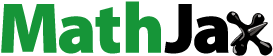
ABSTRACT
The functional and structural properties of enzymatic hydrolysis and glycosylation synergistic modified ovalbumin (OVA) were investigated. The results showed that hydrolysis and glycosylation had synergetic effects on emulsifying and foaming properties, water and oil-binding capacity of OVA. And hydrolysis and glycosylation also could improve gel strength and thermal properties of OVA. Polyacrylamide gel electrophoresis analysis implied that the formation of 20.1 kDa ~ 29 kDa Maillard reaction products (MRPs). UV absorption and Fourier Transform Infrared spectroscopy analyses confirmed that enzymatic hydrolysis and glycosylation could change the secondary structure of OVA. Moreover, hydrolysis and glycosylation could produce OVA with compact and orderly network structure.
Introduction
Egg white protein (EWP) is widely used as a good source of dietary proteins in the food industry, due to its nutritional value, functional properties, and availability.[Citation1,Citation2] Ovalbumin (OVA) is the main constituent of EWP and most widely used model protein.[Citation3] It is an important food ingredient, which is frequently used as a surfactant protein in the food industry to improve and maintain the quality (texture and volume) of aerated food.[Citation4] Much research has been conducted in order to improve the functional properties of OVA,[Citation5] including phosphorylation,[Citation6,Citation7] glycosylation,[Citation8–Citation10] enzymatic modification,[Citation11] and high pressure.[Citation5] Compared to other modification methods, glycosylation is an effective method to improve the food—use functional properties of OVA.[Citation3,Citation9,Citation12] Nevertheless, some research showed that the glycosylation of OVA with other polysaccharides is not economical, which is bound to limit the application of the OVA and polysaccharides conjugates in food industry.[Citation9] Therefore, the search for an economic and effective modification method is of significance.
Enzymatic hydrolysis also has been demonstrated to be an effective method to improve the functional properties of protein[Citation11] because of its high specificity, controllable and minimal formation of by-products.[Citation13] Limited enzymatic hydrolysis could reduce molecular weight, but exposured hydrophobic groups previously buried in the protein,[Citation14–Citation16] which might increase the protein foaming capacity and emulsifying properties. However, to the best of our knowledge, most papers have been reported to use enzymatic hydrolysis to produce EWP peptides[Citation17–Citation19] or reduce its allergenicity,[Citation11] few reports about using enzymatic hydrolysis to improve the functional properties of EWP.
Therefore, based on the knowledge of enzymatic hydrolysis and glycosylation, we hypothesized that the combination of enzymatic hydrolysis and glycosylation might improve the functional properties of OVA. Thus, the aim of the present work was to study how enzymatic hydrolysis of OVA followed by glycosylation affect the functional properties of OVA. Structural properties were also studied to evaluate a proper modification method to obtain OVA with desirable functional properties.
Materials and methods
Materials
Ovalbumin (OVA, A5378, purity as 80–90%, molecular weight as 45 kDa) was purchased from Sigma. Commercial alkaline protease (1.0 × 105 U/g) was obtained from Shanghai Blue Season Of Science and Technology Development Co., Ltd. Acrylamide was purchased from Beijing Baiao Leibo Technology Co., Ltd. Sodium dodecyl sulfate and Tetramethyl ethylenediamine purchased from Tianjin Kemiou Chemical Reagent Co., Ltd. Glucose was acquired from local market. All other chemical agents were of analytical grades.
Methods
Limited hydrolysis of OVA followed by glycosylation: OVA powder was dissolved in distilled water at room temperature and kept under stirring at 100 rpm for 1 h to prepare 5% (w/v) OVA solution. The pH of protein solution was adjusted to 8.25 using 0.1 M NaOH and HCl. The amount of alkaline protease (5500 U/g protein) was added to OVA solution and the mixture was kept at 55°C for 5 h. The pH of the mixture was maintained at the desired value by the continuous addition of 0.1 M NaOH during the reaction. Then the mixture was centrifuged at 4500 r/min for 10 min using a centrifuge (TDL-5-A, Anke, Shanghai, China) at room temperature. The liquid supernatant was freeze-dried, named as hydrolyzed OVA. The hydrolyzed OVA (8%, w/v) was mixed with glucose at a weight ratio of 1:4 in the disstilled water. The resulting mixture was freeze-dried and followed by incubating at 50°C for three days in a desiccator containing saturated KBr solution (79% relative humidity), then mixture was dialyzed (5000 Da of dialysis bag) and freeze-dried, and named as modified OVA.
Functional properties of the modified OVA
Emulsifying properties: Emulsifying properties of protein samples were determined according to the method of Huang et al.[Citation20] with slight modifications. Protein solution (1%, w/v) was prepared by dissolving dry protein in phosphate buffer (pH 7.0) at room temperature. Vegetable oil (5 mL) and protein solution (15 mL) was homogenized using a homogenizer at 10000 rpm for 1 min. Emulsion (0.1 mL) was pipetted out at 0 min and 10 min, and 100 folds diluted with 0.1% sodium dodecyl sulfate solution, respectively. The absorbance was measured at 500 nm using spectrophotometer (Model UV-3200, Mapada, Shanghai, China). The emulsifying activity index (EAI) and emulsion stability index (ESI) were calculated by the following equations:
where, DF = dilution factor (100), l = path length of cuvette (m), φ = oil volume fraction, and C = protein concentration in aqueous phase (g/m3)
where, A0 = A500 at time of 0 min, A10 = A500 at time of 10 min, and Δt = 10 min.
Foaming properties: The foaming properties of protein solution were evaluated by the method of Liu et al.[Citation5] with some modifications. The samples (70 mL) were homogenized by a homogenizer at 6000 rpm for 2 min. Then the foaming solution was poured into a 50 mL graduated cylinder immediately. The total volume was taken at 0 min for foaming ability. The volume was recorded again at 30 min for foaming stability. Foaming ability and foaming stability were calculated as follows:
where V0 is the foam volume at 0 min, V1 is the foam volume at 30 min.
Water binding capacity (WBC) and oil binding capacity (OBC): The WBC and OBC of OVA were measured according to the method of proposed by Lv et al.[Citation21] with slight modification. Brifely, 0.5 g OVA samples (W0) were dispersed in 6 mL of deionized water or soybean oil in a pre-weighed 15 mL centrifuge tube using a vortex mixer. Samples were left to stand at 30°C for 30 min, and the volume was recorded as V1. The dispersion was centrifuged at 3000 g for 20 min. The supernatant was carefully poured into a 10 mL graduated cylinder, and the volume was recorded as V2. WBC or OBC (mL of oil or water per gram of protein) was calculated from the Equation (5).
Gel strength: The gel strength of OVA samples were measured according to the method of Huang et al.[Citation22] using the texture analyzer (Stable Micro System, Surrey, U.K) with some modifications. The pH of protein suspensions (10%, w/v) were adjusted to 7.0 using 0.1 mmol/L NaOH or 0.1 mmol/L HCl. Then 20 mL of protein samples were poured into 25 mL of glass beaker and heated at 90°C for 30 min in a water bath and maturated at 4°C for 24 h. Gel strength of protein gels samples were measured using texture analyzer was equipped with p0.5 probe, defined the force of penetration distance reached 10 mm as gel strength.
Differential scanning calorimetry (DSC): DSC measurements were perormed using a differential scanning calorimeter (PE Co., USA).[Citation5] Protein samples (10 mg) were placed inside an aluminum pan, sealed tightly, and scaned from 30 to 150°C at a rate of 10°C/min. An empty sealed aluminum pan was used as a reference.
Structural characteristics of OVA
SDS-PAGE: SDS-PAGE was performed to estimate the changes in molecular weights of OVA.[Citation10] The 12% separating gels and 5% stacking gels were subjected to electrophoresis at a constant current of 15 mA/gel using a Mini Protean II unit (Bio-Rad Laboratories, Hercules, CA). Protein bands were stained with Coomassie brilliant Blue R250, and molecular weight were evaluated using Thermo middle molecular weight protein markers (10–180 kDa).
UV absorption spectrum: UV absorption spectrum analysis was performed using a UV-1800 spectrophotometer.[Citation10] OVA (0.01%, w/v) solution was prepared by dissolving protein in 50 mmoL/L Tris - HCl buffer (pH 7.4). The UV absorption spectrum were scanned from 200 to 400 nm.
Fourier transform infrared spectroscopy (FTIR): The spectra of freezing-dried samples were recorded using a Fourier transform infrared spectrometer (NEXUS-470, Thermol Nicolet, USA). Dried protein samples (4 mg) and KBr powder (120 mg) were mixed uniformly and pressed into slice. Spectra were acquired for 32 times in the IR range of 4000–400 cm−1 with a resolution of 4 cm−1 at 25°C.[Citation23]
Scanning electron microscopy: The dried samples were mounted on conducting resin with 2–3 mm thickness.[Citation24] The morphology of OVA was observed using an Ultra Plus scanning electron microscope (Zeiss, Oberkochen, Germany).
Statistics analysis
All measurements were performed in triplicate to obtain mean values ± standard deviation. The results were analyzed using SPSS (17.0, SPSS Inc., Chicago, Illinois, USA) with Duncan’s multiple range test at significant level of p < 0.05.
Results and discussions
Functional properties of OVA
Emulsifying and foaming properties: Proteins have a lot of polar and non-polar groups and could be absorbed between the dispersed oil phase and the continuous water phase to form stable emulsions.[Citation25] As shown in ), hydrolyzed OVA exhibited higher values of EAI and ESI than those of OVA. These suggested that enzymatic hydrolysis could stretch the hydrophilic groups of OVA to the aqueous phase, increasing the protein molecular hydrophilic oil-wet equilibrium value, resulting in the enhancements of emulsifying properties. In comparsion to OVA and hydrolyzed OVA, the EAI of modified OVA increased by 4.9 m2/g and 18.46 m2/g, and the ESI increased by 1.97% and 12.43%, respectively. On the one hand, covalent attachment of glucose to OVA formed better amphiphilic balance, probably due to the formation of a macromolecular stabilizing film around oil droplets.[Citation25,Citation26] On the other hand, glycosylation produced conjugated polymer can significantly retard the thinning process of the continuous film through steric hindrance and contribution to the formation of a macromolecular stabilizing film around oil droplets.[Citation27] Generally, lysine and arginine residues are the main two glycation sites of protein during the Maillard reaction.[Citation27] Enzymatic hydrolysis might exposure more lysine and arginine residues in the protein, contributing to the glycosylation. Furthermore, some researchers also showed that Maillard reaction could improve the emulsifying properties of protein owing to increment protein solubility, due to fast migration and adsorption on the interface of water/oil to form a film around the oil droplets.[Citation21,Citation28,Citation29] Furthermore, it is interesting to state that changements of conformation of protein by glycosylation and presence of non-protein constituents which can interfere with emulsion formation also have effects on the emulsifying properties. In conclusion, compared to OVA and hydrolyzed OVA, the modified OVA showed most excellent emulsifying properties.
Foaming properties express the interfacial area stabilized per unit weight of protein, which characterizes the ability of a protein to be absorbed to the air-water interface.[Citation5] As shown in ), hydrolyzed OVA and modified OVA both showed higher FA and FS than those of native OVA. In contrast to native OVA and hydrolyzed OVA, the FA of modified OVA increased by 44.42% and 80.98%, respectively. This might because that enzymatic hydrolysis lead more unfolding of the proteins, enhanced the hydrophilicity of OVA during the process of whipping and foaming. Glycosylation might induce hydrophilic groups to protein, improving its solubility.[Citation28,Citation30] Furthermore, when sugar molecules bind to proteins, they make the partially unfolded states of proteins, leading to the enhancement of hydrophobic interaction on the protein surface. The hydrophobic interaction may improve the foam formation.[Citation31] Glycosylation may make OVA be able to form stronger continuous viscoelastic membrane-like film around air cells than native OVA. In general, OVA act as a weak amphiphilic emulsifier between the air and the aqueous phase. The quality of protein-based foams depends on the conformational characteristics of proteins.[Citation32] Glycosylation could change conformation structure of OVA, endowing its high quality of emulsifier property.[Citation27]
Water and oil binding capacity: For the food system, WBC of protein is related to the ability of food binding water, which can affect food quality, such as tenderness, juiciness, softness. Intrinsic factors affecting water binding capacity of food proteins include amino acid composition, protein conformation, surface polarity/hydrophobicity.[Citation21,Citation33] As shown in , the modified OVA exhibited highest WBC (5.82 g/g) than those of hydrolyzed OVA (3.64 g/g) and native OVA (1.56 g/g). Enzymatic hydrolysis might expand molecular structure of OVA, forming a loose three-dimensional network structure to intercept more water molecules (), improving its WBC. The further improvement in WBC of modified OVA might have been caused by the exposure of additional binding sites and the production of MRPs were able to bind more water.[Citation21] OBC is another important functional property of protein, and widely used in the meat and confectionery products.[Citation21] The modified OVA also possessed highest OBC (6.83 mL/g) than those of hydrolyzed OVA (4.35 mL/g) and native OVA (3.53 mL/g). The improvement in OBC may have been caused by the balance of hydrophilic and hydrophobic groups of modified OVA.[Citation21,Citation33] Therefore, both enzymatic hydrolysis and glycosylation could improve the WBC and OBC of OVA.
Table 1. Three evaluated indices of OVA, hydrolyzed OVA and modified OVA.
Figure 3. SDS-PAGE of OVA before and after hydrolyzed and glycosylated modification. Lane 1, ovalbumin; Lane 2, hydrolyzed ovalbumin; Lane 3, modified ovalbumin; Lane Mark, standard protein markers.
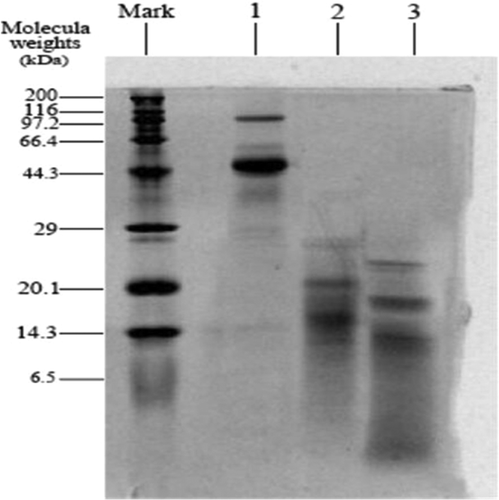
Figure 5. Scanning electron microscopy image of OVA: (a) native OVA (300×), (b) hydrolyzed OVA (100×), (c) modified OVA (100×), (d) modified OVA (500×).
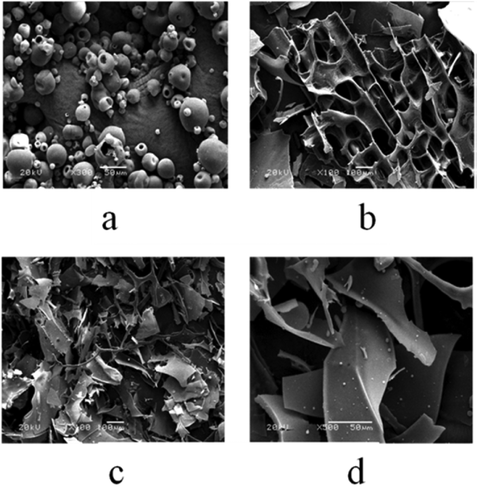
Gel strength: Gel formation of protein imparts desirable textural and sensory attributes to foods.[Citation22] As shown in , the modified OVA possessed highest gel strength than those of hydrolyzed OVA and OVA. Enzymatic may improve the solubility of OVA making more protein molecules are available for participation in the gelation process. This may explain why hydrolyzed OVA possessed higher gel strength than native OVA. Furthermore, glycosylation could affect the space network structure of the protein, which was similar with Sun et al.[Citation34] reported that glycosylation could improve the gelling properties of ovalbumin.
DSC: DSC technique is widely used to study thermal transitions of food proteins, providing information on the modification of native protein to its heat-denatured state.[Citation35] As shown in , the thermal denaturation temperature of OVA was 65.11°C, while hydrolyzed OVA and modified OVA were 71.8 and 77.5°C, respectively. These results are in agreement with the data shown previously for the WBC, OBC, and gel strength. The thermal stability of the protein is associated with its amino acid composition, protein has high content of hydrophobic amino acids usually presents better thermal stability.[Citation36] Enzymatic hydrolysis could break the globular structure of OVA, increase hydrophobic groups of protein and show higher thermal stability. After glycation, thermal denaturation temperature increased, which indicates the enhanced resistance against thermal denaturation after glycation. This was simialr with Kim et al.[Citation35] demonstrated that BSA–fucoidan conjugates are more resistant to thermal treatments. It also has been reported that thermal denaturation of proteins involved conformational changes of native structure and led to the disruption of chemical forces that maintain the structure of the protein molecules.[Citation37] Heat treatment may damage the protein, resulting in loss of protein structure and precipitation, as well as causing a decrease in protein nutritional value.[Citation38] Therefore, the combination of hydrolysis and glycosylation modification is a good method to guarantee quality of food products.
Structural properties of OVA
SDS-PAGE: As shown in , native OVA possessed high molecular weight over 44.3 kDa. While, the main molecular weights of hydrolyzed OVA were ranged from 20.1 kDa to 14.3 kDa, and the band was shallow. This indicated the hydrolysis efficiency of proteins. The high-molecular-weight polymer in OVA were rapidly hydrolysed, producing small amounts of low molecular weight polymer. This endopeptidase enzyme could influence the quaternary and tertiary conformations of proteins by cleaving peptide bonds within individual or aggregated proteins to produce smaller protein sub-units and/or smaller peptides.[Citation14] Glycosylation could produce Maillard reaction products (MRPs) of hydrolyzed OVA, which were between 20.1 kDa and 29 kDa. It has been widely demonstrated that these MRPS provide better emulsifying capability, heat stability, foaming, solubility, as well as reduce allergenicity compared to native protein.[Citation9,Citation27]
UV: The UV absorbance of protein in the near-UV region depends on the residues of the aromatic amino acids, including tryptophan, tyrosine and phenylalanine, with tryptophan being mainly responsible for the near-UV region. The effect of hydrolysis and glycosylation on the UV spectra of OVA is shown in ). The maximum UV absorption peak of OVA was around 280 nm, indicating that OVA contained tyrosine (Tyr) and tryptophan (Trp) residue side chain groups.[Citation39] While, hydrolysis and glycosylation made the UV absorption peak of OVA red shift, indicating the Trp and Tyr residue side groups of OVA had been changed. Compared to native OVA, hydrolyzed OVA usually had higher absorption intensity, suggesting that tryptophan residues were gradually exposed to the surface of protein molecule. Moreover, modified OVA showed highest absorption intensity, demonstrating that the production of MRPs.[Citation27,Citation38] UV absorption spectroscopy indicated that enzymatic hydrolysis and glycosylation could change the structural properties of OVA.
FTIR: FTIR spectroscopy has been usually used to study the functional groups and secondary structure of protein samples. Amide I, amide II, and amide III corresponding to the polar functional groups, such as C = O and OH, were mainly used for protein conformational analysis.[Citation5]
The amide I band (1700–1600 cm−1) is the most important for infrared spectroscopic analysis of the secondary structure of proteins.[Citation40] Amide-I represents C = O stretching vibration hydrogen bonding coupled with COO, coupled to contributions from the CN stretch, CCN deformation and in-plane NH bending mode.[Citation40,Citation41] As shown in ), the amide I absorption peaks of native OVA, hydrolyzed OVA and modified OVA were 1660.2, 1654, and 1651 cm−1, respectively. According to some researchers works,[Citation40,Citation42] modified OVA had highest contents of random coils and β-turns. This suggested that the consumption of amino groups and the formation of Schiff bases due to the Maillard reaction.[Citation42] The amide II band in the infrared spectrum of protein is in the range of 1600–1500 cm−1[Citation5] which primarily represent an out-of- phase combination of CN stretch and in-plane NH deformation modes of the peptide group.[Citation41] In this study, the characteristic absorption value of modified OVA in amide-II region was slightly highest than those of native and hydrolyzed OVA (data not shown). It has also reported that Amide A band of the infrared spectra of polymer is observed at an unusually high frequency (3400–3300 cm−1), which was related to hydrogen bond stretching and the vibration of OH groups.[Citation39,Citation40] The absorption peak of amide A will appear at a lower wavenumber when the NH group of a peptide is involved in especially lower and stronger hydrogen bond.[Citation5] As shown in ), the Amide A wavenumbers of native OVA, hydrolyzed OVA and modified OVA were 3330, 3325.1, 3321.8 cm−1 respectively. The effects of hydrolysis degraded protein might undergo hydrogen bonding interaction, resulting in decreased wavenumbers of amide-A.[Citation41] Glycated protein could be converted to high-molecular-weight conjugates owing to the generation of protein–saccharide crosslinking reactions., decreasing amide-A wavenumbers.[Citation43] These results implied that the secondary structures of OVA could be changed by the hydrolysis and Maillard reaction.
SEM: As shown in ), OVA is a typical globulin protein. Enzymatic hydrolysis could damage spherocal structure of OVA, forming a compact and orderly network structure with many pores (). This could explain why hydrolyzed OVA showed higher gel strength than that of native OVA. While, compared to hydrolyzed OVA, the lump of modified OVA ( and ) is smaller, which increases the contact area with water and oil, result in the improvement of functional properties of proteins, such as foaming and emulsifying properties, WBC and OBC capacities and gel strength.[Citation21]
Conclusion
This present work showed that the functional properties of OVA could be effectively improved by the synergistic effects of alkaline protease hydrolysis and glycosylation. The results showed that enzymatic hydrolysis could improve the functional properties of OVA, and glycosylation could significantly improve emulsifying and foaming properties of OVA, and endowed OVA highest gel strength, WBC, OBC, and thermal stability. These might because the hydrolysis of OVA exposured more glycation sites of protein, contributing to the formation of new MRPs through glycosylation, resulting in changing secondary structure of the OVA and forming ordered structure. Therefore, the combination of hydrolysis and glycosylation could be used as a potential method to modify protein to produce high-quality protein powder. Otherwise, more works about the modified OVA should be done, such as rheological behavior, to evaluated its applicability information in the food industry.
Acknowledgement
This study was supported by Special Fund for Agro-scientific Research in the Public Interest of China (No. U1704114, 201303084), Nation Natural Science Foundation of China (No. 31401622) and Natural Science Research Program of Education Department of Henan Province (No. 13A550255).
Additional information
Funding
References
- Huang, T.; Tu, Z.-C.; Wang, H.; Shangguan, X. Promotion of Foam Properties of Egg White Protein by Subcritical Water Pre-Treatment and Fish Scales Gelatin. Colloids and Surfaces A: Physicochemical and Engineering Aspects 2017, 512, 171–177. DOI: 10.1016/j.colsurfa.2016.10.013.
- Sadahira, M. S.; Lopes, F. C. R.; Rodrigues, M. I.; Yamada, A. T. Effect of pH and Interaction between Egg White Protein and Hydroxypropymethylcellulose in Bulk Aqueous Medium on Foaming Properties. Carbohydrate Polymers 2015, 125, 26–34. DOI: 10.1016/j.carbpol.2015.02.033.
- Ma, X.-J.; Gao, J.-Y.; Chen, H.-B. Combined Effect of Glycation and Sodium Carbonate–Bicarbonate Buffer Concentration on IgG Binding, IgE Binding and Conformation of Ovalbumin. Journal of the Science of Food and Agriculture 2013, 93, 3209–3215. DOI: 10.1002/jsfa.2013.93.issue-13.
- Jia, W.; Cui, B.; Ye, T.; Lin, L. Phase Behavior of Ovalbumin and Carboxymethylcellulose Composite System. Carbohydrate Polymers 2014, 109, 64–70. DOI: 10.1016/j.carbpol.2014.03.026.
- Liu, G.-X.; Tu, Z.-C.; Wang, H.; Zhang, L. Monitoring of the Functional Properties and Unfolding Change of Ovalbumin after DHPM Treatment by HDX and FTICR MS: Functionality and Unfolding of Oval after DHPM by HDX and FTICR MS. Food Chemistry 2017, 227, 413–421. DOI: 10.1016/j.foodchem.2017.01.109.
- Xiong, Z.; Zhang, M.; Ma, M. Emulsifying Properties of Ovalbumin: Improvement and Mechanism by Phosphorylation in the Presence of Sodium Tripolyphosphate. Food Hydrocolloids 2016, 60, 29–37. DOI: 10.1016/j.foodhyd.2016.03.007.
- Xiong, Z.; Ma, M.; Jin, G.; Xu, Q. Effects of Site-Specific Phosphorylation on the Mechanical Properties of Ovalbumin-Based Hydrogels. International Journal of Biological Macromolecules 2017, 102, 1286–1296. DOI: 10.1016/j.ijbiomac.2017.05.028.
- An, Y.; Cui, B.; Wang, Y.; Jin, W. Functional Properties of Ovalbumin Glycosylated with Carboxymethyl Cellulose of Different Substitution Degree. Food Hydrocolloids 2014, 40, 1–8. DOI: 10.1016/j.foodhyd.2014.01.028.
- Tu, Z.-C.; Hu, Y.-M.; Wang, H.; Huang, X.-Q. Microwave Heating Enhances Antioxidant and Emulsifying Activities of Ovalbumin Glycated with Glucose in Solid-State. Journal of Food Science and Technology 2015, 52, 1453–1461. DOI: 10.1007/s13197-013-1120-x.
- Yang, W.-H.; Tu, Z.-C.; Wang, H.; Li, X.; Tian, M. High‐Intensity Ultrasound Enhances the Immunoglobulin (Ig) G and IgE Binding of Ovalbumin. Journal of the Science of Food and Agriculture 2017, 97, 2714–2720. DOI: 10.1002/jsfa.2017.97.issue-9.
- Yang, A.; Long, C.; Xia, J.; Tong, P. Enzymatic Characterisation of the Immobilised Alcalase to Hydrolyse Egg White Protein for Potential Allergenicity Reduction. Journal of the Science of Food and Agriculture 2017, 97, 199–206. DOI: 10.1002/jsfa.7712.
- Huang, X.; Tu, Z.; Wang, H.; Zhang, Q. Probing the Conformational Changes of Ovalbumin after Glycation Using HDX-MS. Food Chemistry 2015, 166, 62–67. DOI: 10.1016/j.foodchem.2014.05.155.
- Tavano, O. L.;. Protein Hydrolysis Using Proteases: An Important Tool for Food Biotechnology. Journal of Molecular Catalysis B: Enzymatic 2013, 90, 1–11. DOI: 10.1016/j.molcatb.2013.01.011.
- Ghribi, A. M.; Gafsi, I. M.; Sila, A.; Blecker, C. Effects of Enzymatic Hydrolysis on Conformational and Functional Properties of Chickpea Protein Isolate. Food Chemistry 2015, 187, 322–330. DOI: 10.1016/j.foodchem.2015.04.109.
- Van Der Ven, C.; Gruppen, H.; De Bont, D. B.; Voragen, A. G. Correlations between Biochemical Characteristics and Foam-Forming and-Stabilizing Ability of Whey and Casein Hydrolysates. Journal of Agricultural and Food Chemistry 2002, 50, 2938–2946. DOI: 10.1021/jf011190f.
- Tamm, F.; Sauer, G.; Scampicchio, M.; Drusch, S. Pendant Drop Tensiometry for the Evaluation of the Foaming Properties of Milk-Derived Proteins. Food Hydrocolloids 2012, 27, 371–377. DOI: 10.1016/j.foodhyd.2011.10.013.
- Nimalaratne, C.; Bandara, N.; Wu, J. Purification and Characterization of Antioxidant Peptides from Enzymatically Hydrolyzed Chicken Egg White. Food Chemistry 2015, 188, 467–472. DOI: 10.1016/j.foodchem.2015.05.014.
- Abeyrathne, E.; Lee, H.; Jo, C.; Nam, K.; Ahn, D. Enzymatic Hydrolysis of Ovalbumin and the Functional Properties of the Hydrolysates. Poultry Science 2014, 93, 2678–2686. DOI: 10.3382/ps.2014-04155.
- Abeyrathne, E.; Lee, H.; Jo, C.; Suh, J.; Ahn, D. Enzymatic Hydrolysis of Ovomucin and the Functional and Structural Characteristics of Peptides in the Hydrolysates. Food Chemistry 2016, 192, 107–113. DOI: 10.1016/j.foodchem.2015.06.055.
- Huang, T.; Tu, Z.-C.; Xinchen-Shangguan,; Wang, H. Rheological and Structural Properties of Fish Scales Gelatin: Effects of Conventional and Ultrasound-Assisted Extraction. International Journal of Food Properties 2017, 20(S2), S1210–S1220 . DOI: 10.1080/10942912.2017.1295388.
- Lv, L.; Chi, Y.; Chen, C.; Xu, W. Structural and Functional Properties of Ovalbumin Glycated by Dry-Heating in the Presence of Maltodextrin. International Journal of Food Properties 2015, 18, 1326–1333. DOI: 10.1080/10942912.2011.620204.
- Huang, T.; Tu, Z.-C.; Wang, H.; Shangguan, X. Pectin and Enzyme Complex Modified Fish Scales Gelatin: Rheological Behavior, Gel Properties and Nanostructure. Carbohydrate Polymers 2017, 156, 294–302. DOI: 10.1016/j.carbpol.2016.09.040.
- Guo, Z.; Zhang, T.; Fang, K.; Liu, P. The Effect of Porosity and Stiffness of Glutaraldehyde Cross-Linked Egg White Scaffold Simulating Aged Extracellular Matrix on Distribution and Aggregation of Ovarian Cancer Cells. Colloids and Surfaces A: Physicochemical and Engineering Aspects 2016, 504, 43–52. DOI: 10.1016/j.colsurfa.2016.05.050.
- Guo, Z.; Zhang, T.; Chen, X.; Fang, K. The Effects of Porosity and Stiffness of Genipin Cross-Linked Egg White Simulating Aged Extracellular Matrix on Proliferation and Aggregation of Ovarian Cancer Cells. Colloids and Surfaces A: Physicochemical and Engineering Aspects 2017, 520, 649–660. DOI: 10.1016/j.colsurfa.2017.02.031.
- Huang, T.; Tu, Z.-C.; Shangguan, X.; Rheological Behavior, Emulsifying Properties and Structural Characterization of Phosphorylated Fish Gelatin. Food Chemistry 2018, 246:428–436. DOI: 10.1016/j.foodchem.2017.12.023.
- Tavernier, I.; Wijaya, W.; Van Der Meeren, P.; Dewettinck, K.; Patel, A. R. Food-Grade Particles for Emulsion Stabilization. Trends in Food Science & Technology 2016, 50, 159–174. DOI: 10.1016/j.tifs.2016.01.023.
- Lee, Y. Y.; Tang, T. K.; Phuah, E. T.; Alitheen, N. B. M. New Functionalities of Maillard Reaction Products as Emulsifier and Encapsulating Agent and Its Processing Parameters: A Brief Review. Journal of the Science of Food and Agriculture 2016, 30, 1379–1385.
- Pirestani, S.; Nasirpour, A.; Keramat, J.; Desobry, S.; Jasniewski, J. Effect of Glycosylation with Gum Arabic by Maillard Reaction in a Liquid System on the Emulsifying Properties of Canola Protein Isolate. Carbohydrate Polymers 2017, 157, 1620–1627. DOI: 10.1016/j.carbpol.2016.11.044.
- Liu, F.; Wang, D.; Xu, H.; Sun, C.; Gao, Y. Physicochemical Properties of β-carotene Emulsions Stabilized by Chlorogenic Acid–Lactoferrin–Glucose/Polydextrose Conjugates. Food Chemistry 2016, 196, 338–346. DOI: 10.1016/j.foodchem.2015.09.047.
- Hemar, Y.; Tamehana, M.; Munro, P.; Singh, H. Viscosity, Microstructure and Phase Behavior of Aqueous Mixtures of Commercial Milk Protein Products and Xanthan Gum. Food Hydrocolloids 2001, 15, 565–574. DOI: 10.1016/S0268-005X(01)00077-7.
- O’Charoen, S.; Hayakawa, S.; Ogawa, M. Food Properties of Egg White Protein Modified by Rare Ketohexoses through Maillard Reaction. International Journal of Food Science & Technology 2015, 50, 194–202. DOI: 10.1111/ijfs.2015.50.issue-1.
- Enomoto, H.; Nagae, S.; Hayashi, Y.; Li, C.-P. 79 Improvement of Functional Properties of Egg White Protein through Glycation and Phosphorylation by Dry-Heating. Asian-Australasian Journal of Animal Sciences 2009, 22, 591. DOI: 10.5713/ajas.2009.80363.
- Meinlschmidt, P.; Ueberham, E.; Lehmann, J.; Schweiggert-Weisz, U.; Eisner, P. Immunoreactivity, Sensory and Physicochemical Properties of Fermented Soy Protein Isolate. Food Chemistry 2016, 205, 229–238. DOI: 10.1016/j.foodchem.2016.03.016.
- Sun, Y.; Hayakawa, S.; Izumori, K. Modification of Ovalbumin with a Rare Ketohexose through the Maillard Reaction: Effect on Protein Structure and Gel Properties. Journal of Agricultural and Food Chemistry 2004, 52, 1293–1299. DOI: 10.1021/jf030428s.
- Kim, D.-Y.; Shin, W.-S. Functional Improvements in Bovine Serum Albumin–Fucoidan Conjugate through the Maillard Reaction. Food Chemistry 2016, 190, 974–981. DOI: 10.1016/j.foodchem.2015.06.046.
- Katekhong, W.; Charoenrein, S. Color and Gelling Properties of Dried Egg White: Effect of Drying Methods and Storage Conditions. International Journal of Food Properties 2017, 20, 1–12. DOI: 10.1080/10942912.2016.1233429.
- Hu, Y.; Liang, H.; Xu, W.; Wang, Y. Synergistic Effects of Small Amounts of Konjac Glucomannan on Functional Properties of Egg White Protein. Food Hydrocolloids 2016, 52, 213–220. DOI: 10.1016/j.foodhyd.2015.07.001.
- De Oliveira, F. C.; Coimbra, J. S. D. R.; De Oliveira, E. B.; Zuñiga, A. D. G.; Rojas, E. E. G. Food Protein-Polysaccharide Conjugates Obtained via the Maillard Reaction: A Review. Critical Reviews in Food Science and Nutrition 2016, 56, 1108–1125. DOI: 10.1080/10408398.2012.755669.
- Kong, N.; Guo, L.; Guan, D.; Liu, L. An Ultrasensitive ELISA for Medroxyprogesterone Residues in Fish Tissues Based on a Structure-Specific Hapten. Food Analytical Methods 2015, 8, 1382–1389. DOI: 10.1007/s12161-014-0023-4.
- Dong, A.; Huang, P.; Caughey, W. S. Protein Secondary Structures in Water from Second-Derivative Amide I Infrared Spectra. Biochemistry 1990, 29, 3303–3308. DOI: 10.1021/bi00465a022.
- Tu, Z.-C.; Huang, T.; Wang, H.; Sha, X.-M. Physico-Chemical Properties of Gelatin from Bighead Carp (Hypophthalmichthys Nobilis) Scales by Ultrasound-Assisted Extraction. Journal of Food Science and Technology 2015, 52, 2166–2174. DOI: 10.1007/s13197-013-1239-9.
- Liu, G.; Zhong, Q. Glycation of Whey Protein to Provide Steric Hindrance against Thermal Aggregation. Journal of Agricultural and Food Chemistry 2012, 60, 9754–9762. DOI: 10.1021/jf302883b.
- Bu, G.; Zhu, T.; Chen, F. The Structural Properties and Antigenicity of Soybean Glycinin by Glycation with Xylose. Journal of the Science of Food and Agriculture 2017, 97, 2256–2262. DOI: 10.1002/jsfa.8036.