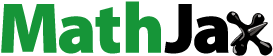
ABSTRACT
Ferulic acid, a well-known natural phenolic compound, is considerably reported for its hydroxyl and peroxyl radical scavenging activities. However, the antioxidant capacity of ferulic acid is limited by its relatively low solubility in hydrophobic media thereby preventing its application for autoxidation of fats and oils. To this end, several research efforts are being made to maximize the therapeutic benefits of ferulic acid and these efforts included but not limited to structural modification of ferulic acid to produce its derivatives. In this study, we synthesized ester and amide derivatives of ferulic acid and evaluated them for in vitro antioxidant potential as well as molecular docking properties. Data revealed that ferulic ester and amide derivatives had excellent antioxidant capacity and demonstrated strong inhibitory potential. Analysis of molecular docking indicated ferulic ester as potent inhibitor of target proteins in breast cancer as well as in oxidative stress. Taken together, the findings support potent antioxidant properties by these ferulic derivatives. Findings may become relevant where structural modification to enhance physico-chemical properties without compromising the antioxidant and/or medicinal potential are desirable.
Introduction
Ferulic acid (FA) is a phenolic compound with excellent antioxidant potential.[Citation1,Citation2] FA is one of the most abundant phenolic acids found in plants, and in many staple foods, such as fruits, vegetables, cereals, and coffee.[Citation3,Citation4] The antioxidant potential of FA has been explored for several therapeutic applications, and wide spectrum of beneficial activity for human health has been advocated for this phenolic compound, at least in part, because of its strong antioxidant activity.[Citation5,Citation6] It possesses antioxidant property by virtue of its phenolic hydroxyl group in its structure. The hydroxy and phenoxy groups of FA donate electrons to quench the free radicals. The presence of electron donating groups on the benzene ring (3-methoxy and more importantly, 4-hydroxyl) of FA gives the additional property of terminating free radical chain reactions. Another functional group in the FA, i.e., the carboxylic acid group with an adjacent unsaturated C-C double bond, can provide additional attack sites for free radicals, and thus, prevent them from attacking the membrane. In addition, this carboxylic acid group also acts as anchor of FA by which it binds to the lipid bilayer, providing some protection against lipid peroxidation.[Citation7] FA is considerably reported for its hydroxyl and peroxyl radicals scavenging activity in both brain cells and macrophages as a result of its ability to easily form resonance-stabilized phenoxy radical. Its antioxidant potential is however limited by its relatively low solubility in hydrophobic media which thus prevents its application for the autoxidation of fats and oils,[Citation8–Citation11] it is therefore necessary and stimulating to investigate the antioxidant potentials of synthesized FA derivatives which are most likely to be soluble in hydrophobic environment.
For example, many studies reported that amide derivatives of FA acid enhance insulin secretion, prevent oxidation of low density lipoprotein, as well as inhibit the cyclooxygenase COX-2-induced inflammatory diseases.[Citation12,Citation13] In addition, several other investigations have demonstrated better physico-chemical and antioxidant property by FA derivatives.[Citation14,Citation15] These studies revealed that FA derivatives possess diverse potentials, such as anticancer,[Citation16,Citation17] antiatherogenic,[Citation18] anticarcinogenic,[Citation19] and antibacterial agents,[Citation20] as well as anti-inflammatory activity.[Citation21,Citation22] Further, several FA derivatives have been shown to be more lipophilic, thus increasing the ability of the derivatives to cross lipid-rich cell membranes and thereby offer better antioxidant potential.[Citation23–Citation28]
In light of the aforementioned, we therefore studied structurally modified FA ester and amide derivatives and comparatively evaluated their in vitro antioxidant potentials as well as their possible protein binding interactions, using computational docking studies.
Materials and methods
General experimental
All chemical reagents used were of analytical grade and obtained from Sigma (St Louis, MO, USA) unless otherwise stated. IR spectra were recorded on Shimadzu (8400S) Fourier Transform-Infrared using KBr pellet. 1H-NMR (300 MHz) and 13C-NMR (75 MHz) spectra were recorded in CDCl3 or MeOD on Bruker 300 spectrometer. Absorbance measurements were taken on an UV/Vis (Jenway) Spectrophotometer. Chemical shifts are expressed in parts per million downfield from tetramethylsilane as an internal standard. Mass spectra were taken on JMS spectrometer (ESI+) ion trap LCQ, and thermoelectron corporation. Chromatography was carried out on a gravity column packed with silica gel as the stationary phase. Thin layer chromatography was performed on 0.2 mm pre-coated plates of silica gel 60F-264 (Merck), and spots were visualized using an ultraviolet-light lamp.
Synthesis of FA derivatives
FA syringaldehyde ester (compound 1)
FA (2.0 g, 10 mmol) and syringaldehyde (3.0 g, 16 mmol) with 2 drops of sulfuric acid were refluxed in 40 ml of DMSO for 3 h. The progress of the reaction was monitored by thin layer chromatography, and it was stopped when there was no sign of any further reaction taking place. The resulting mixture was chromatographed on silica gel (hexane-dichloromethane, 1:1) and this yielded 3.2 g of compound 1 (): (E)-4-formyl-2,6-dimethoxyphenyl 3-(4-hydroxy-3-methoxyphenyl)acrylate. Brown liquid (Yield: 87%). IR (KBr): vmax (cm−1) 3450 (OH), 3005 (Ar C-H), 2958–2858 (CH), 1693 (C = O), 1593 (C = C), 1236 (C-O). 1H NMR (300 MHz, CDCl3): δH 9.87 (s, 1H), 7.83 (d, 1H), 7.24 (s, 1H), 7.24 (s, 1H), 7.13 (s, 1H), 6.95 (s, 1H), 6.87 (d, 1H), 6.87 (s, 1H), 6.76 (d, 1H), 5.23 (ArOH), 3.92 (s,3H, OCH3), 3.89 (s, 6H, 2 OCH3). 13 C NMR (75 MHz, CDCl3): δC 191.1, 154.4, 146.8, 146.7, 145.9, 144.9, 140.1, 137.9, 137.8, 136.8, 126.4, 120.4, 120.2, 114.6, 114.1, 111.7, 56.3, 56.0, 55.9. ESI-MS; m/z: 376.86 (M + 18) + observed for C19H18O7.
Figure 1. Schematic for synthesis of ferulic acid (FA)—Syringaldehyde ester (Compound 1). FA (2.0 g, 10 mmol) and syringaldehyde (3.0 g, 16 mmol) with 2 drops of sulfuric acid were refluxed in 40 ml of DMSO for 3 h. The progress of the reaction was monitored by thin layer chromatography, and it was stopped when there was no sign of any further reaction taking place. The resulting mixture was chromatographed on silica gel (hexane-dichloromethane, 1:1) to afford 3.2 g of compound 1: (E)-4-formyl-2,6-dimethoxyphenyl 3-(4-hydroxy-3-methoxyphenyl)acrylate. Brown liquid (Yield: 87%). IR (KBr): vmax (cm−1) 3450 (OH), 3005 (Ar C-H), 2958–2858 (CH), 1693 (C = O), 1593 (C = C), 1236 (C-O). 1H NMR (300 MHz, CDCl3): δH 9.87 (s, 1H), 7.83 (d, 1H), 7.24 (s, 1H), 7.24 (s, 1H), 7.13 (s, 1H), 6.95 (s, 1H), 6.87 (d, 1H), 6.87 (s, 1H), 6.76 (d, 1H), 5.23 (ArOH), 3.92 (s,3H, OCH3), 3.89 (s, 6H, 2 OCH3). 13 C NMR (75 MHz, CDCl3): δC 191.1, 154.4, 146.8, 146.7, 145.9, 144.9, 140.1, 137.9, 137.8, 136.8, 126.4, 120.4, 120.2, 114.6, 114.1, 111.7, 56.3, 56.0, 55.9. ESI-MS; m/z: 376.86 (M + 18) + observed for C19H18O7.
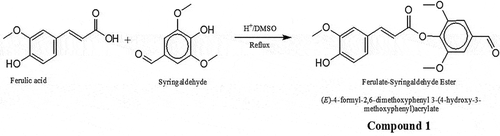
FA urethane amide (compound 2)
FA (5.0 g, 25 mmol) and urethane (10.0 g, 112 mmol) with catalytic amount of imidazole were irradiated for 4 min in a domestic microwave oven operating at 600 W and frequency 14545 Hz. The average optimum time of reaction was initially studied to obtain the most appropriate time required for the complete reaction. Hence, reaction was left to progress for 5 min and product checked on the thin layer chromatography thereafter. The resulting mixture was chromatographed on silica gel and eluted with n-hexane yielding 3.5 g of compound 2 (): (E)-ethyl 3-(4-hydroxy-3-methoxyphenyl)acryloylcarbamate. Brown liquid (Yield: 51%). IR (KBr): vmax (cm−1) 3508–3300 (OH, NH), 3086 (Ar C-H), 2958–2858 (CH), 1815 (C = O), 1606 (C = C), 1238 (C-O). 1H NMR (300 MHz, MeOD): δH 7.8 (s, 1H, NH), 7.00 (dd, 2H), 6.67 (d, 1H, JHH 8.1, Hortho), 6.65 (d, 1H, JHH 8.1, Hortho), 6.66 (s, 1H, Ar-OH), 4.82 (s, 1H, Ar-OH), 3.84 (s, 3H, OCH3), 3.30 (m, 2H), 1.51 (t, 3H). 13 C NMR (75 MHz, MeOD): δC 148.9, 148.3, 147.5, 137.9, 129.3, 120.8, 116.1, 116.0, 111.3, 110.2, 56.4, 56.3, 19.46. ESI-MS; m/z: 283.08 (M + 18) + observed for C13H15NO5.
Figure 2. Schematic for synthesis of FA—Urethane Amide (Compound 2). FA (5.0 g, 25 mmol) and urethane (10.0 g, 112 mmol) with catalytic amount of imidazole were irradiated for 4 min in a domestic microwave oven operating at 600 W and frequency 14545 Hz. The average optimum time of reaction was initially studied to obtain the most appropriate time required for the complete reaction. Hence, reaction was left to progress for 5 min and product checked on the thin layer chromatography thereafter. The resulting mixture was chromatographed on silica gel and eluted with n-hexane to afford 3.5 g of compound 2: (E)-ethyl 3-(4-hydroxy-3-methoxyphenyl)acryloylcarbamate. Brown liquid (Yield: 51%). IR (KBr): vmax (cm−1) 3508–3300 (OH, NH), 3086 (Ar C-H), 2958–2858 (CH), 1815 (C = O), 1606 (C = C), 1238 (C-O). 1H NMR (300 MHz, MeOD): δH 7.8 (s, 1H, NH), 7.00 (dd, 2H), 6.67 (d, 1H, JHH 8.1, Hortho), 6.65 (d, 1H, JHH 8.1, Hortho), 6.66 (s, 1H, Ar-OH), 4.82 (s, 1H, Ar-OH), 3.84 (s, 3H, OCH3), 3.30 (m, 2H), 1.51 (t, 3H). 13 C NMR (75 MHz, MeOD): δC 148.9, 148.3, 147.5, 137.9, 129.3, 120.8, 116.1, 116.0, 111.3, 110.2, 56.4, 56.3, 19.46. ESI-MS; m/z: 283.08 (M + 18) + observed for C13H15NO5.
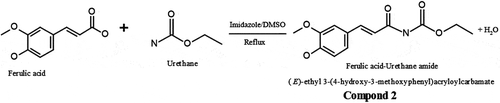
In vitro antioxidant screening
Superoxide anion scavenging assay
This was performed according to the method described by Luo et al.[Citation27] Briefly, 1 ml of FA or its derivatives was added to 4.5 ml of Tris-HCl buffer. After 20 min-incubation at 25°C, pyrogallol (25 mmol) was added and the reaction terminated after 4 min with HCl solution (8 mol/L). The mixture was centrifuged at 1000 g (Uniscope Laboratory Centrifuge SM800B, Surgifriend Medicals, UK) for 15 min, and the absorbance reading was recorded at 325 nm (Jenway UV/Vis spectrophotometer, Staffordshire, UK). The percentage of scavenging activity was estimated as follows:
where Ao: absorbance without sample; As: absorbance with sample.
Hydroxyl radical scavenging assay
This assay was performed according to the method described by Luo et al.[Citation27] Briefly, 1 ml of FA or its derivatives was incubated with 2 mM EDTA-Fe (0.5 ml), 3% H2O2 (1 ml) in 4.5 ml sodium phosphate buffer (150 mM, pH 7.4) for 30 min. Hydroxyl radical was detected by recording the absorbance at 520 nm (Jenway UV/Vis spectrophotometer, Staffordshire, UK). Scavenging activity was estimated as follows:
where Ao: absorbance of control; As: absorbance of sample.
Ferric reducing antioxidant power assay
This assay was performed according to the method described by Benzie and Strain.[Citation29] Briefly, an aliquot of FA or its derivatives was added to a mixture of sodium phosphate buffer (200 mM) containing 1% potassium ferricyanide. After 20 min-incubation at 50°C, 10% TCA was added and the mixture was centrifuged at 650 g for 10 min (Uniscope Laboratory Centrifuge SM800B, Surgifriend Medicals, UK). The supernatant was collected, mixed with equal volume of ddH2O and added to 0.1% ferric chloride. The absorbance of mixture was recorded at 700 nm (Jenway UV/Vis spectrophotometer, Staffordshire, UK) against blank (ddH2O). L-ascorbic acid was used as standard reference. A higher absorbance indicates a higher reducing power.
Scavenging assay for 2,2, diphenyl-1-picrazyl hydrazyl radical
This assay was performed according to the method described by Devi et al.[Citation30] Briefly, an aliquot of FA or its derivatives was added to DPPH-methanol (0.1 mM) solution. After 30 min-incubation at 25°C in the dark, decrease in absorbance was recorded at 517 nm (Jenway UV/Vis spectrophotometer, Staffordshire, UK). Methanol plus DPPH without FA was used as the positive control, while the blank contained only methanol without DPPH. Percentage activity was estimated as:
Nitrogen radical species scavenging assay
This assay was performed according to the method described by Ilavarasan et al.[Citation31] Briefly, equal volumes of the FA or its derivatives and sodium nitroprusside were mixed. After 5 h-incubation at 25°C, the mixture was diluted with Greiss reagent (1:1 v/v). The absorbance of mixture was recorded at 546 nm (Jenway UV/Vis spectrophotometer, Staffordshire, UK). Activity was estimated as:
Deoxyribose degradation inhibitory potential (specific and non-specific)
This assay was performed according to the method described by Lee et al.[Citation32] For non-specific activity, aliquot of FA or its derivatives was added to a reaction buffer (100 µM FeC2l3, 104 µM EDTA, 1.5 mM H2O2, 2.5 mM deoxyribose and 100 µM ascorbic acid, pH 7.4). After 1 h-incubation at 37°C, 0.5% TBA in 0.025 M NaOH and 2.8% TCA were added to the mixture and heated for 30 min at 80°C. The mixture was cooled on ice and absorbance recorded at 532 nm (Jenway UV/Vis spectrophotometer, Staffordshire, UK).
For site-specific activity (which represents ability of FA or its derivatives to directly chelate iron ions and interfere with hydroxyl generation), the same procedure was repeated but without the EDTA. Activity was estimated as:
where Abs: Absorbance.
Total antioxidant capacity
The assay was performed according to Saha et al.[Citation33] Briefly, an aliquot of FA or its derivatives was added to a reagent solution (0.6 M sulfuric acid, 28 mM sodium phosphate, and 4 mM ammonium molybdate). After 90 min-incubation at 95°C, the mixture was cooled to room temperature and the absorbance recorded at 695 nm (Jenway UV/Vis spectrophotometer, Staffordshire, UK) against the blank. Methanol was used as the blank. The activity was expressed as the number of gram equivalents of ascorbic acid.
Membrane stabilizing effect
The assay was performed as described by Malomo et al.[Citation34] The assay mixture consisted of PBS, indomethacin (reference drug) or different concentrations of FA or its derivatives, and 2% erythrocyte suspension in isosaline. The control was prepared without the reference drug (or FA/derivatives) whereas the drug control and the FA control lacked erythrocyte suspension. After 30 min-incubation at 56°C, the mixture was cooled and centrifuged at 1000× g (Uniscope Laboratory Centrifuge SM800B, Surgifriend Medicals, UK) for 15 min. The absorbance of the supernatant was recorded at 560 nm (Jenway UV/Vis spectrophotometer, Staffordshire, UK). The activity was estimated as:
Metal-chelating activity
The assay was performed according to Devi et al.[Citation30] Briefly, aliquot of FA or its derivatives was added to FeCl2. The reaction was initiated by adding ferrozine followed by methanol with vigorous shaking. After 10 min-incubation at room temperature, the absorbance of the mixture was recorded at 562 nm (Jenway UV/Vis spectrophotometer, Staffordshire, UK). The activity was estimated as:
where As: absorbance of sample; Ac: absorbance of control.
Preparation of liver homogenate
Apparently, healthy adult Wistar rats were sourced from the small Animal House, Department of Biological Sciences, Landmark University, Omu Aran, Kwara State. Liver samples were excised into ice-cold isotonic solution (0.15 M KCl), weighed, and homogenized. The homogenate was centrifuged at 5000 × g for 10 min using a refrigerated centrifuge (Anke TDL-5000B, Shanghai, China). The supernatant was collected and used for further biochemical analyses.
Inhibition of lipid peroxidation in rat liver homogenate
Assay for in vitro lipid peroxidation of liver homogenate was carried out according to the procedure described by Okhawa et al.[Citation35] Briefly, aliquots of liver homogenate in KCl (10% w/v) were taken in different test tubes and the test compounds (FA/FA derivatives or ascorbic acid; concentration range 1–10 mg/ml) and 15 mM FeSO4 were added. After 30 min-incubation at 37°C on a shaker, aliquot of the mixture was added to TBA in 50% acetic acid and then heated in a water bath at 85°C for 30 min. The reaction was stopped by adding equal volumes of TCA. The mixture was centrifuged at 1500 × g (Anke TDL-5000B, Shanghai, China) for 10 min, and the absorbance was recorded at 535 nm (UV/VIS spectrophotometer, Jenway, Staffordshire, UK). The inhibiting activity was estimated as: % Inhibition = [(Control – Test) × 100]/Control
Computational docking studies
Dataset
The X-ray crystal structures of the molecular targets along with their respective ligands were downloaded from the Protein Data Bank. The dataset was structurally processed using Discovery Studio, software version 4.1.[Citation36] In this study, we have used two proteins which included; [1] Vanin-1: a key enzyme which links metabolic disease and inflammation (PDB ID: 4CY) along with its original ligand (2R)-2,4-dihydroxy-N-[(3S)-3-hydroxy-4-phenylbutyl]-3, 3-dimethylbutanamide. [2] estrogen receptor in complex with its original ligand 2-phenyl-1-[4-(2-piperidin-1-YL-ethoxy)-phenyl]-1,2,3,4-tetrahydro-isoquinolin-6-ol (PDB ID: 1UOM).
Computational docking protocol
In order to predict the possible molecular mechanism involved, molecular docking was conducted using the three-dimensional X-ray crystals pre-processed and curated (e.g., removal of water, addition of explicit hydrogen) using the Protein Preparation Wizard from the Gold Suite.[Citation37] The histidine protonation states were determined and fixed in the protein structures. Binding site was determined using the prior knowledge of the original ligand interaction site for the respective protein structures. GoldScore was used as the scoring function to rank docked compounds. However, scores were not considered for final pose selections. In the docking simulation process, each ligand was kept flexible but the amino acid residues of the proteins were held rigid. The selection of atoms in the active site within 6 A or original ligand was chosen. The number of best generated pose was set to 10.
Data analysis
Data were analyzed using the one-way ANOVA (GraphPad Software Inc., San Diego, CA) and presented as the mean ± SEM. Difference among the group means was determined by the Tukey’s test. Mean values at p < 0.05 were considered to be significant.
Results
Synthesis of (E)-4-formyl-2,6-dimethoxyphenyl 3-(4-hydroxy-3-methoxyphenyl)acrylate[Citation1]
The synthesis of 1 was carried out in the presence of DMSO and sulfuric acid using a classical method of refluxing to afford 87% yield of the brown liquid product. The reaction product was purified via gravity column chromatography on silica gel using hexane-dichloromethane at 1:1. The reaction which gave an indication of the formation of the product was monitored by the TLC and was terminated as soon as no further reaction was observed. The FTIR indicated the presence of all necessary peaks while the disappearance of the carboxylic proton signal of the FA confirms a successful reaction. The molecular weight of the water adduct of the product was confirmed at m/z 376.
Synthesis of (E)-ethyl 3-(4-hydroxy-3-methoxyphenyl)acryloylcarbamate[Citation2]
The synthesis of 2 was attained via microwave assisted approach to afford 51% of the product. The condensation reaction was accomplished using imidazole as catalyst and the product purified using column chromatography packed with silica gel and eluted with n-hexane. The product indicated the presence of N-H stretching vibration at 3508 to confirm a successful condensation. The NMR showed all corresponding signals while the mass spectrum also showed the molecular ion peak as water adduct at m/z 283.
In vitro antioxidant assays
The FA ester and amide derivatives showed strong protective potential against the hydroxyl-induced deoxyribose degradation both in the site specific and non-site specific assays. The average protective activity of the FA derivatives is > 60% and compares favorably with those of FA and ascorbic acid (–). The FA ester and amide derivatives showed appreciable iron-chelating potential similar to those of the reference compounds, FA, and ascorbic acid (). The FA ester showed zero scavenging activity against superoxide radicals at the doses tested. In contrast, FA amide showed scavenging activity similar to those of FA and ascorbic acid (). The FA amide showed no scavenging activity for hydroxyl radical while the FA ester had scavenging potential higher than those of the FA and ascorbic acid ().
Figure 3. Inhibitory potential of ferulic ester and amide derivatives against degradation of deoxyribose: (A) non-specific inhibition; (B) specific inhibition. Data are presented as mean ± standard error of mean (SEM), n = 3.
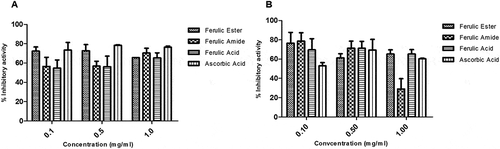
Figure 4. Activity of ferulic ester and amide derivatives. (A) Metal chelating. (B) Superoxide anion scavenging. (C) Hydroxyl radical scavenging. Data are presented as mean ± SEM, n = 3.
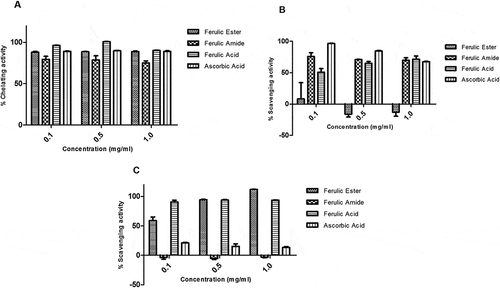
The FA ester and amide derivatives appreciably scavenged the reactive nitrogen radicals with capacity similar to those of the FA and ascorbic acids (). The FA ester and amide derivatives showed appreciable DPPH radical scavenging potential which appeared concentration dependent. The scavenging activity of the FA derivatives compare favorably with those of the FA and ascorbic acid (). The FA ester derivative showed superior ferric reducing antioxidant capacity relative to the FA, ascorbic acid, and/or FA amide derivative ().
Figure 5. Protective activity of ferulic ester and amide derivatives against (A) peroxynitrite radical; (B) DPPH radical; (C) FRAP. Data are presented as mean ± SEM, n = 3.
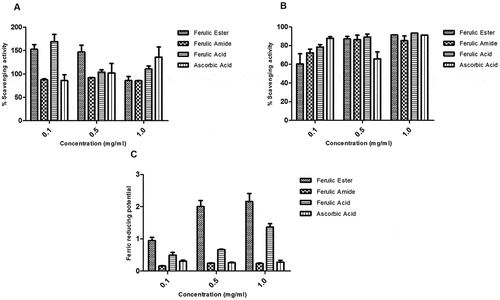
The FA ester and amide derivatives had higher total antioxidant capacity (expressed as equivalent of ascorbic acid) relative to FA (). The FA ester and amide derivatives showed strong membrane-protective effect which increased with rising concentration. Similarly, FA and ascorbic acid demonstrated membrane-protective potential (). The FA ester and amide derivatives demonstrated appreciable protection against iron-induced lipid peroxidation of liver homogenate. The protective activity of the FA derivatives compares well with those of the FA and ascorbic acid ().
Computational docking analysis
Molecular docking poses were analyzed based on the best orientation and interaction of the ligand with respect to the molecular receptor as well as docking scores. However, preferences were given on the binding interactions over the docking scores. A hierarchical clustering was computed using root-mean-square deviation between coordinates of all poses of each compound. The largest cluster at a clustering distance of 3 Å was investigated further. With the chosen settings, it was possible to re-dock the original ligands into a similar orientation of their respective molecular receptors. Analysis of molecular docking indicated ferulic ester as potent inhibitor of target proteins in breast cancer as well as in oxidative stress (–).
Figure 7. Computational prediction of binding mode: (A) original ligand (pink); (B) FA ester derivative (green) docked in the crystal structure of the breast cancer protein (cyan).
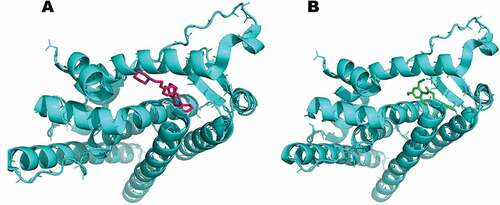
Figure 8. Interaction between receptor side chains and docked ligand. (A) Binding mode interactions of the original ligand with the receptor (Pdb Code: 1UOM), the ligand binds to the receptor residues GLU353, ARG 394 of chain A. (B) Binding mode interactions of the FA ester derivative the receptor (Pdb Code: 1UOM). The ligand binds to the receptor residues ARG 394, HIS524 of chain A. The ligand-interaction plots were generated using Discovery Studio (Acceryls Software, Inc).
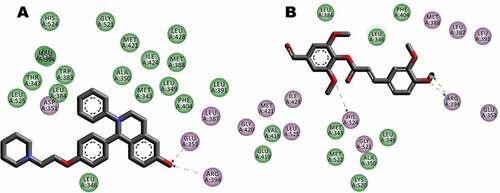
Figure 9. Computational prediction of binding mode: (A) original ligand shown in (cyan); (B) FA ester derivative shown (green) docked in the crystal structure of the oxidative stress protein (pink).
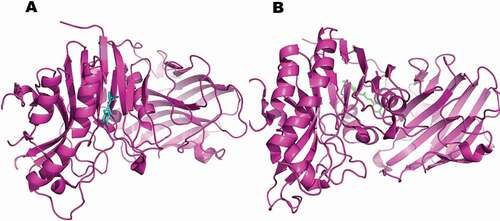
Figure 10. Interaction between receptor side chains and docked ligand. (A) Binding mode interactions of the original ligand with the receptor (Pdb Code: 4CYG), the ligand binds to the receptor residues CYS211, LYS178, GLU390 of chain A. (B) Binding mode interactions of the FA ester derivative the receptor (Pdb Code: 4CYG). The ligand binds to the receptor residues CYS211, LYS178, PHE212, and LYS272 of chain A. The ligand-interaction plots were generated using Discovery Studio (Acceryls Software, Inc).
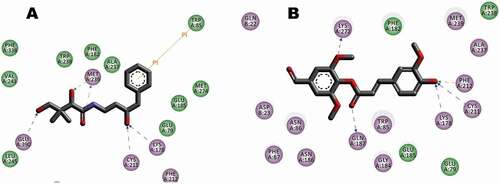
Discussion
Research efforts to maximize the therapeutic benefits of FA have led to the synthesis of several derivatives with enhanced physico-chemical and antioxidant property.[Citation14,Citation15] For example, the esterification of FA has been employed to increase its effectiveness.[Citation23] In the present study, we provide comparative evaluation of the antioxidant activities of FA and its amide and ester derivatives.
The FA ester and amide derivatives as well as the FA showed significant levels of protection against deoxyribose site specific and non-site specific degradation. This may be due to the phenolic groups present in their structures. The findings are consistent with a previous study which showed that FA and its derivatives protected against deoxyribose degradation.[Citation6] The protection against deoxyribose degradation afforded by FA as well as the FA ester and amide derivatives may suggest potential to protect the DNA against reactive oxygen species-induced damage. Also, the FA as well as the FA ester and amide derivatives demonstrated potential to chelate iron. Though the iron-chelating capacity of FA as demonstrated in the present study does not conform with a previous study,[Citation38] FA was reported to lack Fe2+ and Cu2+ chelating activity. The reason for the contrasting result is not known but may not be unconnected with the assay procedure. Nevertheless, the iron- or metal-chelating potential of the FA and FA ester and amide derivatives could serve to boost their antioxidant capacity where appropriate. For example, in the present study, the metal-chelating potential may be linked to higher total antioxidant capacity by the FA ester and amide derivatives. Meanwhile, the capacity of FA to scavenge nitrogen reactive species is consistent with a previous report by Ogiwara et al.[Citation39] which investigated the antioxidant potential of FA.
Furthermore, the FA ester and amide derivatives showed appreciable DPPH radical scavenging potential which was concentration dependent. A previous study[Citation25] showed that FA was more effective than its derivatives in scavenging DPPH radicals. However, in the present study, the DPPH scavenging activity does not appear to be higher than those of FA ester and amide derivatives. This is consistent with findings by Naik et al.[Citation40] The FA ester derivative showed superior ferric reducing antioxidant capacity relative to the FA, ascorbic acid, and/or FA amide derivative. The FA ester and amide derivatives showed good membrane-protective effect which appear to be dose-dependent. The membrane-stabilizing effect may indicate potential for anti-inflammatory potential. Moreover, the ability of a compound to inhibit lipid peroxidation may be an indicator of antioxidant potential. FA ester and amide derivatives strongly inhibited ferrous-induced lipid peroxidation, thus implying that FA derivatives have potent antioxidant properties similar to those of the parent compound FA. This is consistent with previous findings.[Citation5] Furthermore, the capacity of the FA ester and amide derivatives to inhibit lipid peroxidation underscores its membrane-protecting activity. Taken together, our findings are consistent with investigations which have linked lipophilic character of FA derivatives to higher antioxidant property.[Citation25,Citation26] For example, the addition of ethyl groups makes FA more lipophilic, increasing the ability of the derivative to cross lipid-rich cell membranes. The increased lipophilicity may underscore a better antioxidant potential as the membranes such as the blood–brain barrier become more permeable and accessible. Furthermore, the molecular docking analysis indicated ferulic ester as potent inhibitor of target proteins in breast cancer as well as in oxidative stress, thus providing additional evidence in support of the medicinal prospects of the FA derivatives.
Conclusion
Data obtained in this study indicated that strategic modification of FA did not significantly affect its antioxidant activity, thus implying that FA ester and amide derivatives may possess much better antioxidant activities compared with the parent compound. The findings of this study may find relevance in cases where structural modification of FA for physico-chemical purposes as well as the preservation of the antioxidant potential is expedient.
Declaration of interest
The authors declare that there is no conflict of interest.
Acknowledgment
The authors thank the laboratory staff at the Department of Biological Sciences, Landmark University, Nigeria for technical support.
References
- Yogeeta, S.; Hanamuntra, R.; Gnanapragasam, A.; Subramanian, S.; Rajakannu, S.; Devaki, T. Attenuation of Abnormalities in the Lipid Metabolism during Experimental Myocardial Infarction Induced by Isoproterenol in Rats: Beneficial Effects of Ferulic Acid and Ascorbic Acid. Basic and Clinical Pharmacology and Toxicology. 2006, 98(5), 467–472. DOI: 10.1111/j.1742-7843.2006.pto_335.x.
- Rice-Evans, C.; Miller, N.; Paganga, G. Antioxidant Properties of Phenolic Compounds. Trends Plant Science. 1997, 2(4), 152–159. DOI: 10.1016/S1360-1385(97)01018-2.
- Cui, C.; Wang, Z.; Du, X.; Wang, L.; Yu, S.; Liu, X.; Li, Z.; Zhao, W. Synthesis and Antiviral Activity of Hydrogenated Ferulic Acid Derivatives. Journal of Chemistry. 2013, 2013, 1–5, Article ID 269434. DOI: 10.1155/2013/269434.
- Paiva, L.; Goldbeck, R.; Santos, W.; Squina, F. Ferulic Acid and Derivatives: Molecules with Potential Application in the Pharmaceutical Field. Braz. Journal of Pharmaceutical Sciences. 2013, 49(3), 395–411. DOI: 10.1590/S1984-82502013000300002.
- Sultana, R.;. Ferulic Acid Ethyl Ester as a Potential Therapy for Neurodegenerative Disorders. Biochimica et Biophysica Acta. 2012, 1822, 748–752. DOI: 10.1016/j.bbadis.2011.10.015.
- Srinivasan, M.; Sudheer, A. R.; Menon, V. P. Ferulic Acid: Therapeutic Potential through Its Antioxidant Property. Journal of Clinical Biochemitry and Nutritoin. 2007, 40(2), 92–100. DOI: 10.3164/jcbn.40.92.
- Kanasaki, J.; Aksenova, M.; Stoyanova, A.; Butterfield, D. A. Ferulic Acid Antioxidant Protection against Hydroxyl and Peroxy Radical Oxidation in Synaptosomal and Neuronal Cell Culture Systems in Vitro: Structure Activity Studies. Journal of Nutritional Biochemistry. 2002, 13, 273–281. DOI: 10.1016/S0955-2863(01)00215-7.
- Hosoda, A.; Ozaki, Y.; Kashiwada, A.; Mutoh, M.; Wakabayashi, K.; Mizuno, K.; Nomura, E.; Taniguchi, H. Syntheses of Ferulic Acid Derivatives and Their Suppressive Effects on Cyclooxygenase-2 Promoter Activity. Bioorganic and Medicinal Chemistry. 2002, 10(4), 1189–1196. DOI: 10.1016/S0968-0896(01)00386-8.
- Anselmi, C.; Bernardi, F.; Centini, M.; Gaggelli, E.; Gaggelli, N.; Valensin, D.; Valensin, G. Interaction of Ferulic Acid Derivatives with Human Erythrocytes Monitored by Pulse Field Gradient NMR Diffusion and NMR Relaxation Studies. Chemistry and Physics of Lipids. 2005, 134(2), 109–117. DOI: 10.1016/j.chemphyslip.2004.12.005.
- Rakotondramanana, D.L.A.; Delomenèd, M.; Baltas, M.; Duran, H.; Bedos-Belval, F.; Rasoanaivo, P.; Negre-Salvayre, A.; Gornitzka, H. Synthesis of Ferulic Ester Dimers, Functionalisation and Biological Evaluation as Potential Antiatherogenic and Antiplasmodial Agents. Bioorganic and Medicinal Chemistry. 2007, 15(18), 6018–6026. DOI: 10.1016/j.bmc.2007.06.047.
- Chigorimbo-Murefu, N. T. L.; Riva, S.; Burton, S. G. Lipase-Catalysed Synthesis of Esters of Ferulic Acid with Natural Compounds and Evaluation of Their Antioxidant Properties. Journal of Molecular Catalalysis B: Enzymatic. 2009, 56(4), 277–282. DOI: 10.1016/j.molcatb.2008.05.017.
- Kalgutkar, A. S.; Marrnet, A. B.; Crews, B. C. Ester and Amide Derivatives of the Nonsteroidal Antiinflammatory Drug, Indomethacin, as Selective Cyclooxygenase-2 Inhibitors. Journal of Medicinal Chemistry. 2000, 43(15), 2860–2870. DOI: 10.1021/jm000004e.
- Wang, F.; Lu, W.; Zhang, T.; Dong, J.; Gao, H.; Li, P.; Wang, S.; Zhang, J. Development of Novel Ferulic Acid Derivatives as Potent Histone Deacetylase Inhibitors. Bioorganic and Medicinal Chemistry. 2013, 21(22), 6973–6980. DOI: 10.1016/j.bmc.2013.09.021.
- Fang, X.; Shima, M.; Kadota, M.; Tsuno, T.; Adachi, S. Suppressive Effect of Alkyl Ferulate on the Oxidation of Linoleic Acid. Bioscience Biotechnology and Biochemistry. 2006, 70, 457–461. DOI: 10.1271/bbb.70.457.
- Stamatis, H.; Sereti, V.; Kolisis, F. N. Enzymatic Systematic Systhesis of Hydrophilic and Hydrophobic Derivatives of Natural Phenolic Acids in Organic Media. Journal of Molecular Catalalysis B: Enzymatic. 2001, 11, 323–328. DOI: 10.1016/S1381-1177(00)00016-3.
- Li, W.; Li, N.; Tang, Y.; Li, B.; Lu, L.; Zhang, X.; Fu, H.; Duan, J. Biological Activity Evaluation and Structure-Activity Relationships Analysis of Ferulic Acid and Caffeic Acid Derivatives for Anticancer. Bioorganic and Medicinal Chemistry. Letters. 2012, 22, 6085–6088. DOI: 10.1016/j.bmcl.2012.08.038.
- Balakrishnan, S.; Menon, V. P.; Manoharan, S. Ferulic Acid Inhibits 7,12-dimethylbenz[α]anthracene-induced Hamster Buccal Pouch Carcinogenesis. Journal of Medicinal Food. 2008, 11(4), 693–700. DOI: 10.1089/jmf.2007.0103.
- Kwon, E. Y.; Do, G. M.; Cho, Y. Y.; Park, Y. B.; Jeon, S. M.; Choi, M. S. Anti-Atherogenic Property of Ferulic Acid in Apolipoprotein E-Deficient Mice Fed Western Diet: Comparison with Clofibrate. Food and Chemical Toxicology. 2010, 48(8–9), 2298–2303. DOI: 10.1016/j.fct.2010.05.063.
- Lin, X. F.; Min, W.; Luo, D. Anticarcinogenic Effect of Ferulic Acid on ultraviolet-B Irradiated Human Keratinocyte HaCaT Cells. Journal of Medicinal Plant Research. 2010, 4(16), 1686–1694.
- Shanmugam, H.; Doble, M. Combination of Ferulic Acid and Antibiotics as Effective Antibacterial Agents. Planta Medica. 2010, 76(12), 1191. DOI: 10.1055/s-0030-1264292.
- Ou, L.; Kong, L. Y.; Zhang, X. M.; Niwa, M. Oxidation of Ferulic Acid by Momordica Charantia Peroxidase and Related Anti-Inflammation Activity Changes. Biological and Pharmaceutical Bulletin. 2003, 26(11), 1511–1516. DOI: 10.1248/bpb.26.1511.
- Tetsuka, T.; Baier, L. D.; Morrison, A. R. Antioxidants Inhibit Interleukin-1-Induced Cyclooxygenase and Nitric-Oxide Synthase Expression in Rat Mesangial Cells: Evidence for Post-Transcriptional Regulation. Journal of Biological Chemistry. 1996, 271(20), 11689–11693. DOI: 10.1074/jbc.271.20.11689.
- Nazare, A.; Faria, C.; Chiari, B.; Petronio, M.; Regasini, L.; Silva, D.; Correa, M.; Isaac, V.; Fonseca, L.; Ximenes, V. Ethyl Ferulate, a Component with anti-Inflammatory Properties for Emulsion-Based Creams. Molecules. 2014, 19, 8124–8139. DOI: 10.3390/molecules19068124.
- Kumar, A.; Kanwar, S. Synthesis of Ethyl Ferulate in Organic Medium Using Celite-Immobilized Lipase. Bioresource Technology. 2011, 102(3), 2162–2167. DOI: 10.1016/j.biortech.2010.10.027.
- Karamac, M.; Bucinski, A.; Pegg, R.; Amarowicz, R. Antioxidant and Antiradical Activity of Ferulates. Czech Journal of Food Sciences. 2005, 23(2), 64–68. DOI: 10.17221/3373-CJFS.
- Sorensen, A.; Lyneborg, K.; Villeneuve, P.; Jacobsen, C. Alkyl Chain Length Impacts the Anti-Oxidative Effect of Lipophilized Ferulic Acid in Fish Oil Enriched Milk. Journal of Functional Foods. 2014, 18, 959–967. DOI: 10.1016/j.jff.2014.04.008.
- Luo, X. D.; Basile, M. J.; Kennelly, E. J. Polyphenolic Antioxidants from the Fruits of Chrysophyllum Cainito L. [Star Apple]. Journal Of Agriculture and Food Chemistry. 2002, 50(6), 1379–1382. DOI: 10.1021/jf011178n.
- Meeprom, A.; Sompong, W.; Chan, C.; Adisakwattana, S. Isoferulic Acid, a New Anti-Glycation Agent, Inhibits Fructose- and Glucose-Mediated Protein Glycation in Vitro. Molecules. 2013, 18, 6439–6454. DOI: 10.3390/molecules18066439.
- Benzie, F. F.; Strain, J. J. Ferric Reducing/Antioxidant Power Assay: Direct Measure of Total Antioxidant Activity of Biological Fluids and Modified Version for Simultaneous Measurement of Total Antioxidant Power and Ascorbic Acid Concentration. Methods in Enzymology. 1999, 299, 15–23.
- Devi, G. K.; Manivannan, K.; Thirumaran, G.; Rajathi, F. A. A.; Anantharaman, P. In Vitro Antioxidant Activities of Selected Seaweeds from Southeast Coast of India. Asian Pacific Journal of Tropical Medicine. 2011, 4, 205–211. DOI: 10.1016/S1995-7645(11)60070-9.
- Ilavarasan, R.; Mallika, M.; Venkataraman, S. Antiinflammatory and Antioxidant Activities of Cassia fistula Linn. Bark Extracts. African Journal of Traditional Complementary and Alternative Medicines. 2005, 2(1), 70–85.
- Lee, M. J.; Maliakal, P.; Chen, L.; Meng, X.; Bondoc, F. Y.; Prabhu, S.; Lambert, G.; Mohr, S.; Yang, C. S. Pharmacokinetics of Tea and [+] -Epigallocatechin-3-Gallate by Humans: Formation of Different Metabolites and Individual Variability. Cancer Epidermiology Biomarker Prevention. 2002, 11, 1025–1032.
- Saha, M. R.; Hasan, S. M. R.; Akter, R.; Hossain, M. M.; Alam, M. S.; Alam, M. A.; Mazumder, M. E. H. In Vitro Free Radical Scavenging Activity of Methanol Extract of the Leaves of Mimusops Elengi Linn. Banglandesh Journal of Veterinary Medicine. 2008, 6, 197–202.
- Malomo, S. O.; Ore, A.; Yakubu, M. T. In Vitro and in Vivo Antioxidant Activity of the Aqueous Extract of Celosia argentea Leaves. Indian Journal of Pharmacology. 2011, 43(3), 278–285. DOI: 10.4103/0253-7613.81519.
- Ohkawa, H.; Ohishi, N.; Yagi, K. Assay for Lipid Peroxides in Animal Tissues by Thiobarbituric Acid Reaction. Analytical Biochemistry. 1979, 95, 351–358. DOI: 10.1016/0003-2697(79)90738-3.
- Accelrys Software Inc. Discovery Studio Modeling Environment, Release 3.5; Accelrys Software Inc, San Diego, 2012.
- Jones, G.; Willett, P.; Glen, R. C.; Leach, R.; Taylor, R. Development and Validation of a Genetic Algorithm for Flexible Docking. Journal of Molecular Biology. 1997, 267, 727–748. DOI: 10.1006/jmbi.1996.0897.
- Psotová, J.; Lasovský, J.; Vičar, J. Metal-Chelating Properties, Electrochemical Behavior, Scavenging and Cytoprotective Activities of Six Natural Phenolics. Biomedical Papers. 2003, 147(2), 147–153. DOI: 10.5507/bp.2003.020.
- Ogiwara, T.; Satoh, K.; Kadoma, Y.; Murakami, Y.; Unten, S.; Atsumi, T.; Sakagami, H.; Fujisawa, S. Radical Scavenging Activity and Cytotoxicity of Ferulic Acid. Anticancer Research. 2002, 22(5), 2711–2717.
- Naik, G. H.; Priyadarsini, K. I.; Satav, J. G.; Banavalikar, M. M.; Sohoni, D. P.; Biyani, M. K.; Mohan, H. Comparative Antioxidant Activity of Individual Herbal Components Used in Ayurvedic Medicine. Phytochemistry. 2003, 63, 97–104. DOI: 10.1016/S0031-9422(02)00754-9.