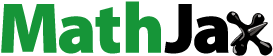
ABSTRACT
Rheological properties and gel characteristics of Sparassis crispa polysaccharides (SCPs) were investigated under various concentrations, temperature, pH, salt concentrations, and sucrose concentrations. SCP solutions behaved as shear thinning pseudoplastic fluids; apparent viscosity increased with concentrations but decreased with extreme conditions and was highest for 1% SCPs at 80 ℃ under neutral conditions; 5% SCPs solutions formed a hysteresis loop and exhibited thixotropic properties. By oscillatory measurements, SCPs were viscoelastic materials. 0.5% and 1% SCPs solutions exhibited viscous behavior at low frequency and enhanced elastic property with the oscillation frequency increased. With the concentration increased to 3% and 5%, the elastic property was predominant in solutions and exhibited gel-like behavior. SCPs gel textural properties and water holding capacity increased with concentration (to 20%) and decreased with salinity, extreme sucrose, and pH. 10% SCPs gels were optimized at 10% sucrose in neutral conditions. Thus, these results implied SCPs had the potential utilization as a new hydrocolloid source in food industries.
Introduction
Sparassis crispa, also called Cauliflower Fungus or Cauliflower Mushroom, is an edible mushroom used for a natural medicine that recently became cultivatable in China, Korea, and Japan. The major component of Sparassis crispa is β-D-glucan, and its content was 39.9% ~ 43.6% of the dry weight of the fruiting bodies.[Citation1–Citation3] β-D-glucan extracted from Sparassis crispa is a well-documented biological response modifier and has been reported to possess multiple pharmacological activities, such as anti-tumor,[Citation4] anti-inflammatory,[Citation5,Citation6] antioxidant,[Citation7] and immunological[Citation8] effects. Rheological properties and gel characteristics of β-glucans are one of the important properties in establishing food texture and processing properties which indirectly reflect the structural and functional changes of β-glucans, they have great value for the design of food processing parameters, engineering calculations and evaluating food qualities when β-glucans used as raw materials of functional foods.
Rheology is a branch of mechanics, and the main research objects of rheology are the deformation and flow of objects under an external force.[Citation9] During the food production and processing, many materials are often in the liquid form. Prediction of their rheological properties can provide parameters for evaluating food quality, formulations as well as processing conditions to optimize food quality.[Citation10] Fungi polysaccharides consisted of chains conformations and formed macromolecular aggregates when dispersed in water due to intermolecular hydrogen bonding. According to the type of flow, most fungi polysaccharides solutions behaved as shear thinning pseudoplastic fluids and showed viscoelastic properties, their rheological properties were affected by the polysaccharides concentrations, temperature, pH, and salt concentrations, for example, the polysaccharides from Ganoderma lucidum,[Citation11] and Agaricus brasiliensis[Citation12] exhibited shear-thinning flow behavior, their viscosities increased with the polysaccharides concentrations increased. However, the viscosities of polysaccharides from Tremella foliacea[Citation13] and Auricularia auricular-judae[Citation14] were decreased with the addition of acid, alkali, and salts. The formation of polysaccharides gels depends on intermolecular hydrogen-bonding interactions, which are generated by intermolecular collisions. During solution storage, the molecules are constantly moving and an increased degree of intermolecular crosslinking leads to gel formation. These hydrophilic colloids formed by polysaccharides are often used as thickening, suspending, binding, and stabilizing agents to improve the food flavor and structure in food industries.[Citation15]
Current studies of Sparassis crispa polysaccharides have mainly focused on functional research[Citation16,Citation17] whereas few have reported the basis of developing functional products especially their changes during processing. Therefore, the objectives of this research were to investigate the effects of concentration, heating temperature, salt, sucrose, and pH on the rheological properties as well as gel characteristics of SCPs. We hypothesize that this information may be useful for developing applications of SCPs used as a new hydrocolloid source in food industries.
Materials and methods
Materials and chemicals
The fruiting bodies of Sparassis crispa obtained from the Edible Fungi Research Center, Shanxi Agricultural University (Shanxi, China) were air-dried at 55°C in an air oven (DHG-9243BS, Shanghai Xingmiao Instrument Co. Ltd., China). The dried samples were milled and sifted through a 200-mesh sieve.
Isolation and purification of SCPs
The powder samples were mixed with distilled water (40:1 mL/g) and extracted at room temperature using an ultrasonic cell disintegrator (TY92-D, Ningbo Xinzhi Bio-Sciences Co. Ltd., China) for 20 min at 1200 w. After sample hydrolysis with 1% cellulase at pH 4 and 1% neutral protease at pH 7 for 3 h, respectively, they were extracted in boiling water twice for 3 h. The extract was centrifuged at 3500 g for 20 min, and the supernatant was concentrated to approximately 100 mL using a rotary evaporator (Büchi Labortechnik AG, Switzerland). The supernatant was precipitated by adding four volumes of anhydrous ethanol. Thereafter, the mixture of supernatant and anhydrous ethanol was maintained at 4 ℃ for 12 h.The precipitate from the ethanol dispersion was collected by centrifuging at 4000 rom (3357g) for 15 min. After that, the collected materials were washed with ether and acetone for three times, respectively. The polysaccharides denuded of proteins using Sevage reagent and then purified using D101 macroporous resin to obtain SCPs. The total carbohydrate, fat, moisture, and ash content of SCPs were 97.11 ± 0.09%, 1.63 ± 0.02%, 1.14 ± 0.00% and 0.11 ± 0.01%, which were determined by the phenol-sulfuric acid method with glucose as a standard, Soxhlet extraction, direct exsiccation, and dry ashing methods, respectively. The molecular weight of SCPs was estimated to 215 Da ~ 393 KDa based on High-performance gel permeation chromatography analysis. The monosaccharide compositions of SCPs were Galactose, Glucose, Xylose, Mannose, and Fructose in the mole ratios of 10.55: 24.76: 2.51: 5.64: 1 based on ion chromatography analysis following complete acid hydrolysis. Its major structural units were a β-(1→3)-D-glucan with a single β-(1→6)-D-glucosyl side branching every three residues based on 1D and 2D NMR spectrum analysis.
Rheological measurement
Steady flow behavior
The effect of polysaccharides concentrations, temperature, NaCl concentration, and pH on SCPs viscosity was investigated using in a rheometer (DHR-3, TA Instruments, USA) equipped with a cone and plate geometry (cone angle 1º; diameter 40 mm) at 25 ℃. Flow curves were obtained, using a steady state flow ramp at 0.1–100 s−1 shear rate. The power-law model was used to describe the experimental flow curves of polysaccharides with the formula: τ = kγn, where τ is the apparent viscosity (Pa· sn), k is the consistency index, and n is the flow behavior index.
Flow properties at different concentrations: SCPs solutions were prepared at concentrations of 0.5%, 1%, 2%, 3%, and 5% by dispersing the required amount in distilled water. The apparent viscosity was measured for shear rates from 0.1 s−1 to 100 s−1 at 25 ℃ in neutral conditions. The rheological parameters at different concentrations were obtained by fitting the shear rate curve.
Flow properties at different temperatures: 1% SCPs solutions were heated to dissolve at 40, 60, 80, 100, and 120 ℃ for 20 min using distilled water in oil bath, then cooled at room temperature. During the experiments, SCPs solutions were covered with silicone oil to prevent water loss. The apparent viscosity was measured for shear rates from 0.1 s−1 to 100 s−1 at 25 ℃ in neutral conditions. The rheological parameters at different heating temperatures were obtained by fitting the shear rate curve.
Flow properties at different pH: Here, 1% SCPs solutions were adjusted to pH values 3, 5, 7, 9, and 11 using 2 M NaOH and HCl before viscosity measurement. The apparent viscosity was measured after different pH adjustments for shear rates from 0.1 s−1 to 100 s−1 at 25 ℃. The rheological parameters at different pH were obtained by fitting the shear rate curve.
Flow properties at different salt concentrations: Monovalent (NaCl) was added to 1% SCPs solutions to give final concentrations of 0%, 1%, 2%, 4%, and 8%. The apparent viscosity was measured after salt adjustment for shear rates from 0.1 s−1 to 100 s−1 at 25 ℃ in neutral conditions. The rheological parameters at different salt concentrations were obtained by fitting the shear rate curve.
Thixotropy property
The up and down curves of shear stress as a function of shear rate from 0.1 s−1 to 100 s−1 were obtained using a two-step Steady-State Flow program for 5% SCPs solutions at room temperature.
Dynamic viscoelastic behavior
The dynamical oscillatory frequency sweep for SCPs solutions with different concentrations (0.5%, 1%, 3%, and 5%) was carried out using a DHR-3 rheometer equipped with a 40 mm, 1°Cone-plate. This measurement was performed to determine the storage (G´) and loss (G´´) moduli of samples at 25 ℃. Frequency sweeps (0.1 Hz ~ 50 Hz at 2% strain) were applied to examine the frequency dependence of G´ and G´´.
Gel measurements
Instrumental Texture tests: Texture profile analysis was conducted at 20 ℃ ~ 25 ℃ using a TMS-PRO Texture Analyzer (Food Technology Co., Sterling, VA) equipped with a 6 mm cylindrical probe. Samples placed at a mounted fixed table were processed with a speed of 60 mm/min up to a depth of 4 mm. During the test, the control force was 0.5 N and each measurement was performed three times.
Texture attributes at different concentrations: SCPs solutions were prepared at concentrations of 1%, 2%, 5%, 10%, and 20% by dispersing in distilled water and then placed into plastic syringes with a diameter of 2 cm and a volume of 20 mL. The bottom and top of the syringes were sealed by rubber pads and plastic films, respectively. Following overnight storage at −4 ℃, the prepared gels in the plastic syringes were released and allowed to stand at room temperature, then cut to a columned sample of 1-cm height (Փ2 cm) to measure texture attributes.
Texture attributes at different salt or sucrose concentrations and pH: To 10% SCPs solutions, monovalent Na (NaCl) was added to yield final concentrations of 0%, 2%, 4%, and 8%; sucrose was added to yield final concentrations of 0%, 5%, 10%, and 20%; or 2 M NaOH and HCl was added to adjust the pH values to approximately 3, 5, 7, 9, and 11.
Water holding capacity (WHC) of SCPs gels
The gels in plastic syringes (Փ2 cm) were released and cut to a columned sample of 1 cm height. The samples placed in a centrifuge tube with a strainer were centrifuged at 4000 g for 20 min.
Microstructure
10% SCPs gels in plastic syringes (Փ2 cm) were released and cut to a columned sample of 1 cm height. The gel was broken after lyophilization and fixed onto a copper stub. The surface of samples was sputtered with a layer of gold and examined using a JSM-6390LV scanning electron microscope (NTC, Japan).
Statistical analysis
All results are reported as the means ± standard deviation (SD) in triplicates. ANOVA was performed using Origin Pro 8.5 software (OriginLab Corporation, MA, USA).
Results and discussion
SCPs rheological properties
The steady shear flow curves of SCPs solutions at different concentrations are presented in . The viscosities of all SCPs solutions decreased significantly with varying shear rate from 0.1 s−1 to 100 s−1 which indicated that SCPs solutions at different concentrations behaved as shear thinning pseudoplastic fluids. As shown in , the coefficients of determination (R2) were higher than 0.93 for all tested samples indicating the appropriateness of the power law model to describe the SCPs solution flow properties. The flow behavior index (n) values of all SCPs concentrations were decreased continuously from 0.80 to 0.26 and the steady shear viscosity of all SCPs solutions increased with concentration from 0.5% to 5%, showing the high dependence of viscosity on concentration. As the polysaccharides concentration became higher, individual molecules began to overlap making the intermolecular connections or forming “junction zones”, which limited polymer chain’s movement and stretching in the aqueous system, therefore the viscosity increased significantly with the increase of concentrations. Similar results and shear-thinning behavior were also exhibited in other mushrooms polysaccharides such as Agaricus brasiliensis[Citation12] and Ganoderma lucidum.[Citation18] The shear-thinning rheological behavior of polysaccharides provides good mouthfeel and can be operated at lower energy due to the lower viscosity at an elevated shear rate, this feature offered us SCPs may be applied to the food industry in the future.
Table 1. Rheological parameters of the power law model for SCPs solutions with different concentrations.
Effect of heating temperature on SCPs flow properties
The apparent viscosities of 1% SCPs solutions at different heating temperatures from 40 ℃ to 120 ℃ are presented in . The viscosities increased and then decreased, with the highest viscosity at a heating temperature of 80 ℃. This suggested that SCPs had good thermal stability below 80 ℃, potentially owing to an ability to maintain its entangled structure. The subsequent decrease over 80 ℃ may result from intensified the kinetic motion of the macromolecules mediated by the high temperature, and thus promoted disentanglement of the chains leading to a decrease in viscosity.
However, the flow behavior index (n) values shown in were less than one even the heating temperature arrived at 120 ℃, indicating the shear thinning pseudoplastic fluid behavior was still retained and not affected by the heating temperature. Furthermore, different polysaccharides exhibit varying thermal stability, such as polysaccharides from A. auricula-judae demonstrated thermal stability at 5 ℃~ 65 ℃,[Citation19] whereas the viscosities of galactomannans decreased by 50% with temperatures through 20 ℃ ~ 80 ℃.[Citation20] In comparison, SCPs solutions exhibited relatively high temperature tolerance.
Table 2. Rheological parameters of the power law model for 1% SCPs solutions with different heating temperatures.
Effect of pH on SCPs flow properties
The effect of pH on apparent viscosities of 1% SCPs solutions are presented in . SCPs solutions viscosities increased and then decreased with the pH values varied from 3 to 11; the viscosities were highest under neutral conditions. Meanwhile, the flow behavior index (n) values given in . were less than 1 and the smallest value was near pH 7, these indicating the shear thinning pseudoplastic fluid behavior of 1% SCPs solutions remained but its apparent viscosities were decreased in acid or alkali conditions. A similar trend was also observed in other polysaccharides aqueous solutions such as xanthan,[Citation21] and guar gum,[Citation20] this was related to the polysaccharide molecular conformation being changed in strong acid and alkaline conditions, in which hydrogen bond breakage promoted polysaccharides decomposition and thus apparent viscosity was decreased.
Table 3. Rheological parameters of the power law model for 1% SCPs solutions with different pH.
Effect of salt concentration on SCPs flow properties
NaCl as a food additive was widely used in food processing and determining the effect of its concentration on apparent viscosity was important for evaluating SCPs rheological properties. The apparent viscosities shown in . were decreased with NaCl concentrations increased from 0% to 8%, The flow behavior index (n) values shown in was less than 1 and the apparent viscosities decreased with the shear rate increased from 0.1 s−1 to 100 s−1, these suggested 1% SCPs solutions with different NaCl concentrations were typical characteristic of pseudoplastic fluids showing a shear thinning flow behavior, the solution behavior was not affected by the addition of NaCl. The effect of the NaCl on apparent viscosity of polysaccharides was different for different component polysaccharides. The apparent viscosity of polysaccharides from Vaccinium bracteatum Thunb leaves,[Citation22] Polygonatum cyrtonema Hua[Citation23] were increased with the addition of NaCl. However, polysaccharides from Auricularia auricular-judae,[Citation14] chlorophytan seaweeds Ulva fasciata[Citation24] were different with these and had the similar trend with our polysaccharides, the apparent viscosity was decreased with NaCl concentrations increased, this was related to the electrostatic interactions dominated in solutions when the salt concentration was low, the polysaccharides molecules existed in a more extended and rigid conformation that resulted in a higher viscosity of the polysaccharides solutions. With the ionic strength increased, the raised concentrations of counter-ions made the molecules contracted and expressed as lower viscosities owing to the charge shielding effect.
Table 4. Rheological parameters of the power law model for 1% SCPs solutions with different NaCl concentrations.
Thixotropy property of SCPs solutions
As shown in , the thixotropy properties of 5% SCPs solutions were investigated with shear rate ranging from 0.1 s−1 to 100 s−1. The forward and backward curves did not coincide and formed a hysteresis loop, indicating thixotropy property of 5% SCPs solutions was exhibited. The presence of thixotropy in 5% SCPs solutions could be attributed to structural changes in aqueous solutions of polysaccharides caused by the generated hydrodynamic forces and the consequent alignment of the molecules in the flow direction. The SCPs solutions developed three-dimensional networks through hydrogen bonding between adjacent hydroxyl and carboxylate groups. When the solutions were sheared, the structure was broken down and infinitesimal layers of SCPs solutions slided onto the adjacent layer resulting in the settling of laminar flux, showing the increase of the shear stress. Likewise, the theory could also be applied to the opposite procedure, with the shear rate decreased, the regeneration of transitional processes would occur. As a characteristic of many pseudoplastic materials, thixotropy can be useful to predict the behavior of food ingredients during mixing, flowing through pipes, and relaxing.[Citation25] Therefore, we concluded SCPs which had good thixotropy properties would be potentially superior for applications in food processing.
Oscillatory measurements
As a highly polymeric material, the main behavior of the polysaccharides on the application of continuous sinusoidal stress exhibited solid elastic and liquid viscous characteristics, referred to as the storage (G´) and loss (G´´) modulus, respectively. As shown in , the modulus G´and G´´ values of SCPs solutions were increased with the frequency increased, showing that the SCPs were viscoelastic materials. The predominance of the viscoelastic behavior through dynamic measurements could be quantified by relative phase angle (tanδ = G´´/ G´, where δ was the loss angle). Tanδ < 1 indicated elastic behavior predominantly; in contrast, tanδ> 1 indicated viscous behavior predominantly. The loss modulus G´´ of 1% and 0.5% SCPs solutions were higher than the storage modulus G´ at low frequency, the value of tan δ was higher than 1, SCPs solutions mainly showed liquid viscosity properties. When the frequency reached a certain value, the curve of G´and G´´ intersected and its crossover frequency was known as the beginning of the elastic behavior or gel state. With the oscillation frequency increased, the storage modulus G´ began to exceed the loss modulus G´´, the value of tan δ was less than 1, the solid elastic property was predominant in SCPs solutions.
Figure 6. Effect of frequency (0.1 ~ 50 Hz) on the storage modulus G´(○), the loss modulus G´´(□) and the phase angle tanδ (▲) at room temperature of different SCPs concentrations. (A) 0.5%; (B) 1%; (C) 3%; (D) 5%.
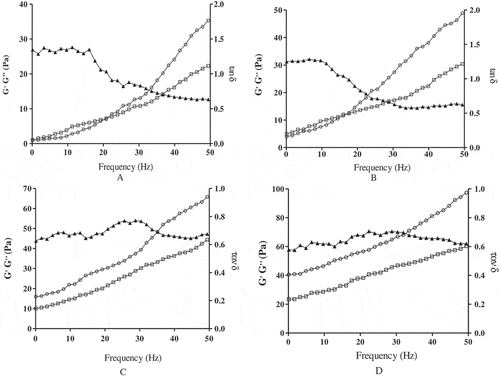
With SCPs concentrations increased, the G´and G´´curves of 3% and 5% had no prominent intersections and G´ was always higher than G´´, the values of tanδ was less than 1, which indicated that the solid elastic property was predominant and could form gel networks to exhibit gel-like behavior. The gel-like behavior of SCPs may be related to its formation of stable molecular chain association and the three-dimensional network structure, in addition, the gel-like behavior was dependent on SCPs concentrations, this may be due to the fact that the structure of SCPs at high concentrations was more stable which could quickly convert the sol fraction to gel to exhibit the stronger gel-like behavior.
Gel properties
Texture properties and WHC of SCPs
Polysaccharides gelation affected the texture of materials in the food processing industry, such as jelly, gelatin, and pudding, moreover polysaccharides gel strength may be affected by various factors, such as the temperature of gel formation, concentration, ionic strength, and pH. In this study, SCPs solutions could form gels overnight at −4 ℃ and SCPs gel properties were closely related to the polysaccharides concentrations as shown in , The texture properties of gels formed by SCPs solutions were not detected with concentrations below 5%. However, at higher concentrations, the polysaccharides gel properties obviously changed, gel strength, elasticity, and WHC increased with the concentration increased from 2% to 20%. Additionally, polysaccharides adhesiveness positively correlated with the concentration. With the concentration increased, the number of network junctions increased and the structure became compact, resulting in enhanced gel strength and WHC.
Table 5. The effect of different SCPs, NaCl, and sucrose concentrations or pH on texture and water holding capacity (WHC) of SCPs gels.
It was found that the addition of NaCl could also affect the gelation of polysaccharides. The texture properties and WHC of SCPs gels were decreased with the NaCl concentrations increased from 0% to 8%. As a strongly polar substance, NaCl exhibited good ability to break hydrogen bonds and enhance competitive hydration, which influenced the solution and aggregation behavior of polymers. The presence of Cl− and Na+ in polysaccharides solutions impacted the molecular structure of polysaccharides and hydrated the electrolytic layers, resulting in a weakened gel formation ability. However, high NaCl concentrations are rarely common in food processing and the conventional addition of NaCl in the processing would not be expected to impact the gel behavior.
The textural properties and WHC of SCPs gels formed by 10% SCPs solutions increased and then decreased with the value of pH increased from 3 to 9; in the neutral environment, the gel properties were highest. With the addition of acid and alkali, the glycosidic and ester bonds of polysaccharides was hydrolyzed, which destroyed the polysaccharides gel structure, leading to gel strength and WHC decreased.
As an important auxiliary material of food processing, the effect of sucrose on SCPs gel behavior was investigated as shown in . The value of gel strength, elasticity, and WHC were increased and then decreased with sucrose concentration varied from 0% to 20%. SCPs gel exhibited the highest gel properties when the addition of sucrose in SCPs solutions was 10%. With the addition of a little sucrose in SCPs solutions, water activity was reduced, leading to the formation of hydrophobic force, which was favorable to form a stable gel network resulting in increased gel strength and WHC. However, excessive sucrose molecules interfered with the contact of polysaccharides molecules and affected the formation of gel network structure, resulting in a decrease of gel strength and WHC. Furthermore, the adhesiveness of the SCPs gel was increased when the sucrose concentration increased from 0% to 20%, which may be related to the stickiness of sucrose itself; thus, the gel adhesiveness became stronger when the sucrose concentrations increased.
Microstructure
The microstructure of 10% SCPs gels is shown in . We observed different size holes distributed on the surface of SCPs gels (). The gel section imaged by scanning electron microscopy exhibited some irregular network structures, and its hole sizes were different (). The results indicated that 10% SCPs solutions could form gels structure overnight at −4 ℃ through the interaction between molecules, however during the freeze-drying process, the space could be left after ice crystals sublimation and formation of crystals caused mechanical damage on the gel network structure and resulted in irregular network structures formed. The gel strength would be affected by gel network structure, the gel structure was generally gotten dense with the number of the network nodes in gel structures increased, which resulted in the gel strength increased.
Conclusion
SCPs were viscoelastic materials, their solutions behaved as shear thinning pseudoplastic fluids as well as exhibited thixotropic and weak gel properties. Their apparent viscosity increased with concentrations but decreased with extreme conditions and was highest for 1% SCPs at 80 ℃ under neutral conditions; 5% SCPs solutions formed a hysteresis loop and exhibited thixotropic properties. 0.5% and 1% SCPs solutions exhibited viscous behavior at low frequency and enhanced elastic property with the oscillation frequency increased. With the concentration increased to 3% and 5%, the elastic property was predominant in solutions and exhibited gel-like behavior. SCPs gel textural properties and water holding capacity increased with concentration (to 20%) and decreased with salinity, extreme sucrose, and pH. 10% SCPs gels were optimized at 10% sucrose in neutral conditions. SCPs thus had the potential utilization as a new hydrocolloid source in food industries. The results can provide reference for the design of food processing parameters and functional food additives in food processing.
Abbreviations
SCPs | = | Sparassis crispa polysaccharides |
G´ | = | storage modulus |
G′′ | = | loss modulus |
N.A. | = | not applicable |
SD | = | standard deviation |
WHC | = | water holding capacity |
Acknowledgments
The research was supported by the Key Research Plan Projects of Shanxi Province (Grant No. 201603D211201), the Key Scientific and Technological Project from Shanxi Province (Grant No. FT2014-03-01) and the Graduate Education Innovation Project of Shanxi Province (Grant No. 2017SY033). The authors declare no conflict of interest.
Additional information
Funding
References
- Harada, T.; Masuda, S.; Arii, M.; Adachi, Y.; Nakajima, M.; Yadomae, T.; Ohno, N. Soy Isoflavone Aglycone Modulates a Hematopoietic Response in Combination with Soluble beta-glucan:SCG. Biological and Pharmaceutical Bulletin 2005, 28, 2342−2345. DOI: 10.1248/bpb.28.2342.
- Kim, M. Y., Chung, I. M., Lee, S. J., Ahn, J. K., Kim, E. H., Kim, M. J., Kim, S. L., Moon, H. I., Ro, H. M., Kang, E. Y., Seo, S. H., & Song, H. K. Comparison of Free Amino Acid, Carbohydrates Concentrations in Korean Edible and Medicinal Mushrooms. Food Chemistry 2009, 113, 386–393. DOI: 10.1016/j.foodchem.2008.07.045.
- Kimura, T. Natural Products and Biological Activity of the Pharmacologically Active Cauliflower Mushroom Sparassis Crispa. Biomedical Research International 2013, 2013, 1-9. DOI: 10.1155/2013/982317.
- Ohno, N.; Miura, N.N.; Nakajima, M.; Yadomae, T. Antitumor 1,3-Beta-Glucan from Cultured Fruit Body of Sparassis Crispa. Biological and Pharmaceutical Bulletin 2000, 23, 866–872. DOI: 10.1248/bpb.23.866.
- Harada, T.; Miura, N.N.; Adachi, Y.; Nakajima, M.; Yadomae, T.; Ohno, N. Highly expressed dectin-1 on bone marrow-derived dendritic cells regulates the sensitivity to beta-glucan in DBA/2 mice. Journal of Interferon & Cytokine Research 2008, 28, 477–486. DOI: 10.1089/jir.2007.0101.
- Ohno, N.; Nameda, S.; Harada, T.; Miura, N.N.; Adachi, Y.; Nakajima, M.; Yoshida, K.; Yoshida, H.; Yadomae, T. Immunomodulating activity of a β-glucan preparation, SCG, extracted from a culinary-medicinal mushroom, Sparassis crispa Wulf.: Fr. (Aphyllophoromycetideae), and application to cancer patients. International Journal of Medicinal Mushrooms 2003, 5, 359–368. DOI: 10.1615/InterJMedicMush.v5.i4.
- Hong, F.; Yan, J.; Baran, J.T.; Allendorf, D.J.; Hansen, R.D.; Ostroff, G.R.; Xing, P.X.; Cheung, N. K.; Ross, G.D. Mechanism by which orally administered beta-1,3-glucans enhance the tumoricidal activity of antitumor monoclonal antibodies in murine tumor models. The Journal of Immunology 2004, 173, 797–806. DOI: 10.4049/jimmunol.173.2.797.
- Harada, T.; Ohno, N. Contribution of dectin-1 and granulocyte macrophage-colony stimulating factor (GM-CSF) to immunomodulating actions of beta-glucan. International Immunopharmacology 2008, 8, 556–566. DOI: 10.1016/j.intimp.2007.12.011.
- Tabilo-Munizaga, G.; Barbosa-Cánovas, G.V. Rheology for the Food Industry. Journal of Food Engineering 2005, 67(1–2), 147–156. DOI: 10.1016/j.jfoodeng.2004.05.062.
- Xu, X.; Xu, J.; Zhang, Y.; Zhang, L. Rheology of Triple Helical Lentinan Insolution: Steady Shear Viscosity and Dynamic Oscillatory Behavior. Food Hydrocolloids 2008, 22(5), 735–741. DOI: 10.1016/j.foodhyd.2007.02.010.
- Fazenda, M.L.; Harvey, L.M.; McNeil, B. Effects of Dissolved Oxygen on Fungal Morphology and proves Rheology during Fed-Batch Processing of Ganoderma Lucidum. Journal of Microbiology and Biotechnology 2010, 20(4), 844–851.
- Gonzaga, M.L.C.; Meneze, T.M.F.; Rebêlo, L.M.; Souza, J.R.R.; Ricardo, N.M.P.S.; Soares, S.D.A. Agaricus brasiliensis mushroom betaglucans solutions: physicochemical properties and griseofulvin solubilisation by self-assembly micro-nano particles formation. Bioactive Carbohydrates and Dietary Fibre 2014, 4(2), 144–154. DOI: 10.1016/j.bcdf.2014.09.004.
- Khondkar, P.; Aidoo, K.E.; Tester, R.F. The Effects of Temperature, pH, and Cations on the Rheological Properties of the Extracellular Polysaccharides of Medicinal Species of Genus Tremella Pers. (Heterobasidiomycetes). International Journal of Medicinal Mushrooms 2010, 12(1), 73–85. DOI: 10.1615/IntJMedMushr.v12.i1.70.
- Bao, H.; You, S.G.; Cao, L.; Zhou, R.; Wang, Q.; Cui, S.W., Chemical and Rheological Properties of Polysaccharides from Fruit Body of Auricularia Auricular-Judae. Food Hydrocolloids 2016, 57, 30–37. DOI: 10.1016/j.foodhyd.2015.12.031.
- Trujillo-Cayado, L.A.; Alfaroa, M.C.; Muñoz, J.; Raymundob, A.; SousabaReología, I., Development and Rheological Properties of Ecological Emulsionsformulated with a Biosolvent and Two Microbial Polysaccharides. Colloids and Surfaces B: Biointerfaces 2016, 141, 53–58. DOI: 10.1016/j.colsurfb.2015.11.046.
- Harada, T.; Kawaminami, H.; Miura, N.N.; Adachi, Y.; Nakajima, M.; Yadomae, T.; Ohno, N. Mechanism of Enhanced Hematopoietic Response by Soluble Beta-Glucan SCG in Cyclophosphamide-Treated Mice. Microbiology and Immunology 2006, 50, 687–700.
- Ikeda, Y.; Adachi, Y.; Ishii, T.; Tamura, H.; Aketagawa, J.; Tanaka, S.; Ohno, N. Blocking effect of anti-Dectin-1 antibodies on the anti-tumor activity of 1,3-beta-glucan and the binding of Dectin-1 to1,3-beta-glucan. Biological and Pharmaceutical Bulletin 2007, 30, 1384–1389. DOI: 10.1248/bpb.30.1384.
- Liu, Y.F.; Zhang, J.S.; Tang, Q.J.; Yang, Y.; Xia, Y.M.; Zhou, S.; Wu, D.; Zhang, Z.; Dong, L.L.; Cui, S.W., Rheological properties of b-D-glucan from the fruiting bodies of Ganoderma lucidum. Food Hydrocolloids 2016, 58, 120–125. DOI: 10.1016/j.foodhyd.2016.01.025.
- Xu, S.; Xu, X.; Zhang, L. Branching structure and chain conformation of water-soluble glucan extracted from Auricularia auricula-judea. Journal of Agricultural and Food Chemistry 2012, 60, 3498–3506. DOI: 10.1021/jf300423z.
- William, P.A.; Phillips, G.O. Introduction to Food Hydrocolloids. In Handbook of Hydrocolloids; Phillips, G.O.; Williams, P.A., Eds.; Woodhead Publishing Limited: Cambridge, 2000; pp 1–19.
- López, M.J.; Vargas-Garcı́a, M.C.; Suarez-Estrella, F.; Moreno, J. Properties of Xanthan Obtained from Agricultural Wastes Acid Hydrolysates. Journal of Food Engineering 2004, 63, 111–115. DOI: 10.1016/S0260-8774(03)00289-9.
- Xu, Q.-X.; Shi, -J.-J.; Zhang, J.-G.; Li, L.; Jiang, L.; Wei, Z.-J. Thermal, Emulsifying and Rheological Properties of Polysaccharides Sequentially Extracted from Vaccinium Bracteatum Thunb Leaves. International Journal of Biological Macromolecules 2016, 93, 1240–1252. DOI: 10.1016/j.ijbiomac.2016.09.098.
- Li, L.; Liao B. Y.; Thakur, K.; Zhang, J. G.; Wei, Z. J. The Rheological Behavior of Polysaccharides Sequential Extracted from Polygonatum cyrtonema Hua. International Journal of Biological Macromolecules 2018, 109, 761–771. DOI: 10.1016/j.ijbiomac.2017.11.063.
- Shao, P.; Qin, M.P.; Han, L.F.; Sun, P.L. Rheology and Characteristics of Sulfated Polysaccharides from Chlorophytan Seaweeds Ulva Fasciata. Carbohydrate Polymers 2014, 113, 365–372. DOI: 10.1016/j.carbpol.2014.07.008.
- Qiao, L.; Li, Y.; Chi, Y.; Ji, Y.; Gao, Y.; Hwang, H.; Aker, W.G.; Wang, P. Rheological Properties, Gelling Behavior and Texture Characteristics of Polysaccharide from Enteromorpha Prolifera. Carbohydrate Polymers 2016, 136, 1307–1314. DOI: 10.1016/j.carbpol.2015.10.030.