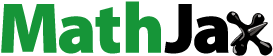
ABSTRACT
This research work was conducted to investigate the impact of critical processing conditions on the selected mechanical properties of maize in the production of fermented ogi slurry. Five varieties of maize (A4W, C3Y, D8W, B2Y, and E9W) were soaked at 28 ºC and average hot soaking at 65ºC, respectively, for 96 h at 12-h interval. Selected mechanical properties were evaluated based on a 5 × 2 × 9 factorial design (varieties× soaking methods× soaking periods). Force (FB) and energy required to break (EB) maize grains decreased significantly (p < 0.05) up to the 12th hour. The EB reduced from 873.3 to 70.0 N mm and from 873.3 to 77.8 N mm for variety E9W at soaking conditions of 28ºC and 65ºC, respectively. Similar trends were observed for other maize varieties. Modulus of elasticity and resilience decreased significantly (p < 0.05) with increase in soaking period and moisture content. The EB to break maize grains was directly proportional to the product of Young’s modulus and area (Em A1.5), the FB and area (Fm A°.5) and force required to break and geometric mean diameter (F Dg2) of maize grains with a high R2 (0.9610.999). This study suggested that the duration of soaking between 12 and 24 h should be enough to significantly (p > 0.05) reduce the hardness, force, and energy required to break whole maize grains in the production of this fermented product and relevant for predicting minimum required energy for a large-scale operation.
Introduction
Ogi is one of the products widely produced from maize in Nigeria,West Africa, and other parts of Africa. Its production involved principally soaking and sedimentation between 24‒72 and 24 h, respectively. [Citation1,Citation2] Studies covering some areas, directly or indirectly linked with ogi production, are abundant in literature. Past reported research works include fermentation process, traditional, modern methods of production, nutritional potentials, chemical composition, social status, and fortification.[Citation1–Citation5] Inyang and Idoko [Citation4] reported findings based on the assessment of ogi quality from malted millet. Drying and rheological properties of ogi were investigated by Adeniji and Potter [Citation5] and Akanbi et al. [Citation3] while storage studies were carried out by some researchers. [Citation2,Citation6]
Despite these studies, the focus of soaking, duration, methods, and temperature effects on the mechanical properties and hardness of maize during the production of ogi are scanty in the literature. These information are useful in the design of relevant handling equipment for large-scale production. In the production of ogi, cereal (maize) is often soaked for the purpose of softening and fermentation. Sourness which is often the outcome of fermentation is not primary in the desire of some consumers. In many cases, sugar, milk, and beverages are usually mixed with pap produced from ogi. “Agidi” (a stiff or thick gruel produced from ogi) is usually eaten with soup, “akara”, and “moinmoin” (product from mixture of cowpea paste, pepper, condiments, palm oil, or vegetable oil and sometimes with egg or fish). Also, there is usually 12–24 h subsequent sedimentation, and this aids further fermentation of sieved “Ogi” slurry. This should take care of consumers who desire tartness. The fact that products processed from ogi slurry are often consumed with various supplements suggest that hardness reduction, is the primary reason for soaking. Three days and more are reported in the literature [Citation1,Citation2,Citation4,Citation6] for this soaking process. This is attempted with an intention to absorb enough water and reduce the grain hardness. The lengthy soaking period may be unnecessary in order to reduce the hardness of grains. However, there is scarcity of information in the literature to suggest this, and thus, the focus of this work.
Grain hardness, which is the resistant to permanent deformation, was seriously implicated as the important factor expected to significantly affect power consumption during milling. [Citation7,Citation8] It was described as the extent of endosperm packing. [Citation7] Hardness may be connected with biochemical interaction between endosperm, storage proteins, and starch granules. [Citation8,Citation9] Several methods were employed in the determination of hardness of agricultural produce. Single-kernel compression instrument equipped with a semiautomated grain feeder was used to crush grains. [Citation9,Citation10] Eckhoff et al. [Citation11] evaluated hardness by shearing individual kernels and recording the associated force breakage curves. Obuchowski and Bushuk [Citation12] determined wheat hardness with the use of abrasive. Stenvert [Citation13] used a Buhler experimental mill to obtain flour and related hardness to the percentage of starch damage. The speed reduction of a mill during grinding was used to determine wheat hardness. [Citation14]
Pomeranz and Williams [Citation15] reported that some environmental factors have tendencies to affect grains hardness. Soil type, planting type, irrigation, fertiliser, cultivation practice, growing season, precipitation and temperature during maturation, protein content, moisture, and kernel size were reported factors that may affect grains hardness. [Citation15–Citation17] Glenn et al. [Citation17] reported the effect of water on the mechanical properties of endosperm. These mechanical properties of food materials may be a heritable trait (16,17,). Cereal grains hardness was reported to have been influenced by kernel protein, vitreousness of grain, size, water-soluble pentosans, moisture content, and lipid content. [Citation8,Citation14,Citation17,Citation18,19]
The need to encourage large-scale production of fermented foods derived from cereal is becoming necessary in African countries for a number of reasons like population increase and increase in working-class mothers. The commercial and industrialisation will inevitably call for standardisation of the process. In large production of ogi, changes of important engineering parameters which application may be relevant in influencing the energy and time reduction as well as design of effective handling equipment need to be investigated. Majority of the research work on physical and engineering properties of agricultural materials did not attempt the evaluation of these parameters at long soaking period and processing conditions attempted in this work.[Citation19]***
Material and methods
Five varieties of dried maize grains, A4W (TZL COM4), C3Y(ACR9931DMRSRY)), D8W(ACR 89 DMR W), B2Y (BR 9928DMRSRY), and E9W (ACR97COM1), were selected based on consistent availability, mild downy, and streak resistance. [Citation20] The “W” and “Y” represent white and yellow maize grains, respectively.[Citation21]*** These were obtained from IITA for this study. These maize varieties were soaked for 12, 24, 36, 48, 60, 72, 84, and 96 h. This was within the range reported in the literature for production of ogi. [Citation2,Citation3,Citation6] Soaking methods of ambient temperature (28ºC) and average temperature of 65ºC (boiled water which later equilibrate with the ambient temperature after 4 h). were employed in accordance to major practice in the production of ogi.
Moisture content and testometric test compression test
The compression test was performed by a testometric universal testing machine (UTM), model Test metric AXM500-25KN. The machine was controlled by a microcomputer. Test results and graphs were automatically generated. The machine was programmed to determine six mechanical properties of the maize seed (peak force, deflection, peak stress, and energy required to fracture maize seed). The maize seeds were randomly compressed by the UTM 50mm/min. The load-deformation curves obtained at each loading orientation and moisture level/soaking period for each variety of maize were analysed for modulus of elasticity, compressive strength, and rapture strength using quations 1, 2, 3, and 4, respectively. [Citation22] The measurement accuracy was ±0.001 N in force and 0.001 mm in deformation. The individual seed was loaded between two parallel plates of the machine and compressed at the preset condition until rupture occurred as denoted by a bio-yield point in the force–deformation curve. The values of all the 40 tests for each maize varieties at respective soaking period and methods were analysed and the average values were reported.
where is the stress, F is the force required to break, is the area of the grain, L is the length, w is the width, and
is the strain
where d (mm) is the displacement of the crosshead. Toughness (Th), is the ratio of energy absorbed (Eb) by the maize seeds to the rupture point to the volume of the seed was calculated from the formula in Equation (5):
Hardness (Hd) was calculated by dividing the average rupture force for each maize varieties at varying soaking period and methods by the respective geometric mean diameter (Dg) as shown in Equation (6)
Data analysis
The data obtained were subjected to statistical analysis using SPSS 16. Analysis of variance was determined and where significant difference existed, Duncan multiple range test was conducted to separate the means. Graphical presentation, regression analysis, interaction effect, and model fits were conducted with the aid of sigma plot version 10.
Results and discussion
Mechanical properties
The result of the maximum force required to break the unsoaked and soaked maize grains is as shown in . The amount of water absorbed was obviously responsible for the noticeable decrease in maximum force required to break. However, the result showed that the water saturation point of these individual maize varieties may not be necessary to keep reducing the force to fracture significantly (p > 0.05). There was a significant reduction of the force to fracture (p < 0.05) for all the maize varieties up to the 12th hour of soaking. The result observed showed that there was consistent reduction in mechanical resistance with increase in the amount of water and these were consistent with the observation of other researchers. [Citation23] The initial force to fracture maize varieties were 758.38, 905.22, 831.63, 700.49, and 743.87 N for maize varieties, E9W, D8W,C3Y, B2Y, and A4W, respectively. The force to fracture in this study for all the maize varieties before and after soaking were all greater than the rupture force obtained by Sirisomboom et al. [Citation23]
There was significant difference (p < 0.05) between the mean force to fracture the unsoaked maize of all the varieties and soaked maize at all the soaking periods. However, there was no significant difference (p > 0.05) between maize soaked at ambient temperature (28 ºC) and maize soaked at average temperature of 65ºC. Exponential linear combination fits the relationship between the maximum force required to break the maize grains and the soaking time with high R2. The mathematical expression is as shown in Equation (7), where, Fm is the maximum force to break, t is the soaking period, a, b, and c are constants which may be linked to intrinsic factors. The value obtained for the mechanical hardness of maize grain soaked at different soaking method and period is as shown in and follows a similar trend of force required to break. The result revealed that maize variety D8W had the highest hardness value which was significantly different (p < 0.05) when compared with unsoaked maize grains. This decreased significantly up to the 12th hour of soaking. There was a linear reduction in the hardness for all the maize varieties with increase in soaking period. According to Henry and Cowe,[Citation24] the hardness of cereal grains may be affected by the structure and position of the endosperm. These factors may indirectly and significantly affect milling and some other unit operations. [Citation25]
The energy required to break maize grains as shown in significantly (p < 0.05) decreased up to the 12th hour and thereafter witnessed insignificant (p > 0.05) decrease for all the maize varieties. The energy to break reduced from 873.29 to 70.01 Nmm and from 873.29 to 77.76 Nmm from maize variety E9W for soaking conditions of 65º and 28ºC, while 907.41‒to 157.02 Nmm and 907.41‒90.61 Nmm, 927.20‒ 160.14 Nmm and 927.20‒98.05 Nmm 824.93‒82.36 Nmm and 824.96‒ 98.91 Nmm and 917.83‒112.82 and 917.83‒104.47 Nmm were obtained for maize varieties A4W, B2Y, C3Y, and D8W at 65ºC and 28ºC soaking conditions, respectively.
The values obtained in this work were relatively lower compared with Sirisomboon et al. [Citation23] and values obtained for calcium-induced egg albumen-flour composite gGel. [Citation26] The predicted energy required to fracture a kilogram of soaked maize grain reduced from 3.34–0.21 kJ/kg and 3.13–0.22- kJ/kg, 3.29–0.29 and 3.35kJ/kg, 3.54–0.33kJ/kg and 3.32–2.52kJ/kg, 2.99 –0.23 and 3.05–0.29kJ/kg, 3.51–0.35kJ/kg and 3.29–0.29kJ/kg (2 decimal points) for maize varieties of E9W, A4W, B2Y,C3Y, and D8W soaked at ambient temperature (28ºC) and 65ºC, respectively. This is as shown in . However, this does not indicate energy consumption during milling to achieve the usual particle sizes in the production of ogi. An exponential linear combination model best fits the energy required to break the maize grains and the soaking time as shown in Equations (8) and (9).
The rupture stress for unsoaked maize for these five varieties are shown in . The least value was recorded for E9W. There was no subsequent significant difference (p > 0.05) in values obtained for soaking beyond the 12th hour for the maize varieties. The rupture stress (N/mm2) obtained in this study were lower compared with the values obtained for calcium-induced egg albumen-flour which ranged from 35.69 to 49.08 KPa. [Citation26] There was no significant difference (p > 0.05) in strain for all the maize varieties at soaking period between 12 and 96 h. The values ranged from 0.22% to 31% for variety D8W for both soaking methods, while 0.19–0.32 and 0.22–0.40 for B2Y and E9W, respectively.
Table 1. Rupture stress (N/mm2) of maize soaked at varying soaking conditions.
The modulus of elasticity is as shown in . The values obtained for unsoaked maize were 14696.72, 21860, 12349.42, 13979.66, and 10248.17 Nmm2 for D8W, B2Y, E9W, C3Y, and A4W, respectively. The result revealed that soaking had a reducing effect on the modulus of elasticity. There was a significant reduction in modulus of elasticity between unsoaked and soaked maize grain up to the 12th hour.
Table 2. Modulus of elasticity (Nmm2) of maize grains at varying soaking conditions.
There was no significant effect (p > 0.05) in the modulus of elasticity between soaking conditions employed. However, the result of modulus of elasticity for unsoaked maize implies tha, the maize variety B2Y will offer a greater resistance to fracture compared with other maize varieties followed by D8W, E9W, C3Y, and A4W. [Citation26–Citation28] The modulus of elasticity decreased subsequently with increase in soaking time. The decrease was, however, non-linear. All the maize grains soaked and unsoaked can be said to present a lower ability to resist force during compression compared with findings of some researchers. [Citation26–Citation29] According to Khodabakhshian,[Citation27] knowledge of apparent elastic properties and elastic modulus of agricultural materials are useful in predicting load-deformation behaviour. The energy absorbed by maize decreased with increase in moisture of the maize grain as shown in . The energy absorbed by maize variety D8W ranged from 67.82 to 163 and from 62.97 to 171.14 J/m3 for ambient soaking and soaking at 65ºC, respectively. The values recorded for maize variety B2Y were in the range of 69.95–126.21 and 87.77–126.09 J/m3, 98.77–149 and 81.55–155.43J/m3 for E9W, 66.49–142.8 and 76.87–163.41 J/m3 for C3Y and for A4W 80.26–114.29 and 87.66–110.01 J/m3 for both hot and ambient soaking, respectively.
Table 3. Energy absorbed (J/m3) by maize grains at varying soaking conditions.
Mathematical expression and interaction effect
Dimensional analysis was applied to establish the relationship between force and energy required to break maize grains and some physical properties. The relationship of force and energy to geometric mean diameter, area, and young modulus was selected based on the high coefficient of determinations (R2), low χ2, and root-mean-square error. The mathematical expressions are as presented in Equations 8–10,where Eb is the energy required to break, Fm is the maximum force required to break, Em, A, and Dg are modulus of elasticity, area, and geometric mean diameter of maize grains, respectively. These mathematical expressions may find application in determining the individual energy required to break maize grains. The interaction effect of force required to break, moisture content and soaking period and force needed to break, moisture content, and geometric mean diameter are as shown in ,, and , respectively. They are quadratic equations and are shown in Equations (11) and (12), respectively. Fm is the force required to break, mc, ts, and Dg are moisture content of grains, soaking period, and geometric mean diameter, respectively. These expressions may be relevant in computing the force and energy required to break a maize grain considering selected processing conditions.
Figure 5. (a) Interaction effect between force required to break, moisture content, and soaking time for maize varieties soaked at ambient temperature (28°C). (b) Interaction effect between force required to break, moisture content and soaking time for maize varieties soaked at ambient temperature (65°C).
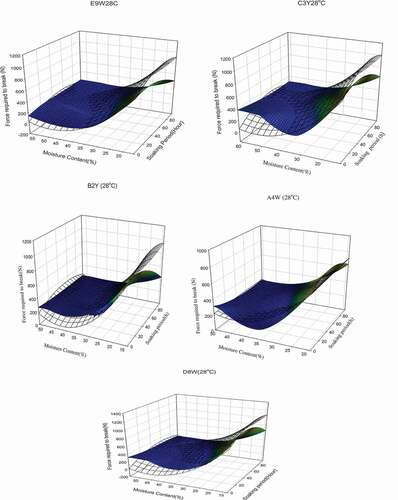
Figure 6. (a) Interaction effect between force required to break, moisture content, and geometric diamter means for maize varieties soaked at ambient temperature (28°C). (b) Interaction effect between force required to break, moisture content, and geometric diameter means for maize varieties soaked at temperature of 65°C.
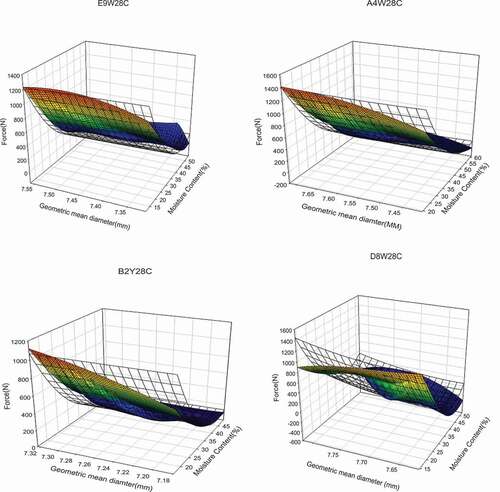
According to Tarighi et al.,[Citation28] the basic concept of studying the engineering properties in food processes is with the aim of designing process, equipment, standardising, and controlling processes to produce food products with specific properties without betraying safety and quality, at reduced time and energy. These mechanical properties are often relevant during the designs, improvement, and optimization of food processes [Citation28]. According to Kerdpiboon et al.,[Citation29], the properties of food materials depend on the nature of product and moisture content. Water absorption characteristics which had been connected with hardness were reported to be dependent on grain geometric size, shapes, and physical structure. [Citation30] Eckhoff et al. [Citation11] reported significant differences in peak force of both soft and hard wheat caused by differences in moisture content at levels of 9%, 11%, and 13%, respectively. Saravacos [Citation31] stated that mechanical compression always reduces the porosity, which subsequently leads to reduction in the effective moisture diffusivity. The water absorbed was obviously responsible for the noticeable decrease in mechanical properties and relationship as revealed in the mathematical expression in Equations 7–12. These mechanical properties also strongly depend on the structure of the material at different levels, including their volume expansion. [Citation25–Citation28] The major determinants of softness and hardness were linked with particle size index [Citation32] and density of gains.[Citation33] According to Armstrong et al.,[Citation33] hardness is often used to reflect the ratio of hard (corneous or vitreous) to soft (starchy) endosperm. [Citation34]This may have contributed to the overall hardness of the maize grains.
The interaction effect between carbohydrates and proteins was reported to strongly influence the processing quality of flour after milling and is closely related with the hardness of endosperm.[Citation35] Density and composition of the material, turgor pressure of cells, cell adhesion, and mode of fracture have been found to affect the mechanical properties of food materials.[Citation36] Additionally, the shell thickness and shell ratio content in seeds may have effect on the rupture force, as observed in sunflower seeds. [Citation37] Mechanical properties can be affected by cell turgor, cell bonding force, cell wall resistance to compression or tensile forces, density of cell packaging, sample size and shape, temperature and strain rate. [Citation38] Penetration of external solutes or solution and physiological cell alteration due to the occurrence of chemical and biochemical reactions may provoke changes in mechanical properties. [Citation38]
The increase in water content was definitely responsible for the interaction effects in , , and , respectively. They were responsible for the grain extensibility (ultimate strain). [Citation37,Citation38] According to Bargale et al.,[Citation39] maximum compressive stress for wheat and canola decreased linearly with an increase in moisture content. The degree of hardness of grain has been linked with the anatomy, structure, and mechanical properties of individual parts of grains. [Citation37–Citation39] Conclusively, Zdunek et al. [Citation40] reported that high turgor may cause high cell wall tension for biological materials, thereby provoke higher resistance to mechanical handling.
Conclusion
This study showed that the duration of soaking beyond 12 h irrespective of the soaking method did not have a significant reduction (p > 0.05) on the hardness, maximum force required to break, modulus of elasticity, and energy required to break wholesome maize grains. There was no significant effect (p > 0.05) of soaking carried out at high temperature of 65ºC on the mechanical properties of soaked maize grains compared with soaking done at ambient temperature of 28°C. Significant effect may have been recorded, if the temperature of boiled water were kept constant throughout the duration of soaking. Also, reliable mathematical expressions with high coefficient of determination between energy required to break (Joules) and soaking period, moisture content of grains, maximum force required to break, modulus of elasticity and hardness, and geometric mean diameter were established in this work.
References
- Odunfa, S. A.; Adeyele, S. Microbial Changes during Traditional Production of Ogibaba, a Western Africa Fermented Sorghum Gruel. Journal Cereal Science 1985, 3, 173–180. DOI: 10.1016/S0733-5210(85)80027-8.
- Teniola, O. D.; Odunfa, S. A. The Effects of Processing Methods on the Level of Lysine, Methionine and the General Acceptability of Ogi Processed Using Starter Cultures. Int. Journal Food Microbiology 2001, 63(1–2), 1–9. DOI: 10.1016/S0168-1605(00)00321-4.
- Akanbi, C. T.; Ade-Omowaye, B. I.; Ojo, A.; Adeyemi, I. A. Effect of Processing Factors on Rheological Properties of Ogi. International Journal of Food Properties 2003, 6(3), 405–418. DOI: 10.1081/JFP-120020118.
- Inyang, C. U.; Idoko, C. A. Assessment of the Quality of Ogi Made from Malted Millet African. Journal of Biotechnology 2006, 5, 22, 2334–2337.
- Adeniji, A. O.; Potter, N. N. Properties of Ogi Powders Made from Normal, Fortified and Opaque-2 Corn. Journal of Food Science 1978, 43, 1571–1574. DOI: 10.1111/jfds.1978.43.issue-5.
- Bolaji, O. T.; Awonorin, S. O.; Olalusi, A. P.; Idowu, M. A.; Henshaw, F. O.; Adepoju, P. A. Effect of Storage Temperature on Some Ogi Properties. Advance Journal of Food Science and Technology 2011, 3, 2, 89–97.
- Kosmolak, F. G. Grinding time-A Screening Test for Kernel Hardness in Wheat. Canadian Journal of Plant Science 1978, 58, 415–420. DOI: 10.4141/cjps78-063.
- Greenblatt, G. A.; Bettge, A. D.; Morris, C. F. The Relationship between Endosperm Texture, and the Occurrence of Friable and Bound Polar Lipids on Wheat Starch. Cereal Chemical 1995, 72, 2, 172–176.
- Elbatawi, I. E.; Arafa, G. K. Factors Affecting Breaking Force Distribution of Wheat Kernel before Milling. Misr Journal of Agricultural Engineering 2008, 25, 3, 1004–1025.
- Sissons, M. J.; Osborne, B. G.; Hare, R. A.; Sissons, S. A.; Jackson, R. Application of the Single-Kernel Characterization System to Durum Wheat Testing and Qualitprediction. Cereal Chemistry 2000, 77, 4–10. DOI: 10.1094/CCHEM.2000.77.1.4.
- Eckhoff, S. R.; Supak, W. A.; Davis, A. B. A Rapid Single Kernel Wheat Hardness Tester. Cereal Chemistry 1988, 65, 503–508.
- Obuchowski, W.; Bushuk, W. Wheat Hardness: Comparison of Methods of Its Evaluation. Cereal Chemistry 1980, 57, 421–425.
- Stenvert, N. L. The Measurement of Wheat Hardness and Its Effect on Milling Characteristics. Aust. Journal Of. Exp. Agricultural Animal Husbandary 1972, 12, 159. DOI: 10.1071/EA9720159.
- Williams, P. C.; Sobering, D. C. Attempts at Standardization of Hardness Testing of Wheat. I. The Grinding/Sieving (Particle Size Index) Method. Cereal Foods World 1986, 31, 359, 362–364.
- Pomeranz, Y.; Williams, P. C. Wheat Hardness: Its Genetic, Structural, and Biochemical Background, Measurement, and Significance. In Advances in Cereal Science and Technology; Pomeranz, Y., ed.; AACCI: St Paul, MN, 1990; Vol. X, pp 471–548.
- Hrušková, M.; Švec, I. Wheat Hardness in Relation to Other Quality Factors. Czech Journal of Food Science 2009, 27(4), 240–248. DOI: 10.17221/71/2009-CJFS.
- Glenn, G. M.; Younce, F. L.; Pitts, M. J. Fundamental Physical Properties Characterizing the Hardness of Wheat Endosperm. Journal of Cereal Science 1991, 13, 179–194. DOI: 10.1016/S0733-5210(09)80035-0.
- Greffeuille, V.; Mabillea, F.; Rousset, M.; Oury, F. X.; Abecassis, J.; Lullien-Pellerin, V. Mechanical Properties of Outer Layers from Near-Isogenic Lines of Common Wheat Differing in Hardness. Journal Cereal Science 2007, 45, 227–235. DOI: 10.1016/j.jcs.2006.09.002.
- Mabille, F.; Gril, J.; Abecassis, J. Mechanical Properties of Wheat Seed Coats. Cereal Chemistry. 2001, 78, 231–235. DOI: 10.1094/CCHEM.2001.78.3.231.
- Adegbite, A. A.; Reaction of Some Maize (Zea Mays L.) Varieties to Infestation with Root-Knot Nematode, Meloidogyne Incognita under Field Conditions. African Journal of Plant Science 2011, 5, 3, 162–167.
- ASAE, ASAE Standard S352-2FEB03. Moisture Measurement. Unground Grain and Seeds; St Joseph Michigan, United State of American, 2003; pp 606.
- Chanvrier, H.; Colonna, P.; Della Valle, G.; Lourdin, D. Structure and Mechanical Behaviour of Corn Flour and Starch/Zeinbased Materials in the Glassy State. Carbohydrate Polymers 2005, 59, 109–119. DOI: 10.1016/j.carbpol.2004.09.005.
- Sirisomboon, P.; Kitchaiya, P.; Pholpho, T.; Mahuttanyavanitch, W. Physical and Mechanical Properties of Jatropha Curcas L. Fruits, Nuts and Kernels. Biosyst. Engineering 2007, 97, 201–207. DOI: 10.1016/j.biosystemseng.2007.02.011.
- Henry, R. J.; Cowe, I. A. Factors Influencing the Hardness (Milling Energy) and Malting Quality of Barley. J.Inst. Brew 1990, 96, 135–136. DOI: 10.1002/j.2050-0416.1990.tb01024.x.
- Bettge, A.; Morris, C. F. Relationships among Grain Hardness, Pentosan Fractions, and End-Use Quality of Wheat. Cereal Chemistry 2000, 77, 241–247. DOI: 10.1094/CCHEM.2000.77.2.241.
- Parichat, H.; Srivikorn, D. Influence of Flour Type on Mechanical Properties of Calcium-Induced Egg Albumen-Flour Composite Gel. International Journal of Food Engineering 2006, 2, 1, 2–7.
- Khodabakhshian, R. Elastic Behavior of Sunflower Seed and Its Kernel. Agricultural Engineering Int: CIGR Journal 2012, 14, 173–178. Open access at http://www.cigrjournal.org.
- Tarighi, J.; Mahmoudi, A.; Alavi, N. Some Mechanical and Physical Properties of Corn Seed (Var. DCC 370). African Journal of Agricultural Research 2011, 6, 16, 3691–3699.
- Kerdpiboon, S.; Kerr, S. L.; Devahastin, S. Neural Network Prediction of Physical Property Changes of Dried Carrot as a Function of Fractal Dimension and Moisture Content. Food Research International 2006, 39(10), 1110–1118. DOI: 10.1016/j.foodres.2006.07.019.
- Lewicki, P. P. Water as the Determinant of Food Engineering Properties. A Review. Journal of Food Engineering 2004, 61(4), 483–495. DOI: 10.1016/S0260-8774(03)00219-X.
- Saravacos, G.; Mass Transfer Properties of Foods. In Engineering Properties of Foods; Rao, M. A., Rizvi, S. S. H., Dalta, A. K., Ed.; Taylor and francis group: New York, 2005. pp 327–350.
- Jolly, C. J.; Rahman, S.; Kortt, A. A.; Higgins, T. J. (1990). Characterization of Grain-Softness Protein, a Marker of Endosperm Texture in Wheat. In: Westcott, T., Williams, Y. (eds), Proceedings of the 40th Australian Cereal Chemistry Conference, Victoria, Australia: The Royal Australian Chemical Institute, pp. 9295. DOI:10.1099/00221287-136-2-327
- Armstrong, P. R.; Lingenfelser, J. E.; McKinney, L. The Effect of Moisture Content on Determining Corn Hardness from Grinding Time, Grinding Energy and Near‐Infrared Spectroscopy. Applied Engineering in Agriculture 2007, 23(6), 793‐799. DOI: 10.13031/2013.24046.
- Landry, L.; Delhaye, S.; Damerval, C. Protein Distribution Pattern in Floury and Vitreous Endosperm of Maize Grain. Cereal Chemistry 2004, 81(2), 153‐158. DOI: 10.1094/CCHEM.2004.81.2.153.
- Peter, J. L.; Vliet, T. V.; de-Velde, F. V. Discussion Session on Solid Food Materials. Food Hydrocolloids 2006, 20(4), 432–437. DOI: 10.1016/j.foodhyd.2005.10.001.
- Waldron, K. W.; Smith, A. C.; Parr, A. J.; Ng, A.; Parker, M. L. New Approaches to Understanding and Controlling Cell Separation in Relation to Fruit and Vegetable Texture. Trends in Food Science and Technology 1997, 8(7), 213–221. DOI: 10.1016/S0924-2244(97)01052-2.
- Denis, L. Coelho, V.; Vear, F. Pericarp Structure and Hullability in Sunflower Inbred Lines and Hybrids. Agronomie 1994, 14(7), 453–461. DOI: 10.1051/agro:19940704.
- Obuchowski, W.; Salmanowic, B.; Banaszak, Z.; Adamski, T.; Surma, M.; Kaczmarek, Z.; Majcher, M.; £Ugowska, B.; Kuczyñska, A.; Krystkowiak, K. Grain Hardness of Wheat Bred in Poland and Its Relationship to Starch Damage during Milling. International Agrophysics 2010, 24, 1, 69–74.
- Bargale, P. C.; Irudayaraj, J.; Bruno, M. Studies on Rheological Behaviour of Canola and Wheat. Journal of Agricultural Engineering Research 1995, 61(4), 267–274. DOI: 10.1006/jaer.1995.1054.
- Zdunek, S.; Gancarz, K. M.; Cybulska, J.; Ranachowski, Z.; Zgórska, K. Turgor and Temperature Effect on Fracture Properties of Potato Tuber (Solanum Tuberosum cv.Irga). International Agrophysics 2008, 22, 1, 89–97.