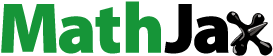
ABSTRACT
Fish oil is an excellent source of omega-3 fatty acids and is easily susceptible to oxidation. Microencapsulation is a commonly employed technique to protect fish oil against oxidation. In the present study, the potential of chitosan in combination with bovine gelatin and maltodextrin as wall material for microencapsulation of fish oil by spray drying was evaluated. To improve the oxidative stability of the fish oil microencapsulates, oregano (Origanum vulgare L) extract was added at 0.50 g/100 g of emulsion. The spray-dried powder showed a moisture content of 2.8 – 3.2 g/100 g of spray-dried powder. The powder contained spherical microparticles with different sizes as indicated by scanning electron microscope images. Encapsulation efficiency of microencapsulates ranged between 68.94% and 81.88%. Differential scanning calorimetry and Fourier-transformed infrared spectroscopy analysis of microencapsulates revealed the possible structural stabilization of core and wall material. The oxidative stability of fish oil microencapsulates were monitored under three different temperature (60°C, 28 ± 2°C, and 4°C). Incorporation of oregano extract minimized the generation of secondary and tertiary oxidation products as indicated by lower peroxide value and thiobarbituric acid reactive substance values compared to control. Overall, the results suggested that combination of chitosan along with bovine gelatin and maltodextrin as wall material improved the surface morphology of the microparticle and encapsulation efficiency, whereas incorporation of oregano extract in fish oil before spray drying enhanced the oxidative stability during storage.
Introduction
Fish oil represents a functional food ingredient, which contains important components for maintenance of good health and prevention of a range of human diseases via its beneficial effects on the heart, brain, and nervous system.[Citation1] Autoxidation of polyunsaturated fatty acids (PUFAs) in fish oil leads to the development of oxidation products and limits the shelf-life of the foods.[Citation2] It can be prevented by the addition of antioxidants, use of controlled storage conditions, and microencapsulation. Spray drying is one of the most commonly used techniques to protect fish oil against oxidation.[Citation3] Spray drying offers many advantages over conventional drying methods such as freeze drying, extrusion, complex-coacervation, etc. However, only limited numbers of wall materials (such as maltodextrin or saccharose, milk or soy proteins, gelatin, and gum Arabic) are compatible with this technology.[Citation4] Hence, there is a need to search new wall materials that can be used at high temperature and high evaporation conditions, which prevail in the spray-drying environment. Chitosan is one of the most abundant naturally occurring polysaccharides and structurally it is a β (1,4)-linked copolymer of D-glucosamine and N-acetyl-D-glucosamine. Several studies have highlighted application of chitosan as an antioxidant, antimicrobials, edible films, emulsion stabilization, and texture modification.[Citation5–Citation7] Despite several properties that make it attractive as an encapsulating material. Very few studies have been conducted using chitosan as a wall material for encapsulation. To increase the efficiency of microencapsulation, a combination of different wall materials are commonly used.[Citation8] Although encapsulation itself prevents lipid oxidation, additional stabilization with antioxidants is required to ensure maximum protection during processing and subsequent storage of microencapsulated bioactive ingredients. The essential oil derived from plant or spices are rich in polyphenols and they act as an effective antioxidant for encapsulating fish oil. It has been reported that oregano essential oil extracted from Origanum vulgare L. has been shown to possess antioxidant, antibacterial, antifungal, and analgesic activities.[Citation9–Citation11]These activities are mainly due to the presence of two major phenolic compounds such as carvacrol and thymol.[Citation12] Based on the above information, the present study was aimed to improve the structural and oxidative stability of fish oil microencapsulates using chitosan––bovine gelatin––maltodextrin with/without addition of oregano extract and to study the physiochemical changes of microencapsulated fish oil under different temperature condition.
Materials and methods
Raw materials
Fish oil (Seacod, Universal Medicare, Mumbai, India) was used for the preparation of emulsion and encapsulates. Fatty-acid composition of fish oil as determined by gas chromatography–mass spectroscopic (GC–MS) analysis indicated the following major fatty acids: C14:0–3.89%, C16:0–5.08%, C16:1–5.42%, C18:0–3.70%, C18:1(n7)–2.92%, C18:1(n9)–12.47%, C18:2–5.19%, C18:3–7.50%, C18:4–2.18%, C20:1–11.29%, C20:4–1.87%, C22:5–3.32%, C20:5–12.23%, and C22:6–11.12%. Chitosan was prepared from shrimp shell waste in the laboratory by the method of Nair and Madhavan.[Citation13]The degree of deacetylation was found to be 90%. Bovine gelatin and maltodextrin procured from Himedia. Oregano extract containing 45% (w/w) essential oil was purchased from Synthite Industries Ltd. (Cochin, India). The chemical composition of oregano extract as determined by GC–MS analysis indicated the following major components: carvacrol (13.8%), thymol (11.6%), β-fenchyl alcohol (13.2%), and δ-terpineol (6.5%), γ-terpinene (10.5%) and α-terpinene (3.7%), 1-methyl-3-(1-methylethyl)-benzene (6.8%), and monoterpene hydrocarbons (25.2%).
Preparation of emulsion and spray drying
Fish oil and wall material were used at the ratio of 1:4. The composition of the emulsion was selected based on our previously reported results.[Citation14,Citation15] Initially, chitosan was dissolved into the 1% acetic-acid solution followed by other wall materials such as bovine gelatin and maltodextrin. After the complete dissolution of the wall material, the fish oil–oregano extract blends/fish oil were added to wall material slowly while stirring at 1000 rpm for 10 min using magnetic stirrer. Then, it was homogenized with a tissue homogenizer (Poly system PT 2100, Kinematica, AG) at 25000 rpm for 5 min. Three different emulsion formulations were made as follows: (i) fish oil + maltodextrin+ bovine gelatin (MGO), (ii) fish oil + chitosan + bovine gelatin + maltodextrin (CGM), and (iii) fish oil-oregano extract + chitosan+ bovine gelatin + maltodextrin (CGME). All the samples were kept at room temperature for stabilization of the emulsion for 1 h and then spray dried using a pilot-scale spray dryer (Hemraj Pvt. Ltd, Mumbai, India) equipped with a two-fluid nozzle atomizer under the following experimental conditions: inlet temperature 180°C; outlet temperature 90°C; spray flow feed nozzle diameter 1.5 mm; feed rate was maintained at 15–20 g/min; and air pressure 2.5 bar. The production yield of microencapsulates by spray drying was 62.5 ± 1.5%. Fish oil encapsulates prepared by spray drying were kept in air tight condition and stored at three different temperature: (i) accelerated temperature (60°C), (ii) room temperature (28 ± 2°C), and (iii) refrigerated temperature (4°C) for further analysis.
Characterization of microencapsulates
Particle morphology
Surface morphology of microencapsulates was evaluated by scanning electron microscope (SEM) (JEOL-JSM-7600F, Japan). Samples were uniformly spread onto double-sided adhesive carbon tape mounted on SEM stubs, coated with platinum using auto fine coater (JEOL-JFC-1600, Japan) in a vacuum and examined by SEM at 10 Kv with the magnification of 2000×.
Moisture content
The moisture contents of fish oil encapsulates were determined by the method of AOAC.[Citation16]
Hygroscopicity
Hygroscopicity of microencapsulates was determined by the method of Cai and Corke[Citation17] with slight modification. Approximately 0.5 g of each sample was taken in a preweighed petri-dish and was kept in a desiccator filled with saturated NaCl solution (75% RH) at 25°C. After a week, samples were weighed and hygroscopicity of the samples was determined.
Flow properties
The flow properties of microspheres were determined using bulk density (ρB) and tapped densities (ρT) measurements by the method of Chinta et al.[Citation18] The cohesiveness of microencapsulates was evaluated in terms of Hausner ratio (HR). HR was calculated as described by Turchiuli et al.[Citation19]
Encapsulation efficiency
Encapsulation efficiency (EE) was derived from the relationship between total oil and surface oil or free oil. Total oil was extracted from microcapsules by Soxhlet method.[Citation15] Surface oil was extracted according to Sankarikutty et al.[Citation20]. Encapsulation efficiency ratio was calculated as follows:
Color analysis
The color of microencapsulated fish oil was measured using a Hunter-Lab Scan XE––Spectrocolorimeter (Hunter Associates Laboratory, Reston, USA) at D-65 illuminant and 10° observer. Results were expressed by CIE (Commission Internationale de L Eclairage’s) color values [L*(lightness), a*(redness), and b*(yellowness)]. The instrument was calibrated using white and black standard ceramic tiles and the readings were recorded in the inbuilt software.
Differential scanning calorimetry analysis
About 3–5 mg of microencapsulates were placed into differential scanning calorimetry (DSC) aluminum pans and equilibrated over saturated salt solutions in desiccators at 25°C until equilibrium were reached. The samples were then hermetically sealed with lids for analysis and weighed. The DSC analysis was performed in a TA-MDSC-2920(Ta Instruments, New Castle, De, USA) in an inert atmosphere (45 mL/min of N2). Instrument calibration was carried out with indium (T melting = 156.6°C). Thermo-analytical curves were obtained by heating/cooling rate of the sample at 10°C/min at the temperature ramp of 20°C180°C under nitrogen atmospheric condition.
Fourier-transformed infrared spectroscopy analysis
The Fourier-transformed infrared spectroscopy (FTIR) spectra of encapsulates were recorded in a Shimadzu IR-Prestige-21 using diffuse reflectance assembly in the spectral range of 4500–400 cm−1. About 1 mg of microencapsulates were mixed with potassium bromide (100 mg) in the ratio of 1:100 (w/w) and the reflectance spectra were recorded up to 64 scans with a resolution of 4 cm−1. All the reflectance spectra were transformed to transmission spectra using the Kubelka–Munk algorithm.
Determination of oxidative stability
Microencapsulated fish oil was packed in amber color bottle and stored at three different temperature: (i) accelerated temperature (60°C), (ii) room temperature (28 ± 2°C), and (iii) refrigerated temperature (4°C). Oxidative stability of microencapsulated fish oil stored under accelerated temperature of 60ºC was tested up to 7 days at 24-h interval. Microencapsulates kept at room and the refrigerated temperature was analyzed up to 4 weeks at 1-week interval. Lipid peroxides of microencapsulates were determined according to the method described by Shantha and Decker[Citation21] and expressed in millieq.O2/kg. Thiobarbituric acid reactive substance (
TBARS) value was determined according to the method described by McDonald and Hultin.[Citation22] TBARS was expressed in mg of malonaldehyde (MDA)/kg.
Statistical analysis
One-way analysis of variance (ANOVA) using Statistical Package for Social Science (SPSS) software version 16.0 (SPSS Inc, Chicago, IL) were carried out to evaluate the difference in the mean value (microencapsulates properties, color, PV, and TBARS). All mean separations were carried out by Tukey multiple range test using the significance level of 95% (P < 0.05). Statistical significance is indicated by appropriate letters within the figures and table.
Results and discussion
Moisture content and flowability of microencapsulated fish oil
The moisture content of the fish oil encapsulates ranged between 2.8 and 3.2%. The obtained results are in good agreement with those obtained previously (2.89–3.02%) for tuna oil powders.[Citation23]There was a significant difference (p < 0.05) in moisture content between the samples. Moisture content was high in MGO followed by CGME and CGM. It might be due to the composition of emulsion used in the study. The flow properties are one of the important property in processing operations such as formulation, mixing, packaging, and storage.[Citation24] Quality parameters like bulk density, tapped density, and HR have been used to assess powder flowability.[Citation25] In the present study, the bulk density of the microencapsulates varied from 0.23 to 0.34 g/mL (). Moreover, CGM and CGME had lower bulk density. Muzaffar and Pradyuman kumar[Citation26] observed lower bulk density for spray-dried powder at higher inlet temperature (180°C). In the present study, similar inlet temperature (180°C) was followed which might be resulted lower bulk density of sample. Another possible reason for a decrease in bulk density is the increase in viscosity of the feed material.[Citation27] Accordingly, fish oil emulsion of CGM and CGME had viscous nature than MGO. It might be due to combined mixture of chitosan and bovine gelatin. In the present study, fish oil encapsulates had an HR between 1.21 and 1.24. Based on the HR, the powder flowability is categorized as follows: 1–1.1, excellent; 1.12–1.18, good; 1.19–1.25, fair; 1.26–1.34, passable; 1.35–1.46, poor; 1.46–1.59, very poor; and >1.60, awful.[Citation18] Accordingly, in the present study, CGM and CGME were followed fair flowability. However, MGO had higher HR (1.33) which resulted in passable flowability. Results indicates that CGM and CGME have a better encapsulation of fish oil than MGO as indicated by powder flow properties and higher EE.
Table 1. Physical properties of microencapsulated fish oil.
Encapsulation efficiency
Determination of free and encapsulated oil will provide the information of EE. EE is used to assess the quality of the dried microcapsules because it has an effect on oxidation sensitivity and flow powder properties. It also reflects the percentage of protection of oil droplets embedded within the wall material. In the present study, MGO had lower EE of 68.94%. However, CGM and CGME had an EE of 76.55%, 81.88%, respectively. In the present study, CGME sample had higher EE than CGM and MGO. Results confirm the structural stabilization of the wall material (chitosan–gelatin–maltodextrin) with polyphenols. Binsi et al.[Citation15] observed similar results for fish oil microencapsulates produced with sage polyphenols. Vishnu et al.[Citation28] reported that mixture of vanillic acid grafted chitosan and protein could improve EE of fish oil microencapsulates.
Hygroscopicity
In general, spray-dried products are easily absorb moisture from the surrounding air. Among the three microencapsulates, CGM and CGME had less hygroscopicity (3.45–4.96%) than MGO (6.86%). Results indicated that chitosan provided a good film forming properties along with maltodextrin and bovine gelatin which reduced moisture absorption. Moreover, CGM and CGME had higher EE than MGO. Higher hygroscopicity (6.86%) of MGO might be due to its higher moisture content. It has been reported that an increase in particle size leading to a reduction of total powder surface through which the water may be absorbed.[Citation29]
Surface morphology of microencapsulates
Surface morphology of MGO sample showed spherical shape with shrinkage ()). It may be due to the composition of emulsion and also it could due to the lower encapsulated oil of MGO resulted in extensive shrinkage during the early stage of the drying process and vacuole formation during later drying period.[Citation30] Microencapsulates prepared by spray drying had a maximum size of 15 µm, while the smallest size of 1.82 µm. Jeyakumari et al.[Citation14] and Kolanowski et al.[Citation8] observed spherical structure of microencapsulates with different sizes by spray drying of oil. Surface morphology of both CGM and CGME fish oil encapsulates showed individual spherical shape (). It indicates stable structural interactions between chitosan—bovine gelatin—maltodextrin polymers. This is also confirmed from the higher EE of both the samples. Microparticle contained chitosan had smoother surface morphology than those produced without chitosan. The present results are consistent with previous studies reported for fish oil microencapsulates produced by spray drying.[Citation31–Citation33]
Thermal behavior of microencapsulates
DSC analysis will give the information on the physico-chemical changes of microcapsules during heating. The DSC analysis of MGO showed an endothermic peak at 76.12°C due to melting followed by decomposition ()). CGM showed two exothermic peaks at 52.84°C and 96.07°C followed by it showed melting peak at the temperature of 167.05°C ()). Similarly, CGME showed two exothermic peaks at 66.84°C and 96.23°C followed by it showed melting peak at the temperature of 167.15°C ()). It indicated that both CGM and CGME showed exothermic event with crystallization phase initially and melting with decomposition at later stages. The spray-drying process results in the formation of an amorphous or disordered crystalline phase due to the rapid drying of the droplets.[Citation34] These endothermic events are characteristic of dehydration, whereas exothermic effects can be associated with crystallizations, oxidations, or some decomposition reactions.[Citation34] Results indicated that lower thermal stability of MGO than CGM and CGME. There was no characteristic peak of wall material in the thermogram of fish oil microencapsulates. Results indicated that fish oil and wall material is completely dispersed in the microparticles. These results are in good agreement with that of previous studies related to encapsulation of fat-soluble compounds.[Citation28,Citation35]
FTIR analysis
FTIR spectroscopic analysis of encapsulate was carried out to understand the nature of the interaction between the wall material and fish oil. The stretching region of the hydroxyl group, –OH was shown in the band range of 3600–3200 cm−1. The band at 3397–3405 cm−1 indicates the presence of hydroxyl group in the microencapsulates. The C–H peak appeared at 2923 cm−1 to 2926 cm−1 in the microencapsulates also observed in the pure fish oil at 2923 cm−1. The amide I (mainly C = O stretch) and II (C–N stretching coupled with N–H bending modes) peaks for MGO,CGM, and CGME appeared at the similar frequency of 1658 cm−1, 1656 cm−1, and 1651 cm−1, respectively (). The slight decrease in the intensity of the amide I band in the CGME spectra suggests a major conformational change by the presence of polyphenols. Furthermore, a more pronounced ester group was detectable at 1745 cm−1 in CGME than CGM, which might have resulted from the interaction of terminal carboxyl group of bovine gelatin/chitosan/maltodextrin with phenolic components[Citation36] A major difference in the spectra observed at 2358–2360 cm−1 for CGM and MGO indicated the characteristics of the maltodextrin and bovine gelatin. However, it was found to absent in CGME. It might be occurred due to the merging of polymer with a phenolic group. A minor band shift from 3334 cm–1 in MGO, 3397 cm–1 in CGM toward a higher wavenumber of 3405 cm_1 was observed in CGME, suggesting a possible interaction between the wall polymers and oregano polyphenols.[Citation37] The characteristic absorption of the chitosan is the band at 1559.17 cm−1, which is assigned to the stretching vibration of an amino group of chitosan and 1333.5 cm−1 assigned to vibration of C–H. Another band at 3367 cm−1 is due to amine N–H symmetric vibration. The peak of 2927.41 cm−1 is typical C–H vibration. The peaks around 896.73 cm−1 and 1154.19 cm−1 indicates the saccharide structure of chitosan and the C–O stretching vibration found at 1080.91 cm−1.[Citation38,Citation39] Furthermore, clearly explaining, the carbonyl area split into three clear peaks at 1745 cm–1, 1651 cm–1, 1547 cm–1, which could be assigned to the C–O stretching vibration, and to the NH3 and COO– groups characteristics of the interpolyelectrolyte complex.[Citation40] Other peaks observed were at 1153 cm–1, 1027 cm–1 due to, C–O stretching of carboxylic acid and C–N stretching of amines, respectively.
Physicochemical changes of microencapsulated fish oil during storage
Changes in color value
Oxidation of fish lipids results in the formation of brown-color which leads to color changes in the product. Tristimulus color values of L*(lightness/whiteness), a*(redness), and b*(yellowness) are used as indices for the color changes in the food product during storage. Initial L* for MGO, CGM, and CGME was found to be 94.07, 88.95, and 79.65, respectively. The differences in the L* value may be due to the composition of emulsion used in the present study. L* value showed decreased trend during accelerated storage condition ()). A similar trend also observed in microencapsulates stored at room and refrigerated temperature (). However, a lower rate of L* value was observed in microencapsulates stored under refrigerated condition. It may be due to the presence of oregano extract in the sample. Results showed that there is a significant difference (p < 0.05) in L* value with respect to storage days and between the sample. All the encapsulates showed a positive b* and a* value with increasing trend during storage which indicated that the progress of lipid oxidation in the microencapsulates. The present results are in good agreement with the previous reports for microencapsulates contained ginger essential oil/sage polyphenols.[Citation14,Citation15] Klaypradit and Hugan[Citation23] reported that reaction of chitosan with protein and sugar molecules might lead to a higher yellowness formation in the microencapsulated fish oil.
Changes in peroxide value
Fish lipids are susceptible to oxidation due to its unsaturation nature. Determination of peroxide value (PV) in food products indicates the amount of formation of hydro peroxides, i.e., primary lipid oxidation products. In the present study, PV values showed increasing trend during storage (). Initial PV of microencapsulates was 3.5 millieq.O2/kg and it increased to 16.5 millieq.O2/kg, 8.8 millieq.O2/kg, 7.2 millieq.O2/kg in MGO sample stored under accelerated, room and refrigerated condition, respectively. Incase of CGM, it was increased to14.5 millieq.O2/kg, 7.5 millieq.O2/kg, 5.2 millieq.O2/kg, respectively. However, in CGME, the level of lipid hydroperoxide formation was lower. Results showed that there is a significant difference (p < 0.05) in PV between the samples with respect to storage temperature and days. It may be due to the antioxidant activities of phenolics compounds present in the oregano extracts. Results are in good agreement with previous reports for microencapsulates contained plant extracts and essential oil.[Citation13,Citation41] It was observed that the rate of peroxidation was less in fish oil microencapsulates stored at room and refrigerated temperature which was comparable with standard specification value of 10 millieq.O2/kg value is accepted in oils and fats.[Citation42] However, sample kept under accelerated temperature crossed the limit of acceptability on the 4th day and 5th day for MGO and CGM, respectively. The present results are in good agreement with an earlier report on the protection of microencapsulated fish oil with sage extract under accelerated condition.[Citation15]
Changes in thiobarbituric acid reactive substances
TBARS assay is a useful tool in monitoring lipid peroxidation owing to its sensitivity and simplicity.[Citation43] Measuring secondary oxidation products is important in the determination of lipid oxidation in food products for human consumption because they are generally odor-active, whereas primary oxidation products are colorless and flavorless. In the present study, TBARS values showed increasing trend during storage (). The higher rate of oxidation was observed for the sample stored under accelerated condition. TBARS value of 1–2 mg MDA/kg of fat is regarded as the limit for acceptability of fish and fishery products.[Citation44] Results showed that MGO, CGM, and CGME reached the acceptable limit of TBARS values on 24 h, 48 h, and 96 h, respectively. Results showed that there is a significant difference (p < 0.05) in TBARS value between the samples with respect to storage temperature and time. It was observed that the levels of TBARS values were lower (0.55–1.5 mg MDA/kg) in the CGME which was stored under refrigerated condition than room temperature (3.2 mg MDA/kg) and accelerated condition (7.5 mg MDA/kg). Results suggested that incorporation of oregano extract in fish oil before spray drying could extend the protection of lipid oxidation. The present results are in good agreement with previous reports for fish oil microencapsulates stabilized with ginger essential oil and phenols.[Citation14,Citation15]
Conclusion
Fish oil its natural state has a taste and smells that makes it less attractive to consumers. Microencapsulation of fish oil helps in protecting the unsaturated fatty acids against oxidation and increases their shelf-life. However, the selection of wall material is most important because it determines the success of the microencapsulation process. In the present study, potential of chitosan as a wall material for improving structural and oxidative stability of fish oil microencapsulates was evaluated by incorporating polyphenol-rich oregano extract as an additional antioxidant. SEM analysis indicated that addition of chitosan along with bovine gelatin and maltodextrin improved the surface morphology of the microparticle and EE. DSC and FTIR analysis of microencapsulates revealed the possible structural stabilization of core and wall material. Oxidative stability studies indicated that incorporation of oregano extract in fish oil during emulsion preparation could reduce the lipid oxidation in microcapsules during spray-drying process and storage. Moreover, microencapsulated fish oil prepared in the study can be used as functional food ingredients for developing oxidative stable omega-3 fatty-acid-enriched food products.
Acknowledgments
The authors wish to thank Dr C.N. Ravishankar, Director, CIFT, Cochin for his encouragement and for providing required facilities to carry out this study.
References
- Wu, K.; Chai, X.; Chen, Y. Microencapsulation of Fish Oil by Simple Coacervation of Hydroxypropyl Methylcellulose. Chinese Journal of Chemistry 2005, 23, 1569–1572. DOI: 10.1002/cjoc.200591569.
- Lee, S. W.; Kim, M. H.; Kim, C. K. Encapsulation of Ethanol by Spray Drying Technique: Effects of Sodium Lauryl Sulfate. International Journal of Pharmaceutics 1999, 187, 193–198. DOI: 10.1016/S0378-5173(99)00185-4.
- Bhandari, B. R.; Patel, K. C. Spray Drying of Food Materials - Process and Product Characteristics. Drying Technologies. In Food Processing; Chen, X. D., Mujumdar, A. S. eds.; Blackwell Publishing Ltd: Oxford, 2008; pp 113–159.
- Desai, K. G. H.; Park, H. J. Recent Developments in Microencapsulation of Food Ingredients. Drying Technology 2005, 23, 1361–1394. DOI: 10.1081/DRT-200063478.
- Reesha, K. V.; Panda, S. K.; Bindu, J.; Varhese, T. O. Development and Characterization of an LDPE/chitosan Composite Antimicrobial Film for Chilled Fish Storage. International Journal of Biological Macromolecules 2015, 79, 934–942. DOI: 10.1016/j.ijbiomac.2015.06.016.
- Jeyakumari, A.; Ninan, G.; Joshy, C. G.; Parvathy, U.; Zynudheen, A. A.; Lalitha, K. V. Effect of Chitosan on the Shelf Life of Restructured Fish Products from Pangasius (Pangasianodon Hypophthalmus) Surimi during Chilled Storage. Journal of Food Science and Technology 2016, 53(4), 2099–2107. DOI: 10.1007/s13197-016-2174-3.
- Remya, S.; Mohan, C. O.; Bindu, J.; Sivaraman, G. K.; Venkateshwarlu, G.; Ravishankar, C. N. Effect of Chitosan Based Active Packaging Film on the Keeping Quality of Chilled Stored Barracuda Fish. Journal of Food Science and Technology 2016, 53(1), 685–693. DOI: 10.1007/s13197-015-2018-6.
- Kolanowski, W.; Laufenberg, G.; Kunz, B. Fish Oil Stabilization by Microencapsulation with Modified Cellulose. International Journal of Food Science and Nutrition 2004, 55, 333–343. DOI: 10.1080/09637480410001725157.
- Olmedo, R. H.; Nepote, V.; Grosso, N. R. Preservation of Sensory and Chemical Properties in Flavoured Cheese Prepared with Cream Cheese Base Using Oregano and Rosemary Essential Oils. LWT – Food Science and Technology 2013, 53, 409–417. DOI: 10.1016/j.lwt.2013.04.007.
- Dambolena, J. S.; Zunino, M. P.; Lucini, E. I.; Olmedo, R.; Banchio, E.; Bima, P. J.; Zygadlo, J. A. Total Phenolic Content, Radical Scavenging Properties and Essential Oil Composition of Origanumspecies from Different Populations. Journal of Agricultural and Food Chemistry 2010, 58, 1115–1120. DOI: 10.1021/jf903203n.
- De Falco, E.; Mancini, E.; Roscigno, G.; Mignola, E.; Taglialatela-Scafati, O.; Senatore, F. Chemical Composition and Biological Activity of Essential Oils of OriganumvulgareL. Subsp. Vulgare L. Under Different Growth Conditions. Molecules 2013, 18, 14948–14960. DOI: 10.3390/molecules181214948.
- Baydar, H.; Sagdic, O.; Ozkan, G.; Karadogan, T. Antibacterial Activity and Composition of Essential Oils from Oreganum, Thymbra and Satureja Species with Commercial Importance in Turkey. Food Control 2004, 15, 169–172. DOI: 10.1016/S0956-7135(03)00028-8.
- Nair, K. G. R.; Madhavan, P. Utilization of Prawn waste-Isolation of Chitin and Its Conversion to Chitosan. Fisheries Technology 1974, 11(1), 60.
- Jeyakumari, A.; Kothari, D. C.; Venkateshwarlu, G. Oxidative Stability of Microencapsulated Fish Oil during Refrigerated Storage. Journal of Food Processing and Preservation 2015, DOI: 10.1111/jfpp.12433.
- Binsi, P. K.; Nayak, N.; Sarkar, P. C.; Jeyakumari, A.; Muhamed, A.; Ninan, G.; Ravi Shankar, C. N. Structural and Oxidative Stabilization of Spray Dried Fish Oil Microencapsulates with Gum Arabic and Sage Polyphenols: Characterization and Release Kinetics. Food Chemistry 2017, 219, 158–168. DOI: 10.1016/j.foodchem.2016.09.126.
- AOAC. Official Methods of Analysis, 17th ed.; Association of Analytical Chemists: Washington, DC, 2000.
- Cai, Y. Z.; Corke, H. Production and Properties of Spray-Dried Amaranthus Betacyanin Pigments. Journal of Food Science 2000, 65(7), 1248–1252. DOI: 10.1111/jfds.2000.65.issue-7.
- Chinta, D. D.; Graves, R. A.; Pamujula, S.; Praetorius, N.; Bostanian, L. A.; Mandal, T. K. Spray-Dried Chitosan as a Direct Compression Tableting Excipient. Drug Development and Industrial Pharmacy 2009, 35, 43–48. DOI: 10.1080/03639040802149053.
- Turchiuli, C.; Fuchs, M.; Bohin, M.; Cuvelier, M. E.; Ordonnaud, C.; Peyrat- Maillard, M. N.; Dumoulina, E. Oil Encapsulation by Spray Drying and Fluidized Bed Agglomeration. Innovative Food Science & Emerging Technologies 2005, 6(1), 29–35. DOI: 10.1016/j.ifset.2004.11.005.
- Sankarikutty, B.; Sreekumar, M. M.; Narayann, C. S.; Mathew, A. G. Studies on Microencapsulation of Cardamom Oil by Spray Drying Technique. Journal of Food Science and Technology 1988, 25, 352–356.
- Shantha, N. C.; Decker, E. A. Rapid, Sensitive, Iron-Based Spectrophotometric Methods for Determination of Peroxide Values of Food Lipids. Journal of AOAC International 1994, 77(2), 421–424.
- Mcdonald, R. E.; Hultin, H. O. Some Characteristics of the Enzymic Lipid Peroxidation System in the Microsomal Fraction of Flounder Skeletal Muscle. Journal of Food Science 1987, 52, 15–21. DOI: 10.1111/jfds.1987.52.issue-1.
- Klaypradit, W.; Huang, Y. W. Fish Oil Encapsulation with Chitosan Using Ultrasonic Atomizer. LWT – Food Science and Technology 2008, 41, 1133–1139. DOI: 10.1016/j.lwt.2007.06.014.
- Kim, E. H. J.; Chen, X. D.; Pearce, D. Effect of Surface Composition on the Flow Ability of Industrial Spray-Dried Dairy Powders. Colloids and Surfaces 2005, 46, 182–187. DOI: 10.1016/j.colsurfb.2005.11.005.
- Fitzpatrick, J. J.; Ahrne, L. Food Powder Handling and Processing: Industry Problems, Knowledge Barriers and Research Opportunities. Chemical Engineering and Processing 2005, 44, 209–214. DOI: 10.1016/j.cep.2004.03.014.
- Muzaffar, K.; Pradyuman, K. Spray Drying of Tamarind Pulp: Effect of Process Parameters Using Protein as Carrier Agent. Journal of Food Processing and Preservation 2016, DOI: 10.1111/jfpp.12781.
- Comunian, T. A.; Monterrery-Quintero, E. S.; Thomazini, M.; Balieiro, J. C. C.; Piccone, P.; Pittia, P.; Favaro- Trindade, C. S. Assessment of Production Efficiency, Physicochemical Properties and of Stability of Spray Dried Chlorophyllide, a Natural Food Colourant, Using Gum Arabic, Maltodextrin and Soy Protein Isolate –Based Carrier System. International Journal of Food Science and Technology 2011, 46, 1259–1265. DOI: 10.1111/j.1365-2621.2011.02617.x.
- Vishnu, K. V.; Chatterjee, N. S.; Ajeeshkumar, K. K.; Lekshmi, R. G. K.; Tejpal, C. S.; Mathew, S.; Ravishankar, C. N. Microencapsulation of Sardine Oil: Application of Vanillic Acid Grafted Chitosan as a Bio-Functional Wall Material. Carbohydrate Polymers 2007, DOI: 10.1016/j.carbpol.2017.06.076.
- Samborska, K.; Bienkowska, B. Physicochemical Properties of Spray Dried Honey Preparations, Zesz. Problemowe Postepów Nauk Rolniczych 2013, 575, 91–105.
- Hogan, S. A.; McNamee, B. F.; O’Riordan, E. D.; O’Sullivan, M. Emulsification and Microencapsulation Properties of Sodium Caseinate/Carbohydrate Blends. International Dairy Journal 2011, 11, 137–144. DOI: 10.1016/S0958-6946(01)00091-7.
- Rocha, G. A.; Favaro-Trindade, C. S.; Grosso, C. R. F. Microencapsulation of Lycopene by Spray Drying: Characterization, Stability and Application of Microencapsules. Food and Bioproducts Processing 2012, 90, 37–42. DOI: 10.1016/j.fbp.2011.01.001.
- Gallado, G.; Guida, I.; Martinez, V.; Lopez, M. C.; Bernhardt, D.; Blasco, R.; Hermida, L. G. Microencapsulation of Linseed Oil by Spray Drying for Functional Food Application. Food Research International 2013, 52, 473–482. DOI: 10.1016/j.foodres.2013.01.020.
- Kagami, Y.; Sugimura, S.; Fujishima, N.; Matsuda, K.; Kometani, T.; Matsumura, Y. Oxidative Stability, Structure, and Physical Characteristics of Microcapsules Formed by Spray Drying of Fish Oil with Protein and Dextrin Wall Materials. Journal of Food Science 2003, 68, 2248–2255. DOI: 10.1111/jfds.2003.68.issue-7.
- Corrigan, O. I.;. Thermal Analysis of Spray Dried Products. Thermochimca Acta 1995, 248, 245–258. DOI: 10.1016/0040-6031(94)01891-J.
- Yang, X.; Gao, N.; Hu, L.; Li, J.; Sun, Y. Development and Evaluation of Novel Microcapsules Containing Poppy-Seed Oil Using Complex Coacervation. Journal of Food Engineering 2015, 161, 87–93. DOI: 10.1016/j.jfoodeng.2015.03.027.
- Saravanan, M. K.; Rao, P. Pectin–Gelatin and Alginate–Gelatin Complex Coacervation for Controlled Drug Delivery: Influence of Anionic Polysaccharides and Drugs Being Encapsulated on Physicochemical Properties of Microcapsules. Carbohydrate Polymers 2010, 80(3), 808–816. DOI: 10.1016/j.carbpol.2009.12.036.
- Hasni, I.; Bourassa, P.; Hamdani, S.; Samson, G.; Carpentier, R.; Tajmir-Riahi, H. A. Interaction of Milk A-And B-Caseins with Tea Polyphenols. Food Chemistry 2011, 126(2), 630–639. DOI: 10.1016/j.foodchem.2010.11.087.
- de Souza Costa-Junior, E.; Pereira, M. M.; Mansur, H. S. Properties and Biocompatibility of Chitosan Films Modified by Blending with PVA and Chemically Crosslinked. Journal of Materials Science Materials in Medicine 2009, 20, 553–561.
- Krishna Rao, K. S. V.; Vijaya, K.; Naidu, B.; Subha, M. C. S.; Sairam, M.; Aminabhavi, T. M. Novel Chitosan-Based pH-sensitive Interpenetrating Network Microgels for the Controlled Release of Cefadroxil. Carbohydrate Polymers 2006, 66, 333–344. DOI: 10.1016/j.carbpol.2006.03.025.
- Pranoto, Y.; Lee, M. C.; Park, H. J. Characterizations of Fish Gelatin Films Added with Gellan and K-Carrageenan. LWT-Journal of Food Science and Technology 2007, 40, 766–774. DOI: 10.1016/j.lwt.2006.04.005.
- Ahn, J. H.; Kim, Y. P.; Seo, E. M.; Choi, Y. K.; Kim, H. S. Antioxidant Effect of Natural Plant Extracts on the Microencapsulated High Oleic Sunflower Oil. Journal of Food Engineering 2008, 84, 327–334. DOI: 10.1016/j.jfoodeng.2007.05.029.
- Romeu-Nadal, M.; Chavez-Servin, J. L.; Castellote, A. I.; Riveron, M.; Lopez-Sabater, M. C. Oxidation Stability of the Lipid Fraction in Milk Powder Formulas. Food Chemistry 2006, 100(2), 756–763. DOI: 10.1016/j.foodchem.2005.10.037.
- Shahidi, F.; Wanasundara, U. N. Chemistry, Nutrition and Biotechnology. In Food Lipids; Akoh, C. C., Min, D. B., eds.; Marcel Dekker, Inc.: New York, NY, 2002; pp 465–487.
- Fan, W. J.; Zhang, Y. K.; Chen, Y. C.; Sun, J. X.; Yi, Y. W. TBARS Predictive Models of Pork Sausages Stored at Different Temperatures. Meat Science 2014, 96, 1–4. DOI: 10.1016/j.meatsci.2013.06.025.