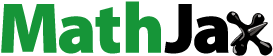
ABSTRACT
In the present study, we investigated the structure and selected functional properties of zein protein as treated with subcritical water. The results showed that subcritical water treatment, with different time (20–120 min) and temperatures (110–170°C), increased the solubility, foam capacity, and foam stability of zein protein significantly (p < 0.05). Analyses of particle size analysis, differential scanning calorimetry, circular dichroism spectra, scanning electron microscopy, and atomic force microscopy indicated that subcritical water treatment improved the thermal stability and the denaturation temperature of zein protein, as well as increased slightly in the percentage of ordered structural elements within the protein molecule. In addition, the particle size of zein protein increased and the surface became rough significantly. All these suggested that subcritical water is a potential green chemical, which could highly be used to change the structure and improve the functional properties of zein protein.
Introduction
Subcritical water is defined as hot water which has a temperature range between 100°C and 374°C, which is under high pressure to keep water in a liquid state. Dielectric constant may be the most important factor when using water as an extraction solvent, which can be changed by temperature and decreased from 80 (at room temperature) to 27 (at 250°C) and it becomes similar to that of ethanol at ambient temperature.[Citation1,Citation2] Subcritical water treatment is a green, environmentally friendly technology with a large range of applications, which include extraction, hydrolysis, and wet oxidation of organic compounds, etc.[Citation3–Citation9] Thus, subcritical water can replace the organic solvent which can’t be accepted by environmental factors and can extract organic compounds. In recent years, subcritical water has attracted researchers’ attention on hydrolysis and conversion of biomass and carbohydrates to useful compounds extensively.[Citation10–Citation16]
Subcritical water has a strong ability to dissolve compounds and also could modify the molecules and change its structure and functional properties.[Citation17] The functional properties of protein (emulsification, foam ability and flavor, water holding capacity, etc.) are related to its structure, composition of amino acids, protein sequence, conformation, molecular shape and size, charge distribution, and intramolecular and intermolecular bonding effect. However, lack of structure or composition of some proteins leads to the failure of some function to express normally. Therefore, the modification of proteins is an important way to improve the practical value of proteins.
At present, a large number of studies have been carried out on the modification of biological macromolecules by using subcritical water. For example, subcritical water treatment had much enhanced rutin-combined capacity of soybean protein isolate (SPI) than that of conventional methods.[Citation18] Remarkable improvement of emulsifying ability and physical stability of emulsion by subcritical water treatment was probably associated with higher surface protein loads.[Citation19] Subcritical water treatment could significantly enhance the emulsifying and foaming properties and solubility of SPI and could modify the structure of SPI,[Citation20] which demonstrated that the subcritical water pretreatment could change the structure and improve the functional properties of SPI.
As we all know, zein is the major storage protein in maize, which accounts for 50% or more of the total endospermic proteins, and is an economically important by-product of the corn starch industry.[Citation21] Zein is hydrophobic and insoluble in water, and even low salt concentrations; thus, ethanol at high concentrations (60–95%) is required to maintain its molecular conformation.[Citation22,Citation23] Therefore, zein has not been widely applied for the food industry as a functional and nutritional food ingredient, mainly due to its hydrophobic amino acid content and many sulfur-containing amino acids, and its deficiencies in essential and polar amino acids.[Citation24]
Most of the previous studies focused on the extraction of active substances by subcritical water.[Citation4,Citation25–Citation27] Fewer studies have investigated the effects of subcritical water pretreatment on the functional properties of food proteins. Some studies have shown that subcritical water pretreatment could change the secondary and advanced structure of proteins.[Citation7] However, the influence of subcritical water pretreatment on the structure and properties of zein still requires further study. Therefore, the main objective of our study was to investigate the effects of the subcritical water pretreatment on the structure and properties of zein protein with variations in the treatment time (20–120 min) and temperatures (110–170°C).
Materials and methods
Materials
Zein sample (>98% pure) was purchased from Gaoyou Ruixing Garmaceutical Adjuvant Co. (Yangzhou, China). All other solvents and chemicals were analytical grade and were purchased from Sinopharm Chemical Reagent Co. (Shanghai, China).
Subcritical water treatment of zein
The batch reactor used a high pressure-resistant vessel made from stainless steel with a net volume of 50 mL. The temperature was monitored and controlled by using an aluminum block heater equipped with a temperature controller and a type-K thermocouple. The pressure inside the vessel varied corresponding to the saturated vapor pressure of water at specific treatment temperature.
Zein protein (0.5 g) was mixed with distilled water (30 mL) in the vessel, the temperature of solution was controlled at 25 ± 2°C through the water bath, and the vessel was tightly closed and heated at the desired temperature. Treatment time and temperature are the two most important parameters of subcritical water treatment. In our study, the heat treatment time was controlled in 20 min with 110°C, 130°C, 150°C, and 170°C, and carried out for durations of 20, 40, 60, 80, 100, and 120 min at 120°C. When reaching the setting time, the vessel was cooled immediately with running tap water to stop the reaction. After the specified time and temperatures, the zein samples were centrifuged at 2,500 g and 20°C for 20 min. The pretreated zein samples were collected and freeze-dried (Freeze dryer ALPHA 1–2, Martin Christ GmbH, Osterode, Germany). Then the samples were measured by particle size analysis (PSA), differential scanning calorimetry (DSC), circular dichroism spectra (CD), scanning electron microscopy (SEM), and atomic force microscopy (AFM). Control sample without subcritical water treatment was also investigated for comparison.
Solubility of zein protein
After subcritical water treatment as described earlier, the samples were centrifuged at 8,000 g for 20 min at room temperature. The protein concentration of the supernatant was measured by the Folin phenol method,[Citation28] and with bovine serum albumin (BSA) as the standard protein.
Particle size determination
The particle size of zein proteins in 2% in aqueous ethanol (70% v/v) was measured at room temperature by laser light scattering with a Mastersizer 2000 instrument (Malvern instruments, Malvern, UK).
Foam capacity and foam stability
Foam capacity (FC) and foam stability (FS) of the zein protein were determined by a previously described method[Citation29] with minor modifications. Zein protein weighing 0.5 g is dissolved in 20 mL distilled water and homogenized using a high speed scattering machine (ULTRA-TURRAX, IKA T18 basic; Staufen, Germany) at 12,000 g for 1 min. Then, the blend is immediately transferred to a 50-mL graduated cylinder. The volume was recorded before and after whipping and measured as the percent of volume increase due to whipping. Changes in foam volume were recorded at 30 min of storage. The FC (% volume) and FS (% volume) of each zein protein suspension were calculated using the following formula:
where V1 is the volume of the suspension (mL) before stirring, V2 is the volume of the suspension (mL) after stirring, and V3 is the volume of the stirred suspension (mL) after it was allowed to stand.
DSC analysis of zein
DSC analyses of zein proteins were carried out on an STA-449C Jupiter thermo-microbalance (Netzsch, Selb, Germany). The programmed heating temperature range was from room temperature to 200°C at the heating rate of 10°C/min under nitrogen atmosphere. Measurements were performed on samples 3–6 mg using an aluminum pan crucible. Zein samples were centrifuged at 2,500 g for 10 min at 20°C. Finally, the zein samples were collected and freeze-dried (ALPHA 1–2). An empty Al pan was used as a reference.
CD analysis of zein
CD spectra were recorded with a spectropolarimeter (Model JASCO J-815, JASCO Corporation, Tokyo, Japan) using a quartz cuvette with a 1-mm optical path length at room temperature (25 ± 1°C). CD spectra were scanned in the far UV range (250–190 nm) with three replicates at 50 nm/min, using a 1.0-nm bandwidth. The content of the protein secondary structure was calculated from the far-UV CD spectra using the Dichro-Web procedure.[Citation30–Citation32] Aqueous ethanol (60% v/v), used to dissolve the zein samples, was also considered as the blank solution for the samples.
Scanning electron microscopy
A thin-layer sample granule was mounted on the copper sample holder with a double-sided carbon tape and then coated with gold (10 nm thick) to make the sample conductive. SEM was conducted with a SEM (JSM-7001F, JEOL. Tokyo. Japan) at an acceleration voltage of 15 kV.
Atomic force microscopy
AFM measurements were performed using a method described by Guo et al.[Citation33] A NanoScopellla (Digital instruments Inc., New York, USA) was used to investigate the surface morphology of fibers. The images were scanned in tapping mode in air using commercial silicon cantilevers (Digital Instruments Inc.) with a resonance frequency of 320 kHz.
Statistical analysis
All measurements were carried out in triplicate. Analysis of variance was performed to compare the effects of subcritical water under the significant level of p < 0.05. All graphs and tables were created and calculations were performed using Origin 2016 and Microsoft Office Excel 2007, respectively.
Results and discussion
Effect of subcritical water treatment on the solubility of zein protein
In the present study, we found that subcritical water treatment parameters, time and temperature, have dramatic effects on the solubility of zein protein. As shown in , compared with the control, the solubility of zein protein increased significantly (p <0.05) with the time (20–120 min) increasing and showed a upward tendency. Higher temperature (110–170°C) also significantly (p<0.05) improved the solubility of zein protein, especially the temperature of 110°C (). However, further increased temperature induced degradation of zein solubility. Interestingly, the solubility decreased rapidly at 130°C, and then increased much higher than that of 130°C at 150°C, which might relate to the zein protein aggregation started at 130°C. The solubility increased again at 150°C due to the degradation of Zein proteins. Similar findings were also reported by other authors.[Citation33,Citation34]
Effect of subcritical water treatment on molecular particle size of zein protein
To verify if there is a relation between the solubility and the particle size distribution of zein protein (2% in 70% aqueous ethanol), we determined the molecular particle size of zein. As shown in , the particle size of zein significantly increased with the temperature varying from 110°C to 170°C, which suggested that some small aggregates may have been formed. This result was consistent with the finding of Pu et al.,[Citation35] who found an increase in beclomethasone dipropionate particle size with subcritical water treatment at temperature (120–150°C) and the probability of particle collision increased drastically with increased temperature, leading to the aggregation and formation of large particles. In addition (), the particle size was also increased with the increasing treatment time (20–120 min), indicating that the agglomeration phenomenon occurred with the time prolonging. This phenomenon was consistent with the discovery of Chen et al.,[Citation18] who found that after subcritical water treatment, the larger aggregates emerged which hindered the effects due to their low diffusion coefficient.
Effect of subcritical water treatment on the FC and FS of zein protein
Foaming activity is a measurement of ability of liquid to create foam after aeration. Proteins can help forming the foam because of their surface-active property. FC and FS of the zein proteins are provided in . The FC and FS of zein proteins obtained by subcritical water extraction were found to be significantly higher than that of control sample. This might be due to the higher solubility of zein proteins with subcritical water pretreatment. The FC and FS of zein proteins were affected by subcritical water pretreated time and temperatures. From the results shown in , it is found that the structure of the zein protein was excessively destroyed with higher temperature, which adversely affected FC and FS. Thus, higher subcritical water temperature pretreatment should be avoided as it leads to undesirable property changes. Our result was consistent with Khuwijitjaru et al.,[Citation36] which suggested that increasing treatment time and temperatures raised the rice bran foaming activity with different subcritical water conditions.
Table 1. Foam capacity and foam stability of zein samples.
Effect of subcritical water treatment on the DSC curves of zein protein
The transition of protein from control to a denatured conformation is accompanied by the crack of inter- and intramolecular bond, and the process has to occur in a cooperative manner to be discerned by DSC.[Citation37] Therefore, we used DSC to measure the bond strength and the thermal behavior of zein with different pretreatments, by which the thermally induced detectable process of protein molecule was structural melting or unfolding.
and showed that the thermograms of DSC on samples heated from room temperature to 200°C and showed a single broad exothermic transition between 57°C and 70°C that corresponded to the unfolding of the protein molecules. The denaturation temperature of all samples was calculated by using the thermal scans. DSC analysis showed that the denaturation temperature of control sample was 58.5°C. The different denaturation temperatures of subcritical water pretreated samples were 62.1°C, 61.1°C, 62.1°C, 69.7°C, 62.0°C, and 62.1°C, respectively. The denaturation temperature increased from 58.5°C to 66.2°C, 62.0°C, 61.6°C, and 66.1°C with different pretreated temperatures, respectively. The denaturation temperature increased by the pretreated temperatures and time, indicating that the bond strength and, in turn, the degree of cross-linking increased with the increase of pretreated temperatures and time. DSC results suggested that the subcritical water pretreated samples showed a favorable thermal stability, with a high thermal denaturation temperature. Some similar results have been reported in the literature, the DSC spectra of native BSA (as a control) and PBSA prepared at different temperatures, typically, Native BSA had a transition temperature at 64°C. In the case of PBSA, the transition temperatures were 66°C, 77°C, 78°C, and 85.5°C for samples synthesized at 200°C, 250°C, 275°C, and 300°C, respectively.[Citation38] The results showed that the thermal stability of the samples treated by subcritical water treatment had been significantly improved. Thus, the variations in the depth of the DSC profiles suggested that subcritical water altered the thermal behavior of zein. The increase of thermal stability means that zein is more stable and provides the basis for the expression of some functional properties.
Effect of subcritical treatment on the CD spectra of zein
CD spectroscopy was used to study the structural properties of protein at secondary folding level. The results of far-UV CD spectra (190–260 nm) were presented in and . The results showed there were a positive peak at 195 nm and two negative peaks at 208 and 222 nm, indicating the existence of α-helical secondary structure. The absorption peak reflects the content of α-helix, but the change of absorption intensity of positive and negative peak of zein protein with subcritical water pretreatment is irregular. But this still provides some evidence of successive changes in the secondary structure of zein protein.
The content of α-helix, β-sheet, β-turn, and random coil of zein protein were calculated by DichroWeb procedure ( and ), and the data showed some increases in the percentage of α-helix. Different pretreated time and temperatures resulted in irregularity in the percentage of β-sheet, β-turn, and random coils. Our results were in agreement with the study that reported the effect of subcritical water treatment on the secondary structure of soybean protein solutions using CD spectra.[Citation39]
Table 2. The contents of secondary structure of zein after different time with subcritical water.
Table 3. The contents of secondary structure of zein after different temperatures with subcritical water.
Effect of subcritical water pretreatment on the physical structure of zein
SEM is mainly used to observe the surface morphology of the samples with two electronic signal imaging, using extreme narrow electron beam to scan the samples, exert various effects through the interaction between the electron beam and the sample, which is two times the electron emission of samples.
The size of zein is heterogeneous; the diameter ranges from as small as micrometer to as big as millimeter. There were significant differences in diameter between untreated zein and subcritical water treated zein (). Compared with the smooth surface of the untreated sample, the surface of subcritical water treated samples became rough and their specific surface area was larger. showed that the surface of the untreated sample was smooth. However, with the treated time increasing, the surface of the samples treated by subcritical water became rougher (–). For subcritical water pretreated zein with different temperatures, the surface not only became rough but also appeared some small micro-holes (highlighted by black rings in the and ). This phenomenon became more and more obvious with the increase of temperature, which was similar to that of reported literature.[Citation40]
Figure 4. Effect of subcritical water treatment on the physical structure of zein protein. Scanning electron microscopy images of untreated zein protein (a) and subcritical water-treated zein (b–f). Subcritical water treatment conditions: treatment sample 0.5 g/30 mL; subcritical water treatment times: 20 min (b) and 60 min (c) and 100 min (d). Subcritical water treatment temperatures: 130°C (e) and 170°C (f). And all magnifications are ×5,000.
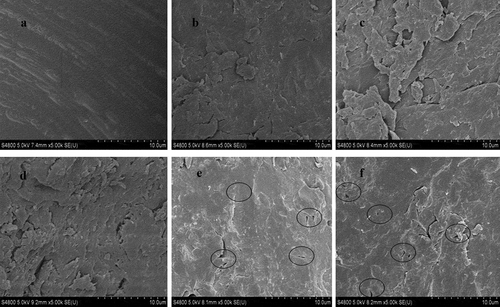
In order to better identify the difference between the surfaces of the zein samples, AFM measurements were also carried out. When the AFM scans the sample, the information of the force distribution can be obtained from the change of sensor detector, so as to obtain the information of surface morphology and surface roughness.
The structural appearance of the untreated zein showed a dense network structure composed of many doughnut-like structures (highlighted by black rings in ), which was related to the film-formation of zein.[Citation41] With the extension of time, the network structure of zein was gradually opened. From the top point of view, the surface of the sample became a dispersed sphere. Moreover, zein particles became more and more large, which was consistent with the determination of particle size of zein (–). At the same time, the network structure of zein protein was also opened with the increase of heating temperature ( and ). Also, the particle size of zein protein became larger and larger, which was also consistent with our previous conclusions.
Figure 5. Effects of subcritical water treatment on the atomic force microscopy (AFM). AFM images of untreated zein (a) and subcritical water-pretreated zein (b–f). Subcritical water treatment conditions: treatment sample (0.5 g/30 mL); subcritical water treatment time, 20 min (b), 60 min (c), and 100 min (d). Subcritical water-treated temperature 130°C (e) and 170°C (f).
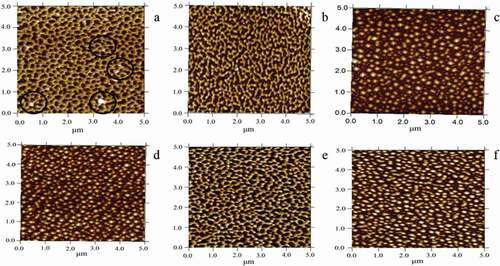
Conclusion
The subcritical water treatment was an effective method to improve structural and functional properties of zein protein. Compared with the untreated zein samples, solubility, FC, and FS were increased by subcritical water pretreatment. PSA, DSC, CD, SEM, and AFM analysis showed that subcritical water treatment not only improved the mean particle size, thermal stability, and the denaturation temperature of zein protein but also slightly increased the percentage of ordered structural elements within the protein molecule. The surface of zein became rough significantly. These findings may be relevant information to think over when attempting to use subcritical water pretreatment for the improvement of functional properties.
Acknowledgment
The authors thank the Postgraduate Research & Practice Innovation Program of Jiangsu Province, China (KYCX17_1799).
Additional information
Funding
References
- Galkin, A. A.; Lunin, V. V. Subcritical and Supercritical Water: A Universal Medium for Chemical Reactions. Russian Chemical Reviews 2005, 74(1), 21–35. DOI: 10.1070/RC2005v074n01ABEH001167.
- Herrero, M.; Cifuentes, A.; Ibañez, E. Sub-And Supercritical Fluid Extraction of Functional Ingredients from Different Natural Sources: Plants, Food-By-Products, Algae and Microalgae: A Review. Food Chemistry 2006, 98(1), 136–148. DOI: 10.1016/j.foodchem.2005.05.058.
- Gamiz-Gracia, L.; De Castro, M. D. L. Continuous Subcritical Water Extraction of Medicinal Plant Essential Oil: Comparison with Conventional Techniques. Talanta. 2000, 51(6), 1179–1185. DOI: 10.1016/S0039-9140(00)00294-0.
- Hawthorne, S. B.; Grabanski, C. B.; Martin, E.; Miller, D. J. Comparisons of Soxhlet Extraction, Pressurized Liquid Extraction, Supercritical Fluid Extraction and Subcritical Water Extraction for Environmental Solids: Recovery, Selectivity and Effects on Sample Matrix. Journal of Chromatography A 2000, 892(1–2), 421–433. DOI: 10.1016/S0021-9673(00)00091-1.
- Ibañez, E.; Kubátová, A.; Señoráns, F. J.; Cavero, S.; Reglero, G.; Hawthorne, S. B. Subcritical Water Extraction of Antioxidant Compounds from Rosemary Plants. Journal of agricultural and food chemistry 2003, 51(2), 375–382. DOI: 10.1021/jf025878j.
- Rogalinski, T.; Liu, K.; Albrecht, T.; Brunner, G. Hydrolysis Kinetics of Biopolymers in Subcritical Water. The Journal of Supercritical Fluids 2008, 46(3), 335–341. DOI: 10.1016/j.supflu.2007.09.037.
- Sereewatthanawut, I.; Prapintip, S.; Watchiraruji, K.; Goto, M.; Sasaki, M.; Shotipruk, A. Extraction of Protein and Amino Acids from Deoiled Rice Bran by Subcritical Water Hydrolysis. Bioresource technology 2008, 99(3), 555–561. DOI: 10.1016/j.biortech.2006.12.030.
- Yang, Y.; Bowadt, S.; Hawthorne, S. B.; Miller, D. J. Subcritical Water Extraction of Polychlorinated Biphenyls from Soil and Sediment. Analytical Chemistry 1995, 67(24), 4571–4576. DOI: 10.1021/ac00120a022.
- Yoshida, H.; Terashima, M.; Takahashi, Y. Production of Organic Acids and Amino Acids from Fish Meat by Sub‐Critical Water Hydrolysis. Biotechnology progress 1999, 15(6), 1090–1094. DOI: 10.1021/bp9900920.
- Thiruvenkadam, S.; Zither, S.; Yoshida, H.; Danquah, M. K.; Harun, R. Process Application of Subcritical Water Extraction (SWE) for Algal Bio-Products and Biofuels Production. Applied Energy 2015, 154, 815–828. DOI: 10.1016/j.apenergy.2015.05.076.
- Ko, M. J.; Cheigh, C. I.; Chung, M. S. Relationship Analysis between Flavonoids Structure and Subcritical Water Extraction (SWE). Food chemistry 2014, 143, 147–155. DOI: 10.1016/j.foodchem.2013.07.104.
- Wang, Z.; Deng, S.; Gu, Q.; Cui, X.; Zhang, Y.; Wang, H. Subcritical Water Extraction of Huadian Oil Shale under Isothermal Condition and Pyrolysate Analysis. Energy & Fuels 2014, 28(4), 2305–2313. DOI: 10.1021/ef5000062.
- Reddy, H. K.; Muppaneni, T.; Sun, Y.; Li, Y.; Ponnusamy, S.; Patil, P. D.; Dailey, P.; Schaub, T.; Holguin, F. O.; Dungan, B.; et al. Subcritical Water Extraction of Lipids from Wet Algae for Biodiesel Production. Fuel 2014, 133, 73–81. DOI: 10.1016/j.fuel.2014.04.081.
- Khajenoori, M.; AslA, H.; Epicene, M. H. Subcritical Water Extraction of Essential Oils from Trachyspermumammi Seeds. Journal of Essential Oil Bearing Plants 2015, 18(5), 1165–1173. DOI: 10.1080/0972060X.2013.831565.
- Luo, X.; Duan, Y.; Yang, W.; Zhang, H.; Li, C.; Zhang, J. Structural Elucidation and Immunostimulatory Activity of Polysaccharide Isolated by Subcritical Water Extraction from Cordyceps Militaris. Carbohydrate Polymers 2017, 157, 794–802. DOI: 10.1016/j.carbpol.2016.10.066.
- Zakaria, S. M.; Kamal, S. M. M. Subcritical Water Extraction of Bioactive Compounds from Plants and Algae: Applications in Pharmaceutical and Food Ingredients. Food Engineering Reviews 2016, 8(1), 23–34. DOI: 10.1007/s12393-015-9119-x.
- Tangkhavanich, B.; Kobayashi, T.; Adachi, S. Properties of Rice Straw Extract after Subcritical Water Treatment. Bioscience, biotechnology, and biochemistry 2012, 76(6), 1146–1149. DOI: 10.1271/bbb.110983.
- Chen, X. W.; Wang, J. M.; Yang, X. Q.; Qi, J.-R.; Hou, -J.-J. Subcritical Water Induced Complexation of Soy Protein and Rutin: Improved Interfacial Properties and Emulsion Stability. Journal of Food Science 2016, 81(9), C2149–C2157. DOI: 10.1111/1750-3841.13403.
- Lu, W.; Chen, X. W.; Wang, J. M.; Yang, X.-Q.; Qi, J.-R. Enzyme-Assisted Subcritical Water Extraction and Characterization of Soy Protein from Heat-Denatured Meal. Journal of Food Engineering 2016, 169, 250–258. DOI: 10.1016/j.jfoodeng.2015.09.006.
- Zhang Q. T., Tu Z. C., Wang H.; Huang, X.; Fan, L.; Bao, Z.; Xiao, H. Functional Properties and Structure Changes of Soybean Protein Isolate after Subcritical Water Treatment. Journal of food science and technology 2015, 52(6), 3412–3421. DOI: 10.1007/s13197-014-1392-9.
- Shukla, R.; Cheryan, M. Zein: The Industrial Protein from Corn. Industrial crops and products 2001, 13(3), 171–192. DOI: 10.1016/S0926-6690(00)00064-9.
- Rees, E. D.; Singer, S. J. A Preliminary Study of the Properties of Proteins in Some Nonaqueous Solvents. Archives of biochemistry and biophysics 1956, 63(1), 144–159. DOI: 10.1016/0003-9861(56)90018-2.
- Smith, B. M.; Bean, S. R.; Selling, G.; Sessa, D.; Aramouni, F. M. Role of Non-Covalent Interactions in the Production of Visco-Elastic Material from Zein. Food chemistry 2014, 147, 230–238. DOI: 10.1016/j.foodchem.2013.09.152.
- Ren, X.; Ma, H.; Mao, S.; Zhou, H. Effects of Sweeping Frequency Ultrasound Treatment on Enzymatic Preparations of ACE-inhibitory Peptides from Zein. European Food Research and Technology 2014, 238(3), 435–442. DOI: 10.1007/s00217-013-2118-3.
- Ayala, R. S.; De Castro, M. D. L. Continuous Subcritical Water Extraction as a Useful Tool for Isolation of Edible Essential Oils. Food chemistry 2001, 75(1), 109–113. DOI: 10.1016/S0308-8146(01)00212-6.
- Rodríguez-Meizoso, I.; Marin, F. R.; Herrero, M.; Señorans, F. J.; Reglero, G.; Cifuentes, A.; Ibáñez, E. Subcritical Water Extraction of Nutraceuticals with Antioxidant Activity from Oregano. Chemical and Functional Characterization. Journal of Pharmaceutical and Biomedical Analysis 2006, 41(5), 1560–1565. DOI: 10.1016/j.jpba.2006.01.018.
- Singh, P. P.; Saldaña, M. D. A. Subcritical Water Extraction of Phenolic Compounds from Potato Peel. Food Research International 2011, 44(8), 2452–2458. DOI: 10.1016/j.foodres.2011.02.006.
- Ledoux, M.; Lamy, F. Determination of Proteins and Sulfobetaine with the Folin-Phenol Reagent . Bioresource technology 1986, 157(1), 28–31. DOI: 10.1016/0003-2697(86)90191-0.
- Zhao, X.; Zhang, X.; Liu, H.; Zhang, G.; Ao, Q. Functional, Nutritional and Flavor Characteristic of Soybean Proteins Obtained through Reverse Micelles. Food Hydrocolloids 2018, 74, 358–366. DOI: 10.1016/j.foodhyd.2017.08.024.
- Sreerama, N.; Woody, R. W. Estimation of Protein Secondary Structure from Circular Dichroism Spectra: Comparison of CONTIN, SELCON, and CDSSTR Methods with an Expanded Reference Set. Analytical biochemistry 2000, 287(2), 252–260. DOI: 10.1006/abio.2000.4880.
- Lobley, A.; Whitmore, L.; Wallace, B. A. DICHROWEB: An Interactive Website for the Analysis of Protein Secondary Structure from Circular Dichroism Spectra. Bioinformatics 2002, 18(1), 211–212. DOI: 10.1093/bioinformatics/18.1.211.
- Whitmore, L.; Wallace, B. A. DICHROWEB, an Online Server for Protein Secondary Structure Analyses from Circular Dichroism Spectroscopic Data. Nucleic acids research 2004, 32(suppl 2), W668–W673. DOI: 10.1093/nar/gkh371.
- Guo, Y.; Liu, Z.; An, H.; Li, M.; Hu, J. Nano-Structure and Properties of Maize Zein Studied by Atomic Force Microscopy. Journal of Cereal Science 2005, 41(3), 277–281. DOI: 10.1016/j.jcs.2004.12.005.
- Sunphorka, S.; Chavasiri, W.; Oshima, Y.; Ngamprasertsith, S. Kinetic Studies on Rice Bran Protein Hydrolysis in Subcritical Water. The Journal of Supercritical Fluids 2012, 65, 54–60. DOI: 10.1016/j.supflu.2012.02.017.
- Pu, Y.; Li, Y.; Wang, D.; Foster, N. R.; Wang, J.-X.; Chen, J.-F. A Green Route to Beclomethasone Dipropionate Nanoparticles via Solvent Anti-Solvent Precipitation by Using Subcritical Water as the Solvent. Powder Technology 2017, 308, 200–205. DOI: 10.1016/j.powtec.2016.12.019.
- Khuwijitjaru, P.; Nualchan, P.; Adachi, S. Foaming and Emulsifying Properties of Rice Bran Extracts Obtained by Subcritical Water Treatment. Silpakorn University Science and Technology Journal 2007, 1(1), 7–12.
- Ma, C. Y.; Harwalkar, V. R. Thermal Analysis of Food Proteins . Advances in food and nutrition research 1991, 35, 317–366.
- Abdelmoez, W.; Yoshida, H. Synthesis of a Novel Protein-Based Plastic Using Sub-Critical Water Technology. AIChE journal 2006, 52(7), 2607–2617. DOI: 10.1002/(ISSN)1547-5905.
- Pińkowska, H.; Oliveros, E. Application of the Doehlert Matrix for the Determination of the Optimal Conditions of Hydrolysis of Soybean Protein in Subcritical Water. Industrial & Engineering Chemistry Research 2014, 53(4), 1320–1326. DOI: 10.1021/ie403451b.
- Deng H.; Zhang Z.; Liu, J.; Gu J. Research on the Calculation Method of Pressure Field Caused by the Ship Moving at Subcritical Speed in Shallow Water. Applied Mathematics and Mechanics 2013, 34(8), 846–853. DOI: 10.4028/www.scientific.net/AMM.316-317.846.
- King, B. L.; Taylor, J.; Taylor, J. R. N. Formation of a Viscoelastic Dough from Isolated Total Zein (α-, β-and γ-Zein) Using a Glacial Acetic Acid Treatment. Journal of Cereal Science 2016, 71, 250–257. DOI: 10.1016/j.jcs.2016.09.005.