principal component analysis (PCA) and partial least squares – discriminant analysis (PLS-DA) to understand metabolic variation. The data were also correlated with the lipase inhibitory activities. For this, normalized data were imported to SIMCA 13.0.2. PLS was performed with all the groups and lipase inhibition. The metabolites were also analyzed by one way ANOVA.
Results and discussion
Metabolic profiling
In sum, 63 metabolites were identified () by GC-MS and HPLC from the seven mango varieties. GC-MS-identified metabolites included organic acids (20), amino acids (10), fatty acids (6), phenols (8), sugar and polyols (13) and other constituents (5), detected using the Agilent Fiehn Metabolomics Library. Mangiferin was detected by HPLC. A comparison of the relative response ratios of these metabolites in different varieties is presented in Supplementary Table 1.
Table 1. GC-MS identified metabolites from mango pulp.
Normalized metabolite data were used for semi-quantitative comparison. The data were initially analyzed from the heat map () generated by the MetaboAnalyst software to determine if the mango varieties could be discriminated from their metabolite profiles, revealing that the seven varieties could be separated. Post hoc tests show that the majority of the metabolites were significantly different from each other (Supplementary Table 2) Hierarchical cluster analysis separated Fazli and Golapkhas from the others. Overall differences in the metabolite profiles were visible from the heatmap. To further study these differences, the data were analyzed by PCA and PLS-DA. Both the models clearly segregated the varieties. Seventy four percent of the metabolites contributed to the separation of the varieties in PCA. Fifty seven percent of the metabolites contributed to the separation of the varieties by PLS-DA. Three-dimensional rotation of the PCA score plot () as well as the PLS-DA score plot () also showed Fazli and Golapkhas to be distinctly separated from the others. The biplot () generated from PCA identified the metabolites responsible for the varietal differences. The thirty most-influential metabolites for the varietal differences were also selected () from the VIP score generated from the PLS-DA model. The color codes depicted in the figure show that the Gopalbhog variety contained low levels of metabolites. Fazli contained high levels of metabolites. The biplot and the VIP scores identified similar metabolites. The results suggested that in addition to the organic acids, amino acids, sugars, fatty acids, and phenolic constituents, e.g., benzene-1,2,4-triol, mangiferin, and pyrogallol, were also responsible for the variation. The fifteen most important metabolites responsible for varietal differences were fumaric acid, 2-amino-3-methoxybenzoic acid, tartaric acid, raffinose, ribonic acid gamma lactone, sedoheptulose, lauric acid, myo-inositol, D-mannitol, sucrose, nicotinic acid, benzene-1,2,4-triol, L-pyroglutamic acid, gluconic acid, and beta-alanine. The relative amount of the xanthone mangiferin also varied, being high in most varieties, low in Amrapali, and undetectable in Golapkhas and Himsagar. The total phenol and flavonoid contents were also considered in multivariate analyses. However, they were not found to be important variables for varietal separation. The distinct separation of Fazli and Golapkhas from the other varieties was due to the presence of high amounts of fumaric acid, tartaric acid, ribonic acid gamma lactone, nicotinic acid, glycolic acid, oxalic acid, L-pyroglutamic acid, beta-alanine, myo-inositol, and benzene-1,2,4-triol in both varieties. The two varieties also contained low levels of melezitose and raffinose. Based on their metabolite profiles, different varieties of mango fruit pulps were clearly shown to chemically differ. This study may be helpful in identifying mango varieties.
Table 2. Lipase inhibitory activity of mango fruit pulp extract.
Figure 1. Heat map illustrating visual differences in metabolite levels detected from seven varieties of mango fruit pulp, using a color scale. Hierarchical cluster analysis is combined with the heat map.
Red color indicates higher relative concentration and blue color indicates lower relative concentration.
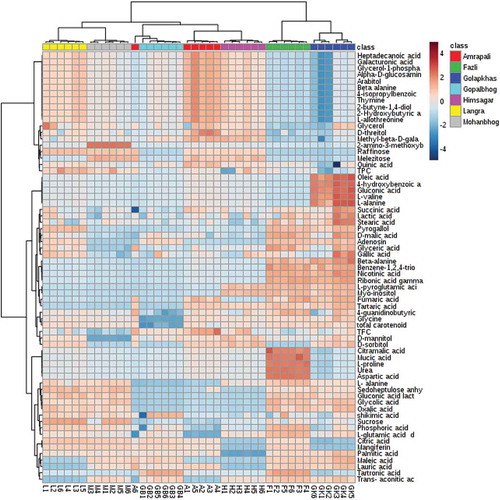
Lipase inhibitory activity
Extracts of mango pulp from all seven varieties collected during the study inhibited PL in a dose-dependent manner. Lipase inhibitory activity, as determined by the IC50 values, ranged from 1.6 mg/ml in Gopalbhog to 5.2 mg/ml in Fazli. Thus, Gopalbhog showed the highest activity and Fazli the lowest. The IC50 values of the varieties are presented in . The present in vitro study thus provides support to the previous report regarding the reduction of body fat in obese persons upon the consumption of mango pulp[Citation19,Citation20] because PL inhibition is involved in reducing fat absorption and treatment of obesity.[Citation1]
Bioactivity and metabolite profiles were subjected to multivariate data analysis. The objective was to correlate the activities (enzyme inhibition percent) of fruit extracts at a concentration of 1.7 mg/ml with metabolites detected by chromatographic analyses. The mangoes could be categorized into high activity (Gopalbhog, Golapkhash, Himsagar, Mohanbhog), medium activity (Amrapali, Langra) and low activity (Fazli) groups. A PLS score plot and an OPLS score plot () show that the mango varieties were clearly segregated by activity into three distinct groups ().
Figure 6. PLS and OPLS score plots segregating varieties on the basis of lipase inhibition activities.
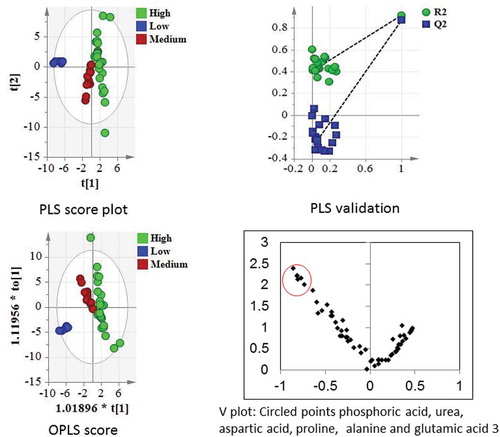
For the PLS model, model validation parameters such as goodness of fit (R2Y: 0.917) and goodness of prediction (Q2: 0.871) were found to be significant. Similarly, for the OPLS model, R2Y was 0.887, and Q2 was 0.871, demonstrating the supervised model to be robust. Additionally, the CV-ANOVA p value of the OPLS model was 2.77E-16. R2 and Q2 values for the PLS-DA model were 0.991 and 0.984, respectively. Further model validation relied on permutation analysis. The analysis was performed only for the best model. If the performance score of the original data lies outside the distribution, then the result is significant.[Citation28] Using 100 permutation tests, the p value was reported as p < 0.01, denoting that none of the results were better than the original one.
Identification of lipase inhibitors
The major metabolites distinguishing the different varieties of mango did not contribute to lipase inhibitory activity. Though not highly significant, some of the metabolites such as urea, proline and glutamic acid () responsible for lipase inhibitory activity were positively correlated to Fazli and negatively correlated to Gopalbhog. These metabolites and a few other metabolites identified from mango fruit pulp were assayed for lipase inhibitory properties. Gallic acid and 4-hydroxy benzoic acid individually showed high anti-lipase activity. It was also observed that L-alanine, L-proline, and aspartic acid inhibited PL in vitro. The IC50 values of these metabolites for lipase inhibition are presented in . In addition, the xanthone compound mangiferin was previously reported to have anti-lipase property with an IC50 value 273 µM.[Citation29] However, there may be synergistic lipase inhibition activities from different components present in mango fruit pulp. Orlistat, a commercially available lipase inhibitor[Citation18], was used as a positive control. Orlistat showed an IC50 value of 0.31 nM. In the present findings, gallic acid had an activity close to that of orlistat. Body fat accumulation can be prevented by inhibiting PL.[Citation2] The identified amino acids as well as gallic acid and 4-hydroxy benzoic acid could be important in this regard. Further in vivo studies are required. However, consumption of the fruit with all components may have a more beneficial effect on health, as suggested previously.[Citation14]
Table 3. Lipase inhibitory activity of metabolites.
Conclusion
This study revealed that the methanol extracts of seven varieties of mango pulp exhibited remarkable differences between the metabolite profiles of different varieties. Hierarchical cluster analysis and multivariate analyses clearly separated Fazli and Golapkhas from the rest of the varieties. The pulp extracts also showed lipase inhibitory activities, which also differed across varieties. Multivariate analysis identified a few amino acids, urea, and phosphoric acid to be positively correlated to the activity. Gallic acid, 4-hydroxy benzoic acid and three amino acids (L-proline, L-alanine, aspartic acid) identified from the fruit extracts inhibited the enzyme in vitro. Phosphoric acid also inhibited lipase. The phenol gallic acid had an activity very close to that of the commercial lipase inhibitor orlistat.
LJFP_A_1357041_Supplementary_Table_2.docx
Download MS Word (16.8 KB)LJFP_A_1357041_Supplementary_Table_1.docx
Download MS Word (30.1 KB)Acknowledgments
The authors acknowledge the Department of Science and Technology FIST Programme for instrumental facilities.
Supplemental materials
Supplemental data for this article can be accessed on the publisher’s website.
References
- Birari, R. B.; Bhutani, K. K. Pancreatic Lipase Inhibitors from Natural Sources: Unexplored Potential. Drug Discov. Today. 2007, 12, 879–889. DOI: 10.1016/j.drudis.2007.07.024.
- Roh, C.; Jung, U.; Jo, S. K. Screening of Anti-Obesity Agent from Herbal Mixtures. Molecules. 2012, 17, 3630–3638. DOI: 10.3390/molecules17043630.
- Apovian, C. M.; Garvey, W. T.; Ryan, D. H. Challenging Obesity: Patient, Provider, and Expert Perspectives on the Roles of Available and Emerging Nonsurgical Therapies. Obes. 2015, 23, S1–S26. DOI: 10.1002/oby.21140.
- Mercadante, A. Z.; Rodriguez-Amaya, D. B.; Britton, G. HPLC and Mass Spectrometric Analysis of Carotenoids from Mango. J. Agric. Food Chem. 1997, 45, 120–123. DOI: 10.1021/jf960276j.
- Ornelas-Paz, J. D. J.; Yahia, E. M.; Gardea-Bejar, A. Identification and Quantification of Xanthophylls Esters, Carotenes, and Tocopherols in the Fruit of Seven Mexican Mango Cultivars by Liquid-Chromatography-Atmospheric Pressure Chemical Ionization-Time –Of-Flight Mass Spectrometry [Lc-(Apcl+)-Ms]. J. Agric. Food Chem. 2007, 55, 6628–6635. DOI: 10.1021/jf0706981.
- Fraser, P. D.; Enfissi, E. M. A.; Goodfellow, M.; Eguchi, T.; Brameley, P. M. Metabolite Profiling of Plant Carotenoids Using the Matrix-Assisted Laser Desorption Ionization Time-Of-Flight Mass Spectrometry. Plant J. 2007, 49, 552–564. DOI: 10.1111/j.1365-313X.2006.02949.x.
- Dorta, E.; Gonzalez, M.; Lobo, M. G.; Sanchez-Moreno, C.; De Ancos, B. Screening of Phenolic Compounds in By-product Extracts from Mangoes (Mangifera Indica L.) by HPLC-ESI-QTOF-MS and Multivariate Analysis for Use as a Food Ingredient. Food Res. Int. 2014, 57, 51–60. DOI: 10.1016/j.foodres.2014.01.012.
- Sogi, D. S.; Siddiq, M.; Roidoung, S.; Dolan, K. D. Total Phenolics, Carotenoids, Ascorbic Acid, and Antioxidant Properties of Fresh-Cut Mango (Mangifera Indica L., Cv. Tommy Atkin) as Affected by Infrared Heat Treatment. J. Food Sci. 2012, 77, C1197–1202.
- Pierson, J. T.; Monteith, G. R.; Roberts-Thomson, S. J.; Dietzgen, R. G.; Gidley, M. J.; Shaw, P. N. Phytochemical Extraction, Characterization and Comparative Distribution across Four Mango (Mangifera Indica L.) Fruit Varieties. Food Chem. 2014, 149, 253–263. DOI: 10.1016/j.foodchem.2013.10.108.
- Liu, F.; Fu, S.; Bi, X.; Chen, F.; Liao, X.; Hu, X.; Wu, J. Physico-Chemical and Antioxidant Properties of Four Mango (Mangifera Indica L.) Cultivars in China. Food Chem. 2013, 138, 396–405.
- Koda, M.; Furihata, K.; Wei, F.; Miyakawa, T.; Tanokura, M. Metabolic Discrimination of Mango Juice from Various Cultivars by Band-Selective NMR Spectroscopy. J. Agric. Food Chem. 2012, 60, 1158–1166. DOI: 10.1021/jf2041438.
- Lalel, H. J. D.; Singh, Z.; Tan, S. C. Glycosidically-Bound Aroma Volatile Compounds in the Skin and Pulp of ‘Kensington Pride’ Mango Fruit at Different Stages of Maturity. Postharvest Biol. Technol. 2003, 29, 205–218. DOI: 10.1016/S0925-5214(02)00250-8.
- Andrade, E. H. A.; Maria, J. G. S.; Zoghbi, M. G. B. Aroma Volatile Constituents of Brazilian Varieties of Mango Fruit. J. Food Composition Anal. 2000, 13, 27–33. DOI: 10.1006/jfca.1999.0841.
- Ansari, A. H.; Ali, M.; Velasco-Negueruela, A.; Perez-Alonso, M. J. Volatile Constituents of the Fruits of Three Mango Cultivars, Mangifera Indica L. J. Essential Oil Res. 1999, 11, 65–68. DOI: 10.1080/10412905.1999.9701073.
- Ansari, S. H.; Ali, M.; Velasco-Negueruela, A.; Perez-Alonso, M. J. Characterization of Volatile Oil Constituents of Mango ‘Qalmi’ (Mangifera Indica L.) Fruit. J. Essential Oil Res. 2004, 16, 417–419. DOI: 10.1080/10412905.2004.9698759.
- Wilkinson, A. S.; Flanagan, B. M.; Pierson, J. T.; Hewavitharana, A. K.; Dietzgen, R. G.; Shaw, P. N.; Roberts-Thomson, S. J.; Monteith, G. R.; Gidley, M. J. Bioactivity of Mango Flesh and Peel Extracts on Peroxisome Proliferator-Activated Receptor Γ[Pparγ] Activation and MCF-7 Cell Proliferation: Fraction and Fruit Variability. J. Food Sci. 2011, 76, H11–H18. DOI: 10.1111/j.1750-3841.2010.01899.x.
- Daud, N. H.; Aung, C. S.; Hewavitharana, A. K.; Wilkinson, A. S.; Pierson, J.; Roberts-Thomson, S. J.; Shaw, P. N.; Monteith, G. R.; Gidley, M. J.; Parat, M. Mango Extracts and the Mango Component Mangiferin Promote Endothelial Cell Migration. J. Agric. Food Chem. 2010, 58, 5181–5186. DOI: 10.1021/jf100249s.
- Robles-Sanchez, M.; Astiazaran-Garcia, H.; Martin-Belloso, O.; Gorinstein, S.; Alvarez-Parrilla, E.; de la Rosa, L. A.; Yepiz-Plascencia, G.; Gonzalez-Aguilar, G. A. Influence of Whole and Fresh-Cut Mango Intake on Plasma Lipids and Antioxidant Capacity of Healthy Adults. Food Res. Int. 2011, 44, 1386–1391. DOI: 10.1016/j.foodres.2011.01.052.
- Lucas, E. A.; Li, W.; Peterson, S. K.; Brown, A.; Kuvibidila, S.; Perkins-Veazie, P.; Clarke, S. L.; Smith, B. J. Mango Modulates Body Fat and Plasma Glucose and Lipids in Mice Fed a High Fat Diet. Br. J. Nutr. 2011, 106, 1495–1505. DOI: 10.1017/S0007114511002066.
- Evans, S. F.; Meister, M.; Mahmood, M.; Eldoumi, H.; Peterson, S.; Perkins-Veazie, P.; Clarke, S. L.; Payton, M.; Smith, B. J.; Lucas, E. A. Mango Supplementation Improves Blood Glucose in Obese Individuals. Nutr. Metab. Insights. 2014, 7, 77–84.
- CSIR. The Wealth of India; A Dictionary of Indian Raw Materials and Industrial Products; Council of Scientific and Industrial Research: New Delhi, 1962; pp 265–285.
- Kind, T.; Wohlgemuth, G.; Lee, D. Y.; Palazoglu, M.; Shahbaz, S.; Fiehn, O. FiehnLib: Mass Spectral and Retention Index Libraries for Metabolomics Based on Quadrupole and Time of Flight Gas Chromatography /Mass Spectrometry. Anal. Chem. 2009, 81, 10038–10048. DOI: 10.1021/ac9019522.
- Das, S.; Dutta, M.; Chaudhury, K.; De, B. Metabolomic and Chemometric Study of Achras Sapota L. Fruit Extracts for Identification of Metabolites Contributing to the Inhibition of Α-Amylase and Α-Glucosidase. Eur. Food Res. Technol. 2016, 242, 733–743. DOI: 10.1007/s00217-015-2581-0.
- Schieber, A.; Berardini, N.; Carle, R. Identification of Flavones and Xanthone Glycosides from Mango (Mangifera Indica L. Cv. Tommy Atkins) Peels by High Performance Liquid Chromatography-Electrospray Ionization Mass Spectroscopy. J. Agric. Food Chem. 2003, 51, 5006–5011. DOI: 10.1021/jf030218f.
- McDougall, G. J.; Kulkarni, N. N.; Stewart, D. Berry Polyphenols Inhibit Pancreatic Lipase Activity in Vitro. Food Chem. 2009, 115, 193–199. DOI: 10.1016/j.foodchem.2008.11.093.
- Sadasivam, S.; Manikam, A. Biochemical Methods; Wiley Eastern Limited: India, 1992.
- Kim, D.; Jeong, S. W.; Lee, C. Y. Antioxidant Capacity of Phenolic Phytochemicals from Various Cultivars of Plums. Food Chem. 2003, 81, 321–326. DOI: 10.1016/S0308-8146(02)00423-5.
- Dutta, M.; Joshi, M.; Srivastava, S.; Lodh, I.; Chakravarty, B.; Chaudhury, K. A Metabonomics Approach as A Means for Identification of Potential Biomarkers for Early Diagnosis of Endometriosis. Mol. Biosyst. 2012, 8, 3281–3287. DOI: 10.1039/c2mb25353d.
- Itoh, K.; Murata, K.; Nakagaki, Y.; Shimizu, A.; Takata, Y.; Shimizu, K.; Tetsuya, M.; Kajiyama, S.; Fumura, M.; Lijima, M.; Matsuda, H. A Pancreatic Lipase Inhibitory Activity by Mango (Mangifera Indica) Leaf Methanolic Extract. J. Plant Stud. 2016, 5, 72–78. DOI: 10.5539/jps.v5n2p72.