ABSTRACT
Morus alba L. (mulberry) is one of the most important medicine and food crops in China and other developing countries in Asia due to its large biomass (twigs) and useful by-products. Additionally, its twigs are an excellent green resource for bioactive compounds that are resistant to ultraviolet (UV) radiation and that can whiten skin and scavenge free radicals, and are of potential interest to the cosmetics and health food industries. In this study, we evaluated the ability of Morus alba L. twig hydroethanol extract and isolated compounds to protect DNA against UV damage. Agarose gel electrophoresis showed that a hydroethanol extract and six different polyphenol monomers (oxyresveratrol, resveratrol, mulberroside A, rutin, quercetin, and morin) present in the extract protected pUC19 plasmid DNA from UV damage. The optimal concentrations of polyphenols and Morus alba L. twig extract for DNA protection were 0.500 mg/mL and 1.00 mg/mL, respectively. These compounds may have potential as skin-care products to prevent UV-induced damage to the skin. Additionally, activity contribution degree assessment revealed other Morus alba L. substances possessing significant DNA protection activity that are worthy of further investigation.
Introduction
DNA, the genetic material of all organisms, can undergo irreversible damage due to mutations occurring over time that, if not promptly repaired, can lead to ageing, cancer, and various other diseases. [Citation1–Citation3] Numerous causes of DNA damage are known, including ultraviolet (UV) radiation, reactive oxygen species (ROS), and hydroxyl radicals (.OH), all of which can destroy the structure of duplex DNA. [Citation4,Citation5]
UV radiation is among the most harmful exogenous factors for human skin due to its ability to induce free radical/ROS production. [Citation6–Citation8] Abundant free radicals/ROS in the skin mainly result from solar UV and cause long-term radiation of the skin. [Citation9] Free radicals/ROS cause immense damage to DNA[Citation10,Citation11], resulting in various biological effects on the skin, such as generation of the pigment AD cool-headed, skin ageing, skin wrinkling, immune suppression, and even skin cancer[Citation12], which is a major health hazard worldwide. Protecting DNA from damage caused by free radicals/ROS can prevent skin cancer and other diseases caused by UV exposure.
DNA damage can also be reversed in some cases. [Citation13–Citation16] In addition to intrinsic damage repair mechanisms, other protection mechanisms may also be operable. For example, DNA damage can be prevented by certain molecules, especially natural antioxidant compounds such as flavonoids, polyphenols, and carotenoids, which exist in fruits, vegetables, and medicinal plants.[Citation17–Citation22] Screening for highly effective, non-toxic, antioxidant compounds that protect against DNA damage is now an active area of biomedical research[Citation23], and interest in this area is growing. Various natural antioxidants have been successfully isolated from plants, such as rutin, resveratrol, and various flavonoids. [Citation24,Citation25]
Morus alba L. twigs contain an interesting yet complex mixture of bioactive substances including phenolic compounds, alkaloids, and polysaccharides. Recent discoveries in the field of Morus alba L. phytochemicals revealed that Morus alba L. twig extracts contain polyphenols, which are major effective antioxidants.[Citation26–Citation29] Meanwhile, monomer polyphenols including oxyresveratrol, resveratrol[Citation30,Citation31], mulberroside, rutin[Citation32], quercetin[Citation33], and morin,[Citation34] which exist in Morus alba L. twig are more extensive, have high antioxidant activity, and are effective scavengers of free radicals. Six polyphenols, in particular, including rutin, quercetin, and morin, have been isolated and shown to possess high free radical scavenging activity[Citation35], and resveratrol and rutin can protect against DNA damage.[Citation25,Citation36,Citation37]
In the present study, we quantitatively evaluated the in vitro DNA protection activity of Morus alba L. twig extract and the six isolated polyphenols (oxyresveratrol, resveratrol, mulberroside A, rutin, quercetin, and morin; ) using Image Lab. Furthermore, we assessed the relative contribution of individual polyphenols using high-pressure liquid chromatography (HPLC), and explored the mechanism by which they protect against UV-induced DNA damage. The findings provide a theoretical basis for the further development and utilisation of Morus alba L. twig.
Materials and methods
Materials and reagents
Plant material
Morus alba L. twigs were provided by the Sericultural Science and Technology Research Institute of Chongqing, China, and they were dried, crushed, and refrigerated for later use.
Reagents
Agarose H, TAE, 6× loading buffer, ampicillin, and rutin (purity 99%) were purchased from Shanghai Sangon Biological Engineering Co. (Shanghai, China). Oxyresveratrol, resveratrol, mulberroside A, and quercetin (purity ≥98%) were obtained from Chengdu Mann Biological Technology Co. (Sichuan, China). Morin (purity >90%) was acquired from TCI (Shanghai, China). The plasmid extraction kit (PD1511) was from Biomiga, Inc (Shanghai, China). K2HPO4, KH2PO4, NaCl, methanol, ethanol, tryptone, yeast powder, methanol, and acetonitrile (HPLC grade) were obtained from Chongqing Titanium New Chemical Co. (Chongqing, China)
Experiments and methods
Sample preparation
Morus alba L. twig powder (50 g) was sonicated with 1 L of 75% ethanol for 30 min using a microwave (350 W) at low temperature and low pressure, and then extracted three times, as described in our previous study. [Citation36] Extracts were enriched and filtered under vacuum, concentrated to dryness, and stored at room temperature (RT) in the dark. Concentration gradients were prepared ranging from 0.0313 to 2.00 mg/mL and stored at 4°C for subsequent experiments.
Plasmid DNA extraction
Replication and amplification of plasmid DNA were performed in Escherichia coli. Fresh E. coli cultures (100 μL) were added to 200 mL lysogeny broth (LB) containing ampicillin (100 μg/mL) and cultured for 14–16 h at 37°C with shaking at 220 rpm. Cells were collected by centrifugation, and plasmid DNA was extracted using a plasmid DNA extraction kit (PD1511) (Biomiga, Inc. Shanghai, China) and stored at 4°C until needed.
Evaluation of DNA damage protection activity
The ability of compounds to protect pUC19 plasmid DNA against strand breakage was investigated as described previously,[Citation15,Citation38,Citation39] with some modifications. Plasmid DNA was oxidised by UV treatment in the presence of polyphenol monomers or Morus alba L. twig extract and monitored by 1% agarose gel electrophoresis.[Citation40] In brief, experiments were performed in a volume of 10 μL in a microfuge tube containing 3 μL pUC19 plasmid DNA (50 ng/μL), 2 μL phosphate buffered potassium (PBP, pH 7.4), and 5 μL of different concentrations of a sample solution (0.0313–2.00 mg/mL). Reactions were initiated by UV irradiation and continued for 30 min using a UV transilluminator (ZF-90, Shanghai Gu Cun Electro-optical Instrument Plant, Shanghai, China) with an intensity of 8000 μW/cm2 at a wavelength of 310 nm at RT. After irradiation, reaction mixtures (4 μL) were mixed with 6× gel loading dye and separated on a 1% agarose gel. Untreated pUC19 plasmid DNA was used as a control, along with partially treated plasmid. Gels were stained with ethidium bromide and photographed using a gel documentation system (Bio-Rad Laboratories, Inc. USA). [Citation41,Citation42]
Analysis of polyphenol monomers by HPLC
Extracts were analysed using HPLC, and polyphenol monomers were detected as described previously[Citation38] using a reversed-phase Inert Sustain C18 column (250 mm x 4.6 mm, 5 μm particle size, SHIMADZU, Tokyo, Japan) with a sample volume of 10 μL and a column temperature of 30°C.
All six polyphenol monomers were isolated using the gradient elution method, and standards were used for five of them (oxyresveratrol, resveratrol, mulberroside A, rutin, and quercetin) via hybrid detection with a mobile phase of acetonitrile (A) and 0.1% phosphate buffer (B). Oxyresveratrol, resveratrol, and mulberroside A were eluted as follows: 0–55 min, A:B (v/v) = 11:89–24:76; 55–60 min, A:B = 11:89.; flow rate = 0.8 mL/min, running time = 60 min, detection wavelength = 330 nm. Rutin and quercetin were eluted as follows: 0–35 min, A:B = 20:80–32:68; 35–40 min, A:B = 20:80; flow rate = 1 mL/min, running time = 40 min, detection wavelength = 254 nm. Morin was eluted with a mobile phase of methanol (C) and 0.1% phosphate buffer (B) as follows: 0–48 min, C:B = 45:55–55:45; 48–50 min, C:B = 45:55; flow rate = 1 mL/min, running time = 50 min, detection wavelength = 360 nm. The proportion of each of the six polyphenol monomers in the Morus alba L. twig extract was calculated using the standard curve.
Analysis of the contribution rate of polyphenol monomers
A mixture of the six polyphenol monomer standards was prepared that corresponded to the relative amounts in the Morus alba L. twig extract, and the ability of this mixture to protect against UV-induced DNA damage was compared with that of the Morus alba L. twig extract.
Statistic and analysis
All assays were carried out in triplicate for all the experiments. The results are expressed as mean and standard deviation values (mean ± S.D.). All values obtained were compared using analysis of variance (ANOVA), and differences at p < 0.01 were considered statistically significant, which were analysed with SPSS (version 17.0). The DNA bands were analysed with Image Lab 3.0 (Bio-Rad Laboratories, Inc. USA).
Results
DNA damage protection potential
Plasmid DNA assumed an annular helical structure (supercoiled DNA, ScDNA) (; ScDNA), but following UV treatment it fractured in multiple places, resulting in molecular rotation and elimination of tension to form open circular DNA (OcDNA) and linear DNA (LnDNA) (; OcDNA, LnDNA). During the extraction process, it could presumably induce similar damage to OcDNA under anthropogenic factors. As can be seen in , untreated pUC19 DNA ran as two bands (ScDNA and OcDNA) on the agarose gel. However, it was damaged differently from LnDNA, which ran between OcDNA and ScDNA after exposure to UV irradiation. The six polyphenol monomers were tested for their ability to protect pUC19 plasmid DNA against UV-induced damage at various concentrations (0.0313–2.00 mg/mL). The results are shown in . At different concentrations, the degree of protection of polyphenol monomers on ScDNA differed. Polyphenol monomers had no evident effect on protecting plasmid DNA at lower concentrations, and most of the ScDNA was damaged similar to that of LnDNA. Concentrations of polyphenols between 0.125 and 1.00 mg/mL protected against DNA damage to varying degrees. At a concentration of 0.125 mg/mL, oxyresveratrol, resveratrol, and rutin exerted an obvious protective effect, and the capacity was ordered oxyresveratrol ≥resveratrol >rutin. However, compared with oxyresveratrol, resveratrol, and rutin, the ability of mulberroside A, morin, and quercetin to protect DNA was weaker. A concentration of polyphenols between 0.250 and 0.500 mg/mL protected against DNA damage; the effect increased with increasing concentration, and less of the ScDNA was damaged compared with OcDNA. A concentration of polyphenols between 0.500 and 1.00 mg/mL showed maximum ability to protect DNA.
Figure 2. Agarose gel assays of the DNA protection activity of the six polyphenol monomers. A. resveratrol, B. ruit, C. oxyresveratrol, D. mulberroside A, E. quercetin, F. morin. PBP: phosphate buffered potassium. 1. DNA (3 μL) + PBP (2 μL) + ddH2O (5 μL); 2. DNA (3 μL) + PBP (2 μL) + solvent (5 μL); 3. DNA (3 μL) + PBP (2 μL) + ddH2O (5 μL) + UV (30 min); 4. DNA (3 μL) +PBP (2 μL) + solvent (5 μL) + UV (30 min); 5–11: DNA (3 μL) + PBP (2 μL) + different concentrations of sample solution (5 μL 0.03125 mg/mL to 2.00000 mg/mL) + UV (30 min).
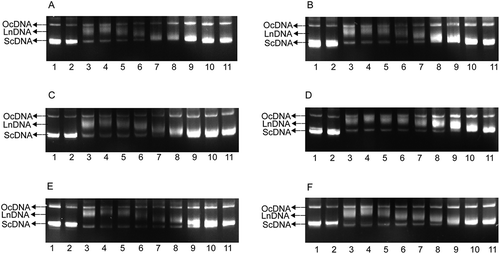
To further analyse their ability to protect ScDNA, equivalent amounts of ScDNA were incubated with the polyphenols, exposed to UV, and analysed with Image Lab. The protection rate (%) was obtained using the following formula: ScDNA strip percentage of treatment/ScDNA strip percentage of solvent control) × 100. The results revealed that the optimum concentration of polyphenols for protecting ScDNA was 0.500 mg/mL in all cases (). The protection rates of polyphenols were ordered morin (97.60%) >mulberroside A (97.54%) >oxyresveratrol (96.22%) >quercetin (93.51%) >rutin (93.34%) >resveratrol (91.91%).
Figure 3. Analysis of ScDNA at the optimum polyphenol concentrations. a Values expressed are means ± S.D. of three parallel measurements. Data in the same column marked with different superscript symbols indicate significant differences (compared with the blank control group of ScDNA; p < 0.01). Re: Resveratrol; Ox: Oxyresveratrol; Ru: Ruit; Mu: Mulberroside A; Qu: Quercetin; Mo: Morin.
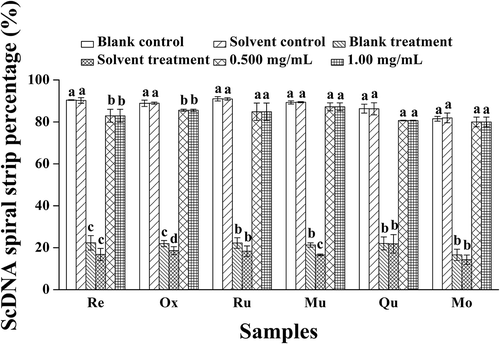
Further analysis of the activity of each polyphenol at different concentrations was performed using OcDNA protection assays (). The results showed that UV irradiation did not induce obvious damage to OcDNA when polyphenols were present compared with solvent controls, but ScDNA was clearly damaged to some degree. When the concentration was lower than 0.500 mg/mL, breaking of the superhelical structure of ScDNA was apparent, resulting in the appearance of LnDNA and an increase in OcDNA, consistent with the research of Jeong et al.,[Citation43] who studied the effects of extracts from pine needles against oxidative DNA damage by hydroxyl radicals. Meanwhile, at the optimal concentration of polyphenol monomers, the amount of OcDNA was lower than that in the solvent treatment, but greater than that in the solvent control. Therefore, it could be concluded that the ability of polyphenol monomers to protect ScDNA gradually enhanced with increasing concentration.
Figure 4. Analysis of OcDNA bands for each experimental group. a Values expressed are means ± S.D. of three parallel measurements. Data in the same column marked with different superscript symbols indicate significant differences (compared with the highest proportion of OcDNA; p < 0.01).Re: Resveratrol; Ox: Oxyresveratrol; Ru: Ruit; Mu: Mulberroside; Qu: Quercetin; Mo: Morin.
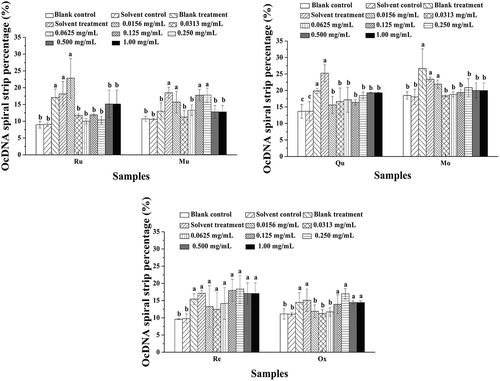
Analysis of the polyphenol monomer content in Morus alba L. twig extract
Standard curve equations based on linear regression resulted in plots for resveratrol, oxyresveratrol, mulberroside A, and morin of Y = 7E+07x – 7894.8 (R2 = 0.9998), Y = 7E+07x – 84136 (R2 = 0.9998), Y = 2E+07x + 5551 (R2 = 0.9995), and Y = 2E+08x – 392504 (R2 = 0.9999), respectively, all with a linear range of 0–0.333 mg/mL. Standard curve equations based on linear regression for rutin and quercetin were Y = 2E+07x – 5506.7 (R2 = 0.9999) and Y = 4E+07x + 29469 (R2 = 0.9999), respectively, with a linear range of 0–0.500 mg/mL.
Regression equations were used to calculate the amount of each of the six polyphenol monomers in the Morus alba L. twig extract (). The most abundant polyphenol monomer was mulberroside A (35.4 mg/g), followed by oxyresveratrol (13.6 mg/g), morin (2.24 mg/g), rutin (2.19 mg/g), quercetin (0.350 mg/g), and resveratrol (0.340 mg/g). HPLC chromatograms of the six polyphenol monomers are shown in .
Table 1. The amount of the six polyphenol monomers in Morus alba L. twig extract.a
Figure 5. HPLC chromatograms of the six polyphenol monomers. A. HPLC chromatograms of oxyresveratrol, resveratrol, and mulberroside A standards. a. HPLC chromatograms of oxyresveratrol, resveratrol, and mulberroside A in Morus alba L. twig extract. B. HPLC chromatograms of rutin and quercetin standards. b. HPLC chromatograms of rutin and quercetin in the Morus alba L. twig extract. C. HPLC chromatograms of the morin standard. c. HPLC chromatograms of morin in the Morus alba L. twig extract.
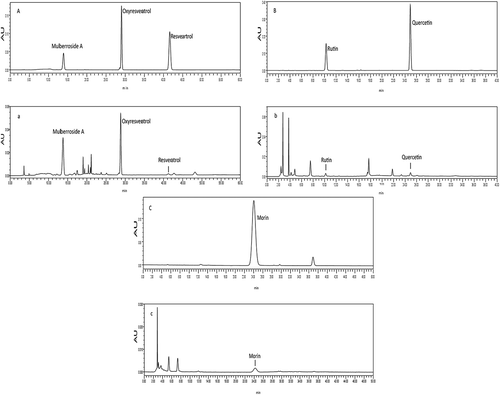
Analysis of polyphenol monomer contributions
The contribution of the six polyphenol monomers in the Morus alba L. twig extract on protection against DNA damage is shown in –. Morus alba L. twig extract did not exert a significant protective effect on ScDNA at low concentrations, and most of the ScDNA was damaged, as evidenced by the presence of LnDNA and OcDNA forms. At the maximum concentration of 1.00 mg/mL, protection of ScDNA was evident, but from the position of DNA bands in , it can be concluded that ScDNA and OcDNA were mixed together, with no clear boundaries between them; hence, Image Lab software could not be used for analysis. The ability of the mixture of the six polyphenol monomers to protect ScDNA was gradually enhanced as the concentration was increased from 0.500 mg/mL to 1.00 mg/mL, but most ScDNA was damaged even at the highest concentration.
Figure 6. Six standard hybrid system analyses of protection against DNA damage. A. Morus alba L. twig extract; B. mixture of six polyphenol monomers. PBP: phosphate buffered potassium. 1. DNA (3 μL) + PBP (2 μL) + ddH2O (5 μL); 2. DNA (3 μL) + PBP (2 μL) + solvent (5 μL); 3. DNA (3 μL) + PBP (2 μL) + ddH2O (5 μL) + UV (30 min); 4. DNA (3 μL) +PBP (2 μL) + solvent (5 μL) + UV (30 min); A: 5–11: DNA (3 μL) + PBP (2 μL) + different concentrations of Morus alba L. twig extract (5 μL 0.0313 mg/mL to 2.00 mg/mL) + UV (30 min); B: 5–11: DNA (3 μL) + PBP (2 μL) + the mixture of six polyphenol monomers of different concentration of Morus alba L. twig extract (5 μL 0.0313 mg/mL to 2.00 mg/mL) + UV (30 min).

Figure 7. OcDNA band percentage analysis in each experimental group. a Values expressed are means ± S.D. of three parallel measurements. Data in the same column marked with different superscript symbols indicate significant differences (compared with the highest proportion of OcDNA; p < 0.01).
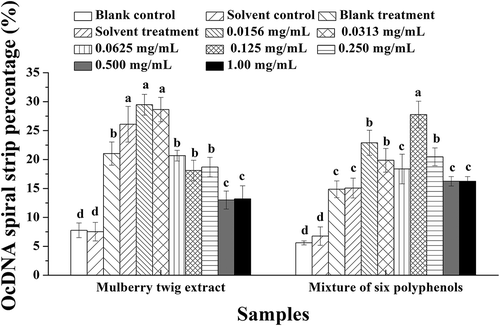
Figure 8. ScDNA band percentage analysis in the presence of optimal polyphenol monomer concentrations. a Values expressed are means ± S.D. of three parallel measurements. Data in the same column marked with different superscript symbols indicate significant differences (compared with the ScDNA of blank control group; p < 0.01).
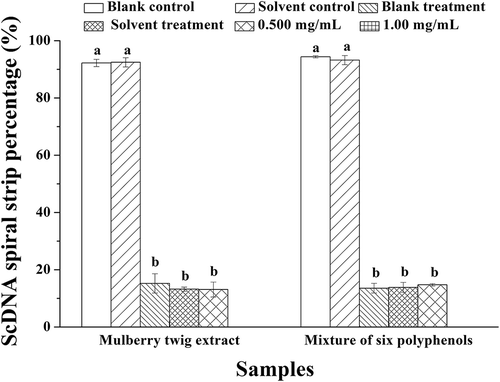
As can be seen from the results presented above, the optimal concentration of Morus alba L. twig extract for protecting against DNA damage was 1.00 mg/mL (in the measured concentration range). Additionally, as is evident in and , UV irradiation did not obviously damage OcDNA, but damage to ScDNA was significant. The results also indicate that other substances in Morus alba L. twig extract in addition to the polyphenol monomers also protect against UV-induced DNA damage. We intend to determine the nature of these compounds in a future work.
Discussion
It is known that UV irradiation for a long time can cause large amounts of DNA damage in the body, leading to gene coding and protein expression errors and metabolic disorders that cause pathological outcomes such as cancer. There are two main forms of DNA damage caused by UV. In the first one, DNA can directly absorb energy from UV irradiation that, if it exceeds the capacity of DNA, can result in the dimerisation of two adjacent pyrimidine bases and destroy the original native DNA structure; in the other type, free radicals/ROS such as hydroxyl free radicals, superoxide anions, oxygen free radicals, and their active precursor singlet oxygen, hydrogen peroxide and ozone, which are generated during oxidative respiration and exposure to UV irradiation, are strongly oxidising and can attack the DNA skeleton on the DNA of deoxyribose by generating substrate free radicals or hydrogenation reaction, and the secondary reaction of free radicals can cause serious damage to the structure of DNA. [Citation6–Citation8,Citation31,Citation43]
Sunlight can produce a strong UV radiation, and it can be difficult for humans to avoid UV radiation, especially for people who work outdoors for extended periods, and consequently the DNA in their skin is under threat from UV-induced damage. Some of the sunscreen products contain chemical compounds that can cause skin allergies and other adverse reactions, and prolonged usage can lead to skin diseases. Therefore, the search for and development of natural products for DNA protection is of medical significance. Oxyresveratrol, resveratrol, mulberroside A, rutin, quercetin, morin, and other compounds are natural polyphenols present in Chinese herbs, fruits, and vegetables that have a strong ability to scavenge free radicals. [Citation34,Citation35] Our current results showed that the active substances and Morus alba L. twig extract display good protection against DNA damage, which might be attributed to their strong free radical scavenging activity. These compounds belong to flavonoids (rutin, quercetin, morin) and stilbenes (oxyresveratrol, resveratrol, mulberroside A). The structure of these three stilbene compounds is very similar, and their activity is also similar. Some studies have indicated that the protective effect of oxyresveratrol prevents riboflavin photosensitive oxygen free radical-induced DNA damage via antioxidant activity[Citation44], and the DNA protective activity is greater than that of the well-known antioxidants trolox and ascorbic acid. Studies by Hu et al.[Citation45] showed that oxyresveratrol can inhibit the production of ROS and 8-hydroxy-2ʹ-deoxyguanosine (8-OHdG) in skin cells by UV irradiation or hydrogen peroxide treatment. It also indicated that oxyresveratrol can enhance the repair ability of 8-OHdG and cyclobutane pyrimidine dimers, increase the expression of the p53 gene to protect the integrity of human epidermal cell DNA, and reduce the occurrence of cancer. Meanwhile, some studies showed that resveratrol can inhibit hydrogen peroxide-induced oxidative DNA damage in human lymphocytes. [Citation46] Burkhardt et al.[Citation47] and López-Burillo et al.[Citation48] reported that resveratrol could inhibit DNA damage in a concentration-dependent manner by reducing the content of 8-OHdG in calf thymus to reduce the incidence rate of cancer. Our current results also showed that these three stilbene compounds have strong DNA protection activity. Therefore, it can be speculated that they could be used in skin-care products to prevent skin cancer. Meanwhile, other studies also showed that quercetin and rutin can inhibit hydrogen peroxide-induced DNA damage in Caco-2 and Hep G2 cells through anti-oxidation and free radical scavenging activities, and there was no significant adverse effect on Caco-2 and Hep G2 cell activities. [Citation49,Citation50] Moreover, other researchers have reported that these compounds and Morus alba L. twig extract possess strong free radical scavenging and antioxidant activities[Citation34,Citation35] due to their phenolic hydroxyl groups that are susceptible to oxidation. Thus, we can conclude that several polyphenols protect against UV irradiation and hydrogen peroxide-induced DNA damage mainly through their antioxidant and free radical scavenging activities. In our experiments, we found that all of these compounds share a characteristic absorbance in the UV–Vis spectrum. Several studies have demonstrated that vitamin C (Vc) can protect DNA from damage induced by UV irradiation by scavenging hydroxyl free radicals and absorbing the energy associated with UV light. [Citation51] Therefore, the mechanism through which Morus alba L. twig polyphenols protected plasmid DNA against UV radiation in the present work may also involve antioxidant and free radical scavenging activities as well as absorbing the energy from UV radiation. [Citation52]
Furthermore, resveratrol can also help repair UV-induced DNA damage by up-regulating inducible Nitric Oxide Synthase (iNOS) gene expression and stimulating the secretion of NO in skin cutin cells and other cells. [Citation37,Citation53] Thus, oxyresveratrol, mulberroside A, rutin, quercetin, and morin in Morus alba L. twig extract, especially oxyresveratrol and mulberroside A, which are derivatives of resveratrol with similar structures and biological activities, may also possess DNA repair activity. This is certainly worthy of further investigation, as are the specific structures and the mechanism of these polyphenols.
Conclusion
These results revealed that Morus alba L. twig extract and the polyphenol monomer secondary metabolites within it possess potent DNA protection activity. These compounds are potentially useful for natural skin-care products to prevent UV-induced skin damage. They may also be useful as natural food additives and in health-care products to prevent diseases caused by .OH/ROS and other radicals. Furthermore, other substances in Morus alba L. twig extract also possess DNA protection activity and are worthy of further study.
Additional information
Funding
References
- Ramos, A. A.; Azqueta, A.; Pereira-Wilson, C.; Collins, A. R. Polyphenolic Compounds from Salvia Species Protect Cellular DNA from Oxidation and Stimulate DNA Repair in Cultured Human Cells. Journal of Agricultural and Food Chemistry 2010, 58, 7465–7471. doi:10.1021/jf100082p
- Hsieh, C. L.; Yen, G. C.; Chen, H. Y. Antioxidant Activities of Phenolic Acids on Ultraviolet Radiation-Induced Erythrocyte and Low Density Lipoprotein Oxidation. Journal of Agricultural and Food Chemistry 2005, 53, 6151–6155.
- Shi, Y. W.; Wang, C. P.; Wang, X.; Zhang, Y. L.; Liu, L.; Wang, R. W.; Ye, J. F.; Hu, L. S.; Kong, L. D. Uricosuric and Nephroprotective Properties of Ramulus Mori Ethanol Extract in Hyperuricemic Mice. Journal of Ethnopharmacology 2012, 143, 896–904.
- Dizdaroglu, M.; Jaruga, P.; Birincioglu, M.; Rodriguez, H. Free Radical-Induced Damage to DNA: Mechanisms and Measurement 1, 2. Free Radical Biology and Medicine 2002, 32, 1102–1115.
- Parman, T.; Wiley, M. J.; Wells, P. G. Free Radical-Mediated Oxidative DNA Damage in the Mechanism of Thalidomide Teratogenicity. Nature Medicine 1999, 5, 582–585.
- Haywood, R.; Rogge, F.; Lee, M. Protein, Lipid, and DNA Radicals to Measure Skin UVA Damage and Modulation by Melanin. Free Radical Biology & Medicine 2008, 44, 990–1000.
- Petruk, G.; Raiola, A.; Del Giudice, R.; Barone, A.; Frusciante, L.; Rigano, M. M.; Monti, D. M. An Ascorbic Acid-Enriched Tomato Genotype to Fight UVA-induced Oxidative Stress in Normal Human Keratinocytes. Journal of Photochemistry and Photobiology B: Biology 2016, 163, 284–289.
- Douki, T.; Reynaud-Angelin, A.; Cadet, J.; Sage, E. Bipyrimidine Photoproducts Rather than Oxidative Lesions are the Main Type of DNA Damage Involved in the Genotoxic Effect of Solar UVA Radiation. Biochemistry 2003, 42, 9221–9226.
- Zastrow, L.; Groth, N.; Klein, F.; Kockott, D.; Lademann, J.; Renneberg, R.; Ferrero, L. The Missing Link - Light-Induced (280-1,600 Nm) Free Radical Formation in Human Skin. Skin Pharmacology and Physiology 2009, 22, 31–44.
- Jackson, M. J.;. Free Radicals in Skin and Muscle: Damaging Agents or Signals for Adaptation? Proceedings of the Nutrition Society 1999, 58, 673–676. doi:10.1017/S0029665199000877
- Jung, K.; Seifert, M.; Herrling, T.; Fuchs, J. UV-generated Free Radicals (FR) in Skin: Their Prevention by Sunscreens and Their Induction by Self-Tanning Agents. Spectrochimica Acta Part A: Molecular and Biomolecular Spectroscopy 2008, 69, 1423–1428. doi:10.1016/j.saa.2007.09.029
- Li, X. K.; Yao, C. C.; Xu, H.; Zheng, Q.; Huang, Y. D.; Liu, S. Z. Study of Effect of UV Ray on Keratinocyte and Its Protection. China Surfactant Detergent & Cosmetics 2002, 32, 30–32.
- Davidson, P. G.; Touger-Decker, R. Chemopreventive Role of Fruits and Vegetables in Oropharyngeal Cancer. Nutrition in Clinical Practice 2009, 24, 250–260.
- Romeu, M.; Rubio, L.; Sanchez-Martos, V.; Castaner, O.; De La Torre, R.; Valls, R. M.; Ras, R.; Pedret, A.; Catalan, U.; De Las Hazas, M. D. L.; Motilva, M. J.; Fito, M.; Sola, R.; Giralt, M., Virgin Olive Oil Enriched with Its Own Phenols or Complemented with Thyme Phenols Improves DNA Protection against Oxidation and Antioxidant Enzyme Activity in Hyperlipidemic Subjects. Journal of Agricultural and Food Chemistry 2016, 64, 1879–1888.
- De Camargo, A. C.; Regitano-d’Arce, M. A. B.; Biasoto, A. C. T.; Shahidi, F. Low Molecular Weight Phenolics of Grape Juice and Winemaking Byproducts: Antioxidant Activities and Inhibition of Oxidation of Human Low-Density Lipoprotein Cholesterol and DNA Strand Breakage. Journal of Agricultural and Food Chemistry 2014, 62, 12159–12171.
- Salem, M. A. I.; Marzouk, M. I.; El-Kazak, A. M. Synthesis and Characterization of Some New Coumarins with in Vitro Antitumor and Antioxidant Activity and High Protective Effects against DNA Damage. Molecules 2016, 21, 249.
- Fang, R.-M.; Tao, X.-Q.; Huang, M.-Y. Study on the anti-Oxidation and Protective Effect of Red Ginseng, Shengshai Ginseng, American Ginseng on DNA Damage. Journal-Sichuan University Natural Science Edition 2003, 40, 1157–1160.
- Su, W.; Tang, S. T.; Xie, C. Z.; Mu, Y. C.; Li, Z. X.; Yang, X. H.; Qiu, S. Y. Antioxidant and DNA Damage Protection Activities of Duck Gizzard Peptides by Chemiluminescence Method. International Journal of Food Properties 2016, 19, 760–767.
- Boubaker, J.; Skandrani, I.; Bouhlel, I.; Ben Sghaier, M.; Neffati, A.; Ghedira, K.; Chekir-Ghedira, L. Mutagenic, Antimutagenic and Antioxidant Potency of Leaf Extracts from Nitraria Retusa. Food and Chemical Toxicology 2010, 48, 2283–2290.
- Chan, S.; Li, S.; Kwok, C.; Benzie, I.; Szeto, Y.; Guo, D. J.; He, X.; Yu, P. Antioxidant Activity of Chinese Medicinal Herbs. Pharmaceutical Biology 2008, 46, 587–595.
- Qu, Y.; Li, C. X.; Zhang, C.; Zeng, R.; Fu, C. M. Optimization of Infrared-Assisted Extraction of Bletilla Striata Polysaccharides Based on Response Surface Methodology and Their Antioxidant Activities. Carbohydrate Polymers 2016, 148, 345–353.
- Cao, W.; Chen, W. J.; Zheng, X. H.; Zheng, J. B. Modified Method to Evaluate the Protection of the Antioxidants against Hydroxyl Radical-Mediated DNA Damage. Acta Nutrimenta Sinica 2008, 30, 74–77.
- Ikeoka, S.; Nakahara, T.; Iwahashi, H.; Mizushina, Y. The Establishment of an Assay to Measure DNA Polymerase-Catalyzed Repair of UVB-induced DNA Damage in Skin Cells and Screening of DNA Polymerase Enhancers from Medicinal Plants. International Journal of Molecular Sciences 2016, 17, 667.
- Kootstra, A.;. Protection from UVB-induced DNA-damage by Flavonoids. Plant Molecular Biology 1994, 26, 771–774.
- Webster, R. P.; Gawde, M. D.; Bhattacharya, R. K. Protective Effect of Rutin, a Flavonol Glycoside, on the Carcinogen-Induced DNA Damage and Repair Enzymes in Rats. Cancer Letters 1996, 109, 185–191.
- Chang, L. W.; Juang, L. J.; Wang, B. S.; Wang, M. Y.; Tai, H. M.; Hung, W. J.; Chen, Y. J.; Huang, M. H. Antioxidant and Antityrosinase Activity of Mulberry (Morus Alba L.) Twigs and Root Bark. Food and Chemical Toxicology 2011, 49, 785–790.
- Park, H. M.; Hong, J.-H. Effect of Extraction Methods on Antioxidant Activities of Mori Ramulus. Journal of the Korean Society of Food Science and Nutrition 2014, 43, 1709–1715.
- Zhang, Z.; Shi, L. Detection of Antioxidant Active Compounds in Mori Ramulus by HPLC-MS-DPPH. China Journal of Chinese Materia Medica 2012, 37, 800–802.
- Zhang, Z. F.; Jin, J.; Shi, L. G. Antioxidant Properties of Ethanolic Extract from Ramulus Mori (Sangzhi). Food Science and Technology International 2009, 15, 435–444.
- Wang, Y. C.; Wu, C.; Chen, H.; Zhang, Y.; Xu, L.; Huang, X. Z. Antioxidant Activities of Resveratrol, Oxyresveratrol, Esveratrol, Mulberroside A from Cortex Mori. Food Science 2011, 15, 030.
- Zhang, Z.-S.; He, W.; Liu, T. T.; Shi, S.; Li, S. Antioxidant Activity of Resveratrol in Vitro. Food Science 2012, 11, 055.
- Chua, L. S.;. A Review on Plant-Based Rutin Extraction Methods and Its Pharmacological Activities. Journal of Ethnopharmacology 2013, 150, 805–817.
- Baghel, S. S.; Shrivastava, N.; Baghel, R. S.; Agrawal, P.; Rajput, S. A Review of Quercetin: Antioxidant and Anticancer Properties. World Journal Pharmaceutical Pharmaceut Sciences 2012, 1, 146–160.
- Yang, J. Y.; Lee, H. S. Evaluation of Antioxidant and Antibacterial Activities of Morin Isolated from Mulberry Fruits (Morus Alba L.). Journal of the Korean Society for Applied Biological Chemistry 2012, 55, 485–489.
- Zhou, L.;. Studies on Extraction Purification and Antioxidant Activity of Flavonoids from Mulberry Branch; Southwest University, 2011.
- Liu, C.; Xiang, W.; Yu, Y.; Shi, Z.-Q.; Huang, X.-Z.; Xu, L. Comparative Analysis of 1-Deoxynojirimycin Contribution Degree to α-glucosidase Inhibitory Activity and Physiological Distribution in Morus Alba L. Industrial Crops and Products 2015, 70, 309–315.
- Chen, M. L.;. The Damage Mechanism of UVA on Human Fibroblast and Keratinocyte and the Photoprotection Role of Resveratrol from UVA Induced Damage; Central South University, 2008.
- Asikin, Y.; Takahashi, M.; Mizu, M.; Takara, K.; Oku, H.; Wada, K. DNA Damage Protection against Free Radicals of Two Antioxidant Neolignan Glucosides from Sugarcane Molasses. Journal of the Science of Food and Agriculture 2016, 96, 1209–1215.
- Sevgi, K.; Tepe, B.; Sarikurkcu, C. Antioxidant and DNA Damage Protection Potentials of Selected Phenolic Acids. Food and Chemical Toxicology 2015, 77, 12–21.
- Tepe, B.; Degerli, S.; Arslan, S.; Malatyali, E.; Sarikurkcu, C. Determination of Chemical Profile, Antioxidant, DNA Damage Protection and Antiamoebic Activities of Teucrium Polium and Stachys Iberica. Fitoterapia 2011, 82, 237–246.
- Xin, C.; Nishida, H.; Konishi, T. Oxidant-Induced DNA Damage and the Reparation in NIH3T3 Mouse Fibroblasts by Single Cell Gel Electrophoresis. Journal of Xi’an Jiaotong University (Medical Sciences) 2007, 1, 006.
- Deng, S.; Xu, K. Q. Research Progress of DNA Damage Detection Technologies. The Chemistry of Life 2013, 33, 700–705.
- Jeong, J. B.; Seo, E. W.; Jeong, H. J. Effect of Extracts from Pine Needle against Oxidative DNA Damage and Apoptosis Induced by Hydroxyl Radical via Antioxidant Activity. Food and Chemical Toxicology 2009, 47, 2135–2141.
- Chatsumpun, M.; Chuanasa, T.; Sritularak, B.; Likhitwitayawuid, K. Oxyresveratrol Protects against DNA Damage Induced by Photosensitized Riboflavin. Natural Product Communications 2011, 6, 41–44.
- Hu, S.; Chen, F.; Wang, M. Photoprotective Effects of Oxyresveratrol and Kuwanon O on DNA Damage Induced by UVA in Human Epidermal Keratinocytes. Chemical Research in Toxicology 2015, 28, 541–548.
- Yen, G.-C.; Duh, P.-D.; Lin, C.-W. Effects of Resveratrol and 4-Hexylresorcinol on Hydrogen Peroxide-Induced Oxidative DNA Damage in Human Lymphocytes. Free Radical Research 2003, 37, 509–514.
- Burkhardt, S.; Reiter, R. J.; Tan, D.-X.; Hardeland, R.; Cabrera, J.; Karbownik, M. DNA Oxidatively Damaged by Chromium (III) and H 2 O 2 Is Protected by the Antioxidants Melatonin, N 1-Acetyl-N 2-Formyl-5-Methoxykynuramine, Resveratrol and Uric Acid. The International Journal of Biochemistry & Cell Biology 2001, 33, 775–783.
- López‐Burillo, S.; Tan, D. X.; Mayo, J. C.; Sainz, R. M.; Manchester, L. C.; Reiter, R. J. Melatonin, Xanthurenic Acid, Resveratrol, EGCG, Vitamin C and α‐lipoic Acid Differentially Reduce Oxidative DNA Damage Induced by Fenton Reagents: A Study of Their Individual and Synergistic Actions. Journal of Pineal Research 2003, 34, 269–277.
- Duthie, S.; Collins, A.; Duthie, G.; Dobson, V. Quercetin and Myricetin Protect against Hydrogen Peroxide-Induced DNA Damage (Strand Breaks and Oxidised Pyrimidines) in Human Lymphocytes. Mutation Research/Genetic Toxicology and Environmental Mutagenesis 1997, 393, 223–231.
- Aherne, S.; O’Brien, N. Protection by the Flavonoids Myricetin, Quercetin, and Rutin against Hydrogen Peroxide-Induced DNA Damage in Caco-2 and Hep G2 Cells. Nutrition and Cancer 1999, 34, 160–166.
- Zhou, D. F.; Ke, W. Z.; Ji, K. Protective Effect of Vitamin C on Ultraviolet Radiation-Induced DNA Damage. Acta Optica Sinica 2005, 25, 643–646.
- Horvathova, E.; Navarova, J.; Galova, E.; Sevcovicova, A.; Chodakova, L.; Snahnicanova, Z.; Melusova, M.; Kozics, K.; Slamenova, D. Assessment of Antioxidative, Chelating, and DNA Protective Effects of Selected Essential Oil Components (Eugenol, Carvacrol, Thymol, Borneol, Eucalyptol) of Plants and Intact Rosmarinus Officinalis Oil. Journal of Agricultural and Food Chemistry 2014, 62, 6632–6639.
- Liu, H. X.; Zhou, L.; Ding, Z. H. UV Radiation-Induced DNA Damage in Keratinocytes and the Protective Effect of Resveratrol. The. Journal of Practical Medicin 2015, 31, 3822–3825.