ABSTRACT
Almond (Terminalia catappa) is a species used in Nigerian folkloric medicine because of its antihypertensive property. In this study, the acetylcholinesterase, arginase, phosphodiesterase-5 and angiotensin-1-converting enzyme inhibition and antioxidant properties of the phenolic-rich extracts from almond leaf and stem bark were determined. The chemical composition was evaluated using high-performance liquid chromatography. The total phenol and flavonoid contents were estimated and the antihypertensive potential was estimated by the determination of acetylcholinesterase, arginase, phosphodiesterase-5 and angiotensin-1-converting enzyme inhibition in vitro. Three assays were used for the evaluation of antioxidant properties of the extracts. Eleven and eight phenolic compounds were detected in leaf and stem bark respectively. The phenolic-rich extracts showed remarkable enzyme inhibition activity in concentration dependent manner, with high antioxidant activities. Almond leaf and stem bark can serve as potential natural source for the development of a novel antihypertensive agent.
Introduction
In this dispensation, high blood pressure (BP), also called hypertension, is one of the most common causes of early death in symptom-free adults. Uncontrolled hypertension increases the risk of serious health issues and is considered a major risk factor for stroke, heart attack or myocardial infarction, ischemia, blindness, erectile dysfunction and kidney disease.[Citation1] The increase in blood pumps from the heart and the narrowing of the arteries normally lead to hypertension. Moreover, there is a direct link between systolic BP and hemorrhagic stroke.[Citation2] Longevity and prevalence of contributing factors such as obesity, physical inactivity and unhealthy diet have made hypertension to be an increasingly common health problem worldwide.[Citation3] Over expression of renin angiotensin aldosterone system (RAAS) is one of major causative factors in the development of hypertension.[Citation4] Angiotensin-1-converting enzyme (ACE) plays a prominent role in RAAS, by converting the precursor angiotensin I to angiotensin II which is a system vasoconstrictor, the peptide responsible for the mechanisms which increase the blood pressure. Inhibition of ACE is a promising way of controlling the over activation of RAAS. In addition, free radicals such as reactive oxygen species (ROS) and reactive nitrogen species (RNS) have been implicated in the pathogenesis of several human diseases including atherosclerosis, diabetes mellitus, chronic inflammation, hypertension, among others.[Citation5] Recently, interest has increased considerably in finding naturally occurring antioxidants for use in food and/or pharmaceutical applications, which can protect human body from free radicals and retard the progress of many chronic human diseases.[Citation6]
Inspite of the availability of several antihypertensive agents, which successfully reduce blood pressure in many hypertensive subjects, numerous patients remain unresponsive to treatment and are left with severely high BP.[Citation7] For instance, hypertension is not effectively managed in approximately 10% of populations who are compliant with prescriptive therapeutics.[Citation8] The available synthetic antihypertensive agents in clinical practice are not also effective in all cases in addition to the well-known severity of the disease itself.[Citation9] Moreover, these agents are characterized by high cost, lack of definitive curative regimen, and combination therapy, which hinders drug adherence and increase the risk of side effects as well as drug-drug interactions.[Citation10] These observations are indications that new agents and/or approaches are still needed to manage hypertension.
Herbs have been used as medical treatments since the beginning of human existence and some derivatives (e.g., quinine, reserpine and cardiac glycosides) have become mainstays of human pharmacotherapy.[Citation10] Terminalia catappa belongs to family Combretaceae and its generic name originated from Latin word “terminalis”. It is a tree commonly grown in tropical and subtropical regions because of its edible, ornamental and medicinal purposes.[Citation11] Terminalia catappa is a valuable remedy for several common ailments. Terminalia catappa have found to possess various pharmacological properties, such as anti-stress, anti-oxidant.[Citation11] The leaves, barks and roots are used in traditional medicine for various purposes which have been greatly attributed to the presence of phenolic compounds such as catechin, protocatechuic acid, prenylated benzoic acid, 2-prenylated benzoic acid, and 2-prenyl-4-O-β-D-glucopyranosyl-oxy-4-hydroxybenzoic acid in the stem, root and leaf part of the almond plant.[Citation12] However, despite its traditional usage in the management of hypertension and cardiovascular diseases, there is limited or no information regarding its effectiveness and mode of action. Therefore, this study was aimed to assess the claim of its involvement in folkloric medicine in hypertension management, and most especially to assess its effect on some enzymes relevant to hypertension in vitro.
Materials and methods
Sample collection
Almond leaf and stem bark were collected from Botanical Garden, Federal University of Technology, Akure, Nigeria during dry season (January, 2017). Identification and authentication of the plant was done in the Department of Biology, Federal University of Technology, Akure and a voucher specimen was deposited at the Departmental Herbarium. The fresh plant materials were dried to a constant weight and ground to powder using Laboratory blender.
Sample extraction
Twenty grams of the powdered plant material was extracted by maceration at room temperature using distilled water with plant/solvent ratio of 1:5. After 48 h, it was filtered with Whatman no 1 filter paper. The filtrate was then freeze-dried in a lyophilizer. The lyophilized samples were transferred into a vial and kept in refrigerator (−4°C) prior to subsequent analysis.
Chemicals and reagents
Phosphodiesterase-5 (PDE-5, from S. frugiperda) was obtained from Merck Millipore. Hippuryl-l-histidyl l-leucine (HHL), para-nitrophenylphenylphosphonate, 1,1-diphenyl-2-picrylhydrazyl (DPPH), acetylthiocholine iodide, 5,5ʹ-dithiobio-(2-nitrobenzoic acid) (DTNB), acetylcholinesterase (AChE, from electric eel, type VI-S), arginase (from bovine liver) and angiotensin-1-converting enzyme (ACE, from rabbit lung) were obtained from Sigma–Aldrich Co. (Steinheim, Germany), potassium ferricyanide from BDH Chemicals Ltd. (Poole, England); trichloroacetic acid, ascorbic acid, ferric chloride (FeCl3) and methanol from Merck KGaA (Darmstadt, Germany). All other chemicals and reagents used were of analytical grade and glass distilled water was used throughout.
Phosphodiesterase-5 inhibition assay
The phosphodiesterase-5 inhibitory assays were carried out by Kelly and Butler[Citation13] method with slight modification. Briefly, solution containing the enzyme (concentration 40 µg/ml) with various concentration of extract in Tris-HCl buffer (pH 8.0) containing 1 mM CaCl2 and 50 mM NaCl was incubated at 37°C for 10 min. Thereafter, 200 µl of substrate (5 mM p-nitrophenyl phenylphosphonate) was added. The intensity of p-nitrophenol produced was measured spectrophotometrically at 405 nm. The control experiment was carried out without addition of extract. Phosphodiestrease-5 inhibitory property of the extract was subsequently calculated and expressed as percentage inhibition.
Arginase inhibition assay
Arginase inhibibtion assay was performed according to the method of Kaysen and Strecker.[Citation14] In brief, a solution containing enzyme (concentration of 30 μg/ml) and various concentration of extracts were pre-incubated for 5 min in 0.01 mM Tris-HCl buffer (pH 7.5) containing 0.05 mM MnCl2. The reaction was initiated by addition of 50 mM L-arginine followed by incubation at 37°C for 10 min. The reaction was terminated by adding Ehlrich’s reagent (p-dimethylaminobenzaldehyde in 3.6 N HCl). The amount of urea produced was measured spectrophotometrically at 450 nm. The control experiment was carried out without addition of extract. Arginase inhibitory property of the extract was subsequently calculated and expressed as percentage inhibition.
Acetylcholinesterase inhibition assay
The acetylcholinesterase (AChE) inhibition of the plant samples was assessed by colorimetric method.[Citation15] The AChE activity was determined in a reaction mixture containing 200 μl of a solution of AChE (0.415 U/ml in 0.1 M phosphate buffer, pH 8.0), 100 μl of a solution of 5,5′dithiobis(2nitrobenzoic) acid (3.3 mM) in 0.1 M phosphate buffer solution (pH 7.0) containing NaHCO3 (6 mM), extract dilutions (0–100 μl), and 500 μl phosphate buffer (pH 8.0). After incubation for 20 min at 25°C, acetylthiocholine iodide (100 μL of 0.05 mM water solution) was added as the substrate, and AChE activities were determined by JENWAY UVVisible spectrophotometer from the absorbance changes at 412 nm for 3.0 min at 25°C. The enzyme inhibitory activities were expressed as percentage inhibition.
Angiotensin-i-converting enzyme (ACE) inhibition assay
The inhibition of ACE activity of the extract was determined according to the described method of Cushman and Cheung.[Citation16] Different concentrations of the extract and 50 μl ACE stock solution (4 mU/ml) were preincubated at 37°C for 15 min. Thereafter, enzymatic reaction was initiated by adding 150 μl of 8.33 mM ACE substrate [hippuryl-l-histidyl l-leucine (HHL)] in 125 mM of Tris-HCl buffer (pH 8.3) to the reaction mixture and incubated at 37°C for 30 min. The reaction was stopped by adding 250 μl of 1 M HCl. The hippuric acid (Bz-Gly) produced by the reaction was extracted with 1.5 ml ethyl acetate. The mixture was then centrifuged to separate the ethyl acetate layer, after which the 1 ml of the ethyl acetate layer was transferred to a clean test tube and evaporated to dryness. The residue was re-dissolved in distilled water and its absorbance was measured at 228 nm.
Measurement of the free radicals scavenging capacity
The 1,1-diphenyl–2 picrylhydrazyl (DPPH) radical scavenging capacity of the Almond leaf and stem bark extract was determined using the method of Gyamfi et al..[Citation17] In addition, ABTS* radicals scavenging capacity of the extract was determined by the method previously described by Re et al..[Citation18] Trolox was used as standard.
Ferric reducing power
The ferric reducing power of the extracts was evaluated using method of Oyaizu,.[Citation19] Ascorbic acid was used as standard and results are expressed in mg ascorbic acid equivalent per gram of dry sample (mgAAE/g).
Determination of total phenolic and flavonoid contents
The total phenolic and flavonoid contents of the extracts were determined through Folin-Ciocalteu and aluminium chloride colorimetric methods as described by Singleton et al.[Citation20] and Meda et al.[Citation21] respectively. Total phenolic contents are expressed as mg of gallic acid per gram of dry sample (mgGAE/g), while total flavonoid contents of the extracts are expressed as mg of quercetin per gram of dry sample (mgQUE/g).
Quantification of phenolic compounds by HPLC-DAD
Samples at a concentration of 12 mg/ml were injected by means of a model SIL-20A Shimadzu Auto sampler. Separations were carried out using Phenomenex C18 column (4.6 mm x 250 mm x 5 μm particle size). The mobile phase was water with 1% formic acid (v/v) (solvent A) and HPLC grade methanol (solvent B) at a flow rate of 0.6 ml/min and injection volume 40 μl. The composition gradient was: 5% solvent B reaching 15% at 10 min; 30% solvent B at 25 min, 65% solvent B at 40 min and 98% solvent B at 45 min, followed by 50 min at isocratic elution until 55 min. At 60 min the gradient reached the initial conditions again, following the method described by Duarte et al.[Citation22] with slight modifications. The sample and mobile phase were filtered through 0.45 μm membrane filter (Millipore) and then degassed by ultrasonic bath prior to use. Stock solutions of standards references were prepared in the methanol: water (1:1, v/v) at a concentration range of 0.025 – 0.300 mg/ml. Quantifications were carried out by integration of the peaks using the external standard method, at 254 nm for gallic acid, 280 nm for catechin, 327 nm for chlorogenic acid and caffeic acid; and 365 for rutin, apigenin, kaempferol and luteolin. The chromatography peaks were confirmed by comparing its retention time with those of reference standards and by DAD spectra (200 to 600 nm). Calibration curve for gallic acid: Y = 13278x + 1192.5 (r2 = 0.9998); catechin: Y = 10957x + 1083.4 (r2 = 0.9999); kaempferol: Y = 11635x + 1403.9 (r2 = 0.9996); chlorogenic acid: Y = 12735x + 1354.0 (r2 = 0.9995); caffeic acid: Y = 11458x + 1191.8 (r2 = 0.9999); apigenin: Y = 14183x + 1194.1 (r2 = 0.9997); luteolin: Y = 12950x + 1362.3 (r2 = 0.9994); ellagic acid: Y = 12631x + 1095.8 (r2 = 0.9999); quercetin: Y = 13256x + 1272.5 (r2 = 0.9995) and rutin: Y = 13791x + 1428.5 (r2 = 0.9999). All chromatography operations were carried out at ambient temperature and in triplicate.
Statistical analysis
All the analyses were carried out in triplicates. The results are expressed as mean ± SD. Statistical comparisons were investigated by one-way ANOVA followed by Duncan’s post-hoc test for multiple comparisons with control. Statistical analyses were performed using Statistical package for social sciences (SPSS) 16.0 software. Furthermore, the IC50 values were calculated using non-linear regression analysis.
Results and discussion
Enzyme inhibition assays
The role of renin-angiotensin system (RAS) in regulating blood pressure cannot be underestimated. Renin catalyzes the conversion of inactive angiotensinogen to angiotensin I, it is thereafter converted to a potent system vasoconstrictor (angiotensin II) by angiotensin-1-converting enzyme (ACE). Elevated ACE activity has been linked with hypertension as it degrades bradykinin, a system vasodilator.[Citation23] However, inhibition of ACE activity could be of immense benefit in lowering blood pressure and in the overall management of hypertension. In this present study, the ACE inhibitory property of almond leaf and stem bark was carried out and result revealed that extracts inhibited ACE in concentration-dependent manner (). However, stem bark extract showed a higher inhibitory effect against ACE activity than leaf extract as shown by IC50 values (). Several synthetic ACE inhibitors are currently in use and have been proved to be effective in the management of hypertension.[Citation24] Nevertheless, adverse effects and cost implications associated with the use of synthetic ACE inhibitors increase the search for naturally-occurring ACE inhibitors. These naturally-occurring substances have been shown to be readily available with little or no adverse effect.[Citation23] Previous findings have highlighted the antihypertensive and cardio-protective properties of some medicinal plants and plant foods.[Citation23,Citation25–Citation28] Almond leaf and stem bark inhibit ACE activity, and could therefore serve as natural and alternative therapy in the management of hypertension. However, ACE inhibitory potential of almond leaf and stem bark could be related to the inherent phytochemicals especially phenolic acids and flavonoids.
One of important strategies for treatment of hypertension is the inhibition of phosphodiesterase-5. Phosphodiesterase-5 (PDE-5) inhibitors act by inhibiting PDE-5, a key metabolic enzyme of intracellular cyclic guanosine monophosphate (cGMP) in pulmonary vasculature. cGMP serves as intracellular second messenger for nitric oxide (NO) and natriuretic peptide (NP) signaling and initiates most of muscle relaxation especially of pulmonary circulation.[Citation29] Almond leaf and stem bark extracts inhibited PDE-5 in a concentration-dependent manner (Fig. 1B). However, leaf extract showed higher inhibitory effect against PDE-5 activity (IC50 = 238.44 µg/ml) than stem bark extract (IC50 = 273.43 µg/ml) (). Recently, it was shown that PDE-5 activity was found to be increased in hypertensive diseases, thereby elevating the cGMP hydrolysis and thereby causing impairment of cGMP-mediated pulmonary vasodilation.[Citation30] Several in vitro studies have screened some medicinal plants for PDE-5 inhibitory potentials.[Citation31-Citation33] In addition, findings revealed that inhibition of PDE-5 have been shown to reverse the acute pulmonary vasoconstriction and thereby attenuate the development of hypertension in animal models.[Citation34] Inhibition of PDE-5 by the extracts of almond leaf and stem bark could serve as therapeutic approach in the management of hypertension, and this could be associated with inherent polyphenols which have been previously described to possess PDE-5 inhibitory effects.[Citation35]
Figure 1. A. angiotensin-1-converting enzyme (ACE), B. phosphodiesterase-5 (PDE-5), C. acetylcholinesterase (AChE) and D. arginase inhibitory activities of almond leaf and stem bark extracts.
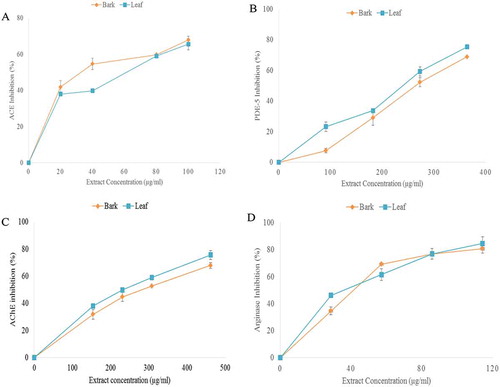
Table 1. Total Phenolic content, flavonoid content and ABTS radical scavenging ability of almond leaf and bark.
Table 2. IC50 values for DPPH radical scavenging ability and arginase, phosphodiesterase-5 (PDE-5), acetylcholinesterase (AChE) and angiotensin-1-converting enzyme (ACE) inhibitory activities of almond leaf and stem bark.
Acetylcholinesterase (AChE) has been reported to play a crucial role in regulating number of vital functions such as learning and cerebral blood flow which reveals the level of importance of acetylcholine as a neurotransmitter target for examining cerebrovascular diseases related hypertension.[Citation36] Acetylcholine is a neurotransmitter produced at the synaptic gap. Deficient cholinergic functions and neurotransmitter disturbances among others have been identified as pathological features in many disorders.[Citation36] This study evaluated the capacity of Almond leaf and stem bark as inhibitor of acetylcholinesterase, an important enzyme in the breakdown of acetylcholine. The study revealed that both almond leaf and stem bark extracts showed concentration-dependent inhibition of AChE (Fig. 1C). The results () showed that leaf extract had a higher inhibitory activity (IC50 = 265.39 µg/ml) when compared with stem bark (IC50 = 303.53 µg/ml). It is within the interest of our study to show that this paramount activity of almond leaf and stem bark may apparently be attributed to their high content of polyphenols such as caffeic acid and chlorogenic acid, which have been shown to possess inhibitory property against AChE.[Citation37,Citation38]
Arginase, a metalloprotein enzyme has been implicated to play a crucial role in heart and kidney function. This study shows that both extracts (almond leaf and stem bark) inhibited arginase activity in a concentration-dependent manner (). However, there was no significant difference in their arginase inhibitory activities (). Increased arginase activity has been linked to several vascular difficulties including atherosclerosis, erectile dysfunction and hypertension.[Citation39,Citation40] Elevated arginase activity can impair endothelium-dependent vasorelaxation by lowering L-arginine availability to endothelium nitric oxide synthase (eNOS), thereby decreasing NO production and uncoupling eNOS function.[Citation41] Interestingly, downregulation of arginase has been shown to increase NO production and vasorelaxant responses to acetylcholine in hypertensive and high-fat diet animal models.[Citation42] Convincingly, the inhibitory property of these extracts on arginase could be a function of their inherent polyphenols. Flavonoids, a typical class of polyphenol have been reported to possess ability to form hydrogen bond and hydrophobic interactions with some amino acids at the active site of arginase, thereby inhibiting its activities.[Citation43,Citation44]
Antioxidant and free radicals scavenging ability of extracts
Fig. 2A depicts the dose dependent DPPH radical scavenging ability of almond leaf and stem bark extracts as expressed as a percentage of the ratio of the decrease in absorbance of the test solution to that of DPPH solution without plants extracts. The results showed that both the extracts had a propensity in quenching DPPH free radicals, as indicated by the geometric increase in radical scavenging ability of the extracts. The IC50 values of the extracts are presented in . Almond leaf extract had a higher DPPH radical scavenging ability (IC50 = 0.49 mg/ml) than stem bark extract (IC50 = 0.57 mg/ml). In addition, ABTS radical scavenging ability of the extracts is presented in . However, almond leaf extract had a higher ABTS radical scavenging ability (0.17 mmolTEAC/g) when compared with stem bark extract (0.09 mmolTEAC/g). It is interesting to note that almond leaf extract showed higher radicals scavenging activity also had higher total phenol and flavonoid contents. This finding agrees with earlier report that established the correlation between the phenolic contents of some medicinal plants and their radicals scavenging ability.[Citation45] It is a known fact that overproduction of free radicals is the basis of pathogenesis of a number of diseases[Citation46], and that intake of natural antioxidants can prevent the associated health problems caused by oxidative stress.[Citation47]
Ferric reducing power
The ferric reducing antioxidant power (FRAP) of almond leaf and stem bark is presented in Fig. 2B. Both samples have strong reducing power, but leaf extract had higher reducing ability (47.73 mgAAE/g) when compared with stem bark (39.55 mgAAE/g). FRAP detects compounds with oxidation-reduction potentials, the potential of the Fe3+-TPTZ product. Unlike other radical scavenging assays, FRAP is an entirely electron transfer reaction rather than hydrogen atom observed in most reactions.[Citation48] In this study, there is a correlation between the phenolic contents and ferric reducing power of the extracts. This finds agreement with earlier study carried out by Frankel et al..[Citation49]
Polyphenolic composition
Composition of phenolic compounds in aqueous almond leaf and stem bark extracts was determined quantitatively by high-performance liquid chromatography (HPLC) analysis. The analysis revealed the presence of gallic acid (tR = 9.97 min; peak 1), catechin (tR = 16.39 min; peak 2), chlorogenic acid (tR = 21.47 min; peak 3), caffeic acid (tR = 25.03 min; peak 4), ellagic acid (tR = 31.20; peak 5), epicatechin (tR = 32.71 min; peak 6), rutin (tR = 39.98 min; peak 7), quercitrin (tR = 44.15 min; peak 8), isoquercitrin (tR = 47.83 min; peak 9), quercetin (tR = 52.64 min; peak 10) and kaempferol (tR = 59.86 min; peak 11) in almond leaf (, ). While in stem bark, the analysis revealed the presence of gallic acid (tR = 10.27 min, peak 1), catechin (tR = 15.83 min, peak 2), caffeic acid (tR = 22.07 min, peak 3), ellagic acid (tR = 23.98 min, peak 4), resveratrol (tR = 29.65 min, peak 5), rutin (tR = 34.51 min, peak 6), quercetin (tR = 39.46 min, peak 7) and kaempferol (tR = 43.01 min, peak 8) (Figs. 3 A & B and ). It was evident that almond leaf and stem bark are rich source of polyphenols which validated high antioxidant, anti-phosphodiesterase-5, anti-arginase, anti-acetylcholinesterase and anti-angiotensin-1-converting enzyme activities of almond leaf and stem bark extracts. Our findings are supported by the facts that some of the identified phenolic compounds namely chlorogenic acid, caffeic acid, rutin and quercetin have already been shown to possess anti-cholinesterase and inhibitory effect on enzymes discussed in this study.[Citation35,Citation50,Citation51] Anouar et al.[Citation52] reported that phenolics act as antioxidants by attenuating enzymes in radical generation. In another study, Xu et al.[Citation53] reported that number of flavonoids affect smooth muscle contractility in response to various agonists.
Figure 3. Representative of high performance liquid chromatography profile A. almond leaf; Gallic acid (peak 1), catechin (peak 2), chlorogenic acid (peak 3), caffeic acid (peak 4), ellagic acid (peak 5), epicatechin (peak 6), rutin (peak 7), quercitrin (peak 8), isoquercitrin (peak 9), quercetin (peak 10) and kaempferol (peak 11) B. almond stem bark; Gallic acid (peak 1), catechin (peak 2), caffeic acid (peak 3), ellagic acid (peak 4), resveratrol (peak 5), rutin (peak 6), quercetin (peak 7) and kaempferol (peak 8).
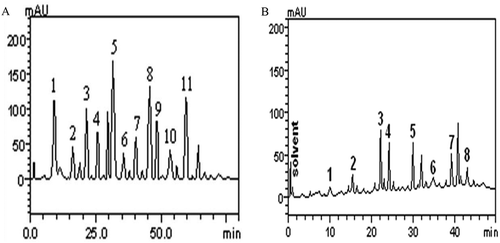
Table 3. Phenolics composition of Almond leaf and stem bark extracts.
Total phenol and flavonoid contents
The total phenol content of almond leaf and stem bark was evaluated using gallic acid as standard. It was found to be higher in leaf extract (62.50 ± 1.84 mgGAE/g) than stem bark extract (37.30 ± 1.05 mgGAE/g). In the same vein, the total flavonoid content was evaluated using quercetin as standard. Results revealed higher flavonoid content in leaf extract (7.80 ± 1.32 mgQUE/g) when compared stem bark extract (3.44 ± 0.51 mgQUE/g) (). The study of total phenol and flavonoid contents of other medicinal plant species excluding almond leaf and stem bark have been carried out by several researchers.[Citation54–Citation57]
Conclusion
This study represents the exhaustive analysis of total phenol and flavonoid contents, antioxidant, anti-acetylcholinesterase, anti-phosphodiesterase-5, anti-arginase, anti-angiotensin-1-converting enzyme and phenolic composition of almond leaf and stem bark, a medicinal plant food in Nigeria. The study revealed that almond leaf and stem bark extracts have high phenolic and flavonoid contents with remarkable antioxidant activities and potential inhibitor of some enzymes involved in hypertension. Study also revealed presence of number of polyphenols with known anti-oxidative and antihypertensive properties. These activities of almond leaf and stem bark extracts suggest their utilization for the development of alternative formulations for general health maintenance and effective antihypertensive agent.
References
- Zimmet, P.; Alberti, K.; Shaw, J. Global and Societal Implications of the Diabetespidemic. Nature 2001, 414, 782–787.
- Kanter, M.; Coskun, O.; Korkmaz, A.; Oter, S. Effects of Nigella Sativa on Oxidative Stress and β-cell Damage in Streptozotocin-Induced Diabetic Rats. Anat. Rec ADiscov Mol Cell Evol Biol 2004, 279, 685–691.
- Kunes, J.; Zicha, J. The Interaction of Genetic and Environmental Factors in the Etiology of Hypertension. Physiology Research 2009, 58, S33–S41.
- Hammoud, R. A.; Vaccari, C. S.; Nagamia, S. H.; Khan, B. V. Regulation of the Renin- Angiotensin System in Coronary Atherosclerosis: A Review of the Literature. Vascular Health Risk Management 2007, 3, 937–945.
- Halliwell, B.;. Antioxidants in Human Health and Diseases. Annual Review of Nutrition 1996, 16, 33–35.
- Prior, R. L.;. Fruits and Vegetables in the Prevention of Cellular Oxidative Damage. The American Journal of Clinical Nutrition 2003, 78, 570S–578S.
- Kloet, A.; Krause, E.; Shi, P.; Zubcevic, J.; Raizada, M.; Sumners, C. Neuroimmune Communication in Hypertension and Obesity: A New Therapeutic Angle? Pharmacology and Therapeutic 2013, 138, 428–440.
- Taylor, D.; Abdel-Rahman, A. Novel Strategies and Targets for the Management of Hypertension. Advance in Pharmacology 2009, 57, 291–345.
- Lonn, E.;. The Clinical Relevance of Pharmacological Blood Pressure Lowering Mechanisms. Canadian Journal of Cardiology 2004, 20(Suppl B), 83B–88B.
- Kagathara, V.; Ambikar, D.; Vyawahare, N. Hypertension-Animal Models and Phytomedicines. A Review on Pharmacology Online 2009, 2, 436–461.
- Pinelo, M.; Rubilar, M.; Sineiro, J.; Nunez, M. J. Phenolics from Almond Hulls (Prunus Amygdalus) and Pine Sawdust (Pinus Pinaster). Food Chemistry 2004, 85, 267–273.
- Subashinee, S. K. W.; Siriwardhana, S. F. Anti-Radical Activity of Extracts of Almond and Its By-Products. Journal of American Oil and Chemical Society 2002, 79, 903–908.
- Kelly, S. J.; Butler, L. G. Enzymatic Hydrolysis of Phosphonate Esters Reaction Mechanism of Intestinal 5ʹ- Nucleotide Phosphodiesterase. Biochemistry 1977, 16, 1102–1104.
- Kaysen, G. A.; Strecker, H. J. Increased Arginase Activity Levels Caused by Nitric Oxide Synthase Dysfunction. New England Journal of Medicine 1973, 323, 1234–1238.
- Perry, N. S.; Houghton, P. J.; Theobald, A.; Jenner, P.; Perry, E. K. In-Vitro Inhibition of Human Erythrocyte Acetylcholinesterase by Salvia Lavandulaefolia Essential Oil and Constituent Terpenes. Journal of Pharmacy and Pharmacology 2000, 52(7), 895–902.
- Cushman, D. W.; Cheung, H. S. Spectrophotometric Assay Band Properties of the Angiotensin-1-Converting Enzyme of Rabbit Lung. Biochemical Pharmacology 1971, 20, 1637–1684.
- Gyamfi, M. A.; Yonamine, M.; Aniya, Y. Free-Radical Scavenging Action of Medicinal Herbs from Ghana: Thonningiasanguineaon Experimentally-Induced Liver Injuries. General Pharmacology 1999, 32, 661–667.
- Re, R.; Pellegrini, N.; Proteggente, A.; Pannala, A.; Yang, M.; Rice-Evans, C. Antioxidant Activity Applying an Improved ABTS Radical Cation Decolorisation Assay. Free Radical Biology and Medicine 1999, 26, 1231–1237.
- Oyaizu, M.;. Studies on Products of Browning Reaction: Antioxidative Activity of Products of Browning Reaction Prepared from Glucosamine. Japanese Journal of Nutrition 1986, 44, 307–315.
- Singleton, V. L.; Orthofor, R.; Lamuela-Raventos, R. M. Analysis of Total Phenols and Other Oxidation Substrates and Antioxidants by Means of Folin-Ciocaltau Reagent. Methods Enzymology 1999, 299, 152–178.
- Meda, A.; Lamien, C. E.; Romito, M.; Millogo, J.; Nacoulma, O. G. Determination of the Total Phenolic, Flavonoid and Proline Contents in Burkina Faso Honey, as Well as Their Radical Scavenging Activity. Food Chemistry 2005, 91, 571–577.
- Duarte, A. E.; Waczuk, E. P.; Roversi, K.; Da Silva, M. A. P.; Barros, L. M.; Cunha, F. A. B.; Menezes, I. R. A.; Costa, J. G. M.; Boligon, A. A.; Ademiluyi, A. O.; Kamdem, J. P.; Rocha, J. B. T.; Burger, M. E. Polyphenolic Composition and Evaluation of Antioxidant Activity, Osmotic Fragility and Cytotoxic Effects of Raphiodon Echinus (Nees & Mart.). Schauer. Molecules 2016, 21(2), 1–15.
- Je, J. Y.; Park, P. J.; Kim, E. K.; Ahn, C. B. Antioxidant and Angiotensin I Converting Enzyme Inhibitory Activity of Bambusae Caulis in Liquamen. Food Chemistry 2009, 13, 932–935.
- Campos, M. R. S.; Guerrero, L. A. C.; Ancona, D. A. B. Angiotensin-I-converting Enzyme Inhibitory and Antioxidant Activities of Peptide Fractions Extracted by Ultrafiltration of Cowpea Vigna Unguiculata Hydrolysates. Journal Science Food and Agriculture 2010, 90, 2512–2518.
- Pinto, M. D.; Ranilla, L. G.; Apostolidis, E.; Lajolo, F. M.; Genovese, M. I.; Shetty, K. Evaluation of Antihyperglycemia and Antihypertension Potential of Native Peruvian Fruits Using in Vitro Models. Journal of Medicinal Food 2009, 12, 278–291.
- Oboh, G.; Ademosun, A. O. Shaddock Peels (Citrus Maxima) Phenolic Extracts Inhibit α-amylase, α-glucosidase and Angiotensin-I-Converting Enzyme Activities: A Nutraceutical Approach to Diabetes Management. Diabetes Metabolic Syndrome Clinical Research and Review 2011, 5(3), 148–152.
- Shodehinde, S. A.; Oyeleye, S. I.; Olasehinde, T. A.; Adebayo, A. A.; Oboh, G.; Boligon, A. A. Lasianthera Africana Leaves Inhibits α-amylase α-glucosidase, angiotensin-I- Converting Enzyme Activities and Fe2+-Induced Oxidative Damage in Pancreas and Kidney Homogenates. Oriental Pharmaceutical and Experimental Medicine 2017. DOI:10.1007/s13596-017-0256-z.
- Oboh, G.; Olasehinde, T. A.; Ademosun, A. O. Inhibition of Enzymes Linked to Type-2 Diabetes and Hypertension by Essential Oils from Peels of Orange and Lemon. International Journal of Food Properties 2017. DOI:10.1080/10942912.2017.1303709.
- Klinger, J. R.;. The Nitric oxide/cGMP Signaling Pathway in Pulmonary Hypertension. Clinical Chest Medicine 2007, 28(1), 143–167.
- Mikhail, G. W.; Prasad, S. K.; Li, W.;, et al. Clinical and Haemodynamic Effects of Sildenafil in Pulmonary Hypertension: Acute and Mid-Term Effects. European Heart Journal 2004, 25, 431–436.
- Oboh, G.; Adebayo, A. A.; Ademosun, A. O.; Boligon, A. A. In Vitro Inhibition of Phosphodiesterase-5 and Arginase Activities from Rat Penile Tissue by Two Nigerian Herbs (Hunteria Umbellata and Anogeissus Leiocarpus). Journal of Basic Clinical Physiology and Pharmacology. 2017, 28(4), 393–401. DOI:10.1515/jbcpp-2016-0143.
- Oboh, G.; Ademiluyi, A.; Oyeleye, S. I.; Olasehinde, T. A.; Boligon, A. Modulation of Some Markers of Erectile Dysfunction and Malonaldehyde Levels in Isolated Rat’s Penile Tissue with Unripe and Ripe Plantain Peels: Identification of the Constituents of the Plants Using HPLC. Pharmaceutical Biology 2017. DOI:10.1080/13880209.2017.1340966.
- Oboh, G.; Adebayo, A. A.; Ademosun, A. O. Erection-Stimulating, Anti-Diabetic and Antioxidant Properties of Hunteria Umbellata and Cylicodiscus Gabunensis Water Extractable Phytochemicals. Journal of Complementary and Integrative Medicine 2017. DOI:10.1515/jcim-2016-0164.
- Zhao, L.; Mason, N. A.; Morrell, N. W.;, et al. Sildenafil Inhibits Hypoxia-Induced Pulmonary Hypertension. Circulation 2001, 104(4), 424–428.
- Ko, W. C.; Shih, C. M.; Lai, Y. H.; Chen, J. H.; Huang, H. L. Inhibitory Effects of Flavonoids on Phosphodiesterase Isozymes from Guinea Pig and Their Structure-Activity Relationships. Biochemical Pharmacology 2004, 68(10), 2087–2094.
- Cardoso, A. M.; Abdalla, F. H.; Bagatini, M. D.; Martins, C. C.; Fiorin, F. S.; Baldissarelli, J.; Da Costa, P.; Mello, F. F.; Fiorenza, A. M.; Serres, J. D.; Gonçalves, J. F.; Chaves, H.; Royes, L. F.; Belló-Klein, A.; Morsch, V. M.; Schetinger, M. R. Swimming Training Prevents Alterations in Acetylcholinesterase and Butyrylcholinesterase Activities in Hypertensive Rats. American Journal of Hypertension 2014, 27, 522–529.
- Oboh, G.; Agunloye, O.; Akinyemi, A.; Ademiluyi, A. O.; Adefegha, S. A. Comparative Study on the Inhibitory Effect of Caffeic and Chlorogenic Acids on Key Enzymes Linked to Alzheimer’s Disease and Some Pro-Oxidant Induced Oxidative Stress in Rat’s Brain– In Vitro. Neurochem Res 2012, 11, 1–4.
- Zengin, G.; Locatelli, M.; Stefanucci, A.; Macedonio, G.; Novellino, E.; Mirzaie, S.; Dvorácskó, S.; Carradori, S.; Brunetti, L.; Orlando, G.; Menghini, L.; Ferrante, C.; Recinella, L.; Chiavaroli, A.; Leporini, L.; Mollica, A. Chemical Characterization, Antioxidant Properties, Anti-Inflammatory Activity and Enzyme Inhibition of Ipomoea Batatas L. Leaf Extracts. International Journal of Food Properties 2017. DOI:10.1080/10942912.2017.1357127.
- Zhang, C.; Hein, T. W.; Wang, W.; Miller, M. W.; Fossum, T. W.; McDonald, M. M.; Humphrey, J. D.; Kuo, L. Upregulation of Vascular Arginase in Hypertension Decreases Nitric Oxidemediated Dilation of Coronary Arterioles. Hypertension 2004, 44, 935–943.
- Morris, C. R.; Kato, G. J.; Poljakovic, M.; Wang, X.; Blackwelder, W. C.; Sachdev, V.; Hazen, S. L.; Vichinsky, E. P.; Morris, S. M., Jr; Gladwin, M. T. Dysregulated Arginine Metabolism, Hemolysis Associated Pulmonary Hypertension, and Mortality in Sickle Cell Disease. Journal of American Medical Association 2005, 294, 81–90.
- Toque, H. A.; Tostes, R. C.; Yao, L.; Xu, Z.; Webb, R. C.; Caldwell, R. B.; Caldwell, R. W. Arginase II Deletion Increases Corpora Cavernosa Relaxation in Diabetic Mice. Journal of Sexual Medicine 2011, 8, 722–733.
- Demougeot, C.; Prigent-Tessier, A.; Marie, C.; Berthelot, A. Arginase Inhibition Reduces Endothelial Dysfunction and Blood Pressure Rising in Spontaneously Hypertensive Rats. Journal of Hypertension 2005, 5, 971–978.
- Dos Reis, M. B. G.; Manjolin, L. C.; Maquiaveli, C. D. C.; Santos-Filho, O. A.; Da Silva, E. R. Inhibition of Leishmania (Leishmania) Amazonensisand Rat Arginases by Green Tea EGCG, (+)-Catechin and (-)-Epicatechin: A Comparative Structural Analysis of Enzyme- Inhibitor Interactions. PLoS ONE 2013, 8(11), Article ID e78387.
- Araújo, T. A. S.; Alencar, N. L.; De Amorim, E. L. C.; De Albuquerque, U. P. A New Approach to Study Medicinal Plants with Tannins and Flavonoids Contents from the Local Knowledge. Journal of Ethnopharmacology 2008, 120, 72–80.
- Katsube, T.; Tabata, H.; Ohta, Y.; Yamasaki, Y.; Anuurad, E.; Shiwaku, K.;, et al. Screening for Antioxidant Activity in Edible Plant Products: Comparison of Low Density Lipoprotein Oxidation Assay, DPPH Radical Scavenging Assay, and Folin–Ciocalteu Assay. Journal of Agricultural and Food Chemistry 2004, 52, 2391–2396.
- Braca, A.; Politi, M.; Sanogo, R.; Sanou, H.; Morelli, I.; Pizza, C.;, et al. Chemical Composition and Antioxidant Activity of Phenolic Compounds from Wild and Cultivated Sclerocarya Birrea (Anacardiaceae) Leaves. Journal of Agricultural and Food Chemistry 2003, 51, 6689–6695.
- Becker, E. M.; Nissen, L. R.; Skibsted, L. H. Antioxidant Evaluation Protocols: Food Quality or Health Effects. European Food Research Technology 2004, 219, 561–571.
- Prior, L. R.; Wu, X.; Schaich, K. Standardized Methods for Determination of Antioxidant Capacity and Phenolics in Foods and Dietary Supplements. Journal of Agricultural and Food Chemistry 2005, 53, 4290–4302.
- Frankel, E. N.; Waterhouse, A. L.; Teissedre, P. L. Principal Phenolic Phytochemicals in Selected California Wines and Their Antioxidant Activity in Inhibiting Oxidation of Human Low-Density Lipoproteins. Journal of Agricultural and Food Chemistry 1995, 43, 890–894.
- Stefan, J.; Schmieder, R. E. Potential Mechanisms of Impaired Endothelial Function in Arterial Hypertension and Hypercholesterolemia. Current Hypertension Reports 2003, 5(3), 199–207.
- Duarte, J.; Perez-Vizcaino, F.; Zarzuelo, A.; Jimenez, J.; Tamargo, J. Vasodilator Effects of Quercetin in Isolated Rat Vascular Smooth Muscle. European Journal of Pharmacology 1993, 239(1–3), 1–7.
- Anouar, E.; Kosinova, P.; Kozlowski, D.; Mokrini, R.; Duroux, J. L.; Trouillas, P. New Aspects of the Antioxidant Properties of Phenolic Acids: A Combined Theoretical and Experimental Approach. Physical Chemistry and Physics 2009, 11, 7659–7668.
- Xu, Y. C.; Leung, S. W. S.; Yeung, D. K. Y.; Hu, L. H.; Chen, G. H.; Che, C. M.; Man, R. Y. K. Structure Activity Relationships of Flavonoids for Vascular Relaxation in Porcine Coronary Artery. Phytochemistry 2007, 68(8), 1179–1188.
- Ademiluyi, A. O.; Oboh, G.; Ogunsuyi, O. B.; Oloruntoba, F. M. A Comparative Study on Antihypertensive and Antioxidant Properties of Phenolic Extracts from Fruit and Leaf of Some Guava (Psidium Guajava L.) Varieties. Comparative Clinical Pathology 2015. DOI:10.1007/s00580-015-2192-y.
- Oboh, G.; Ademiluyi, A. O.; Ademosun, A. O.; Olasehinde, T. A.; Oyeleye, S. I.; Boligon, A. A.; Athayde, M. L. Phenolic Extract from Moringa Oleifera Leaves Inhibits Key Enzymes Linked to Erectile Dysfunction and Oxidative Stress in Rats’ Penile Tissues. Biochemistry Research International 2015, ArticleID 175950, 8.
- Aydın, C.; Mammadov, R. Phenolic Composition, Antioxidant, Antibacterial, Larvacidal against Culex Pipiens, and Cytotoxic Activites of Hyacinthella Lineata Steudel Extracts. International Journal of Food Properties 2016. DOI:10.1080/10942912.2016.1236271.
- Ran, J.; Sun, H.; Xu, Y.; Wang, T.; Zhao, R. Comparison of Antioxidant Activities and HPLC Analysis of Polyphenol from Different Apple Varieties. International Journal of Food Properties 2016. DOI:10.1080/10942912.2015.1037958.