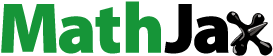
ABSTRACT
In this study, crab shell bioactive peptides were heated with fructose. The antioxidant and antibacterial activities of the crab shell bioactive peptides and the derived Maillard reaction products were evaluated. The results showed that fructose modification was helpful in increasing the antioxidant activity and brought strong antibacterial activity to the crab shell bioactive peptides. The increase of antioxidant activity was a positive correlation with the fructose concentration and pH. And the increase of antibacterial activity was a positive correlation with the reaction pH. In addition, as suggested by the result of molecular weight distribution, the active components in the Maillard reaction product might be the 2–5 kDa fractions. The results indicated that the Maillard reaction was a very effective method for improving the antioxidant and antibacterial activities of bioactive peptides.
Introduction
During the processing of aquatic products, a large amount of waste is produced. Using enzymatic hydrolysis method to produce bioactive peptides is an effective way to utilize these wastes and has gradually become a research hot spot in related fields.[Citation1] Crab is a large marine economic food in the world. Almost 30% of by-products which are mainly crab shells were generated during the processing. Crab shells are rich in protein and many nutrients (such as trace iron, copper, and zinc) and are beneficial to the human body.[Citation2] Using enzymatic hydrolysis method to utilize crab shells and produce antioxidants and antibacterial peptides will effectively improve the application and economic values of the crab.
However, although enzymatic hydrolyzed bioactive peptides have antibacterial or antioxidant activity, there are still some problems with bioactive peptides, such as low antimicrobial activity and easy degradation by proteases. To solve these problems, the Maillard reaction modification is an effective method.[Citation3,Citation4] The Maillard reaction is a reaction between amino compounds (amine, amino acid, peptide, and protein) and carbonyl compounds (carbohydrates) during food processing and storage. It is the main source of the color and aroma for the processed foods and has special meaning in food production. During food processing, the Maillard reaction is often used to improve the functional and flavor properties of proteins or peptides.[Citation5] In addition, through the Maillard reaction, the original bioactivity of the peptides will be enhanced or a new bioactivity property will be obtained, such as enhanced antibacterial activity, antioxidant activity, antihypertensive activity, or anticancer activity.[Citation6–Citation9] The Maillard reaction is a complex system and produces many intermediate products besides melanoidins, such as aldehydes, ketones, and heterocyclic compounds. It has been suggested that the activity of Maillard reaction products (MRPs) is comparable to that of antioxidants or antimicrobials commonly used in food.[Citation8] Karnjanapratum et al. (2016) reported that MRPs derived from gelatin hydrolysate of unicorn leatherjacket skin could be used as alternative antioxidants in food products.[Citation10] Using Maillard reaction-modified crab shell bioactive peptides (CSBPs) can not only improve the activity and stability of the peptides but also effectively prevent peptides from being degraded by proteases. The Maillard reaction will be a good modification method for bioactive peptides.
In this study, proteins in the crab shells were recovered by enzymatic hydrolysis. The effects of Maillard reaction modification on CSBPs were studied for the first time. The antioxidant activity, antibacterial activity, browning intensity, total free amino acid concentration, and molecular weight distribution were estimated. The approach may have practical implications for the effective utilization of crab waste and provide a theoretical basis for further separation and identification of the effective active components in MRPs.
Materials and methods
Materials
A schematic overview of the experimental study is shown in Supplementary Figure S1. Protamex, Flavourzyme, xylose, arabinose, glucose, lactose, fructose, galactose, saccharose, and maltose were purchased from Solarbio (Beijing, China). Ascorbic acid (vitamin C, Vc), 1,1-diphenyl-2-picrylhydrazyl (DPPH), antibiotics, BSA (66.4 kDa), RNase A (13.7 kDa), aprotinin (6.5 kDa), vitamin B12 (1355 Da), and oxidized glutathione (612 Da) were purchased from Sigma–Aldrich (St. Louis, MO, USA). A hydroxyl radical kit, total antioxidant activities (T-AOC) kit, and a total amino acid assay kit were purchased from Nanjing Jiancheng Bioengineering Institute (Nanjing, China). Other chemicals were of analytical grade.
The swimming crab shells were taken directly from the pilot plant transformation line in a sanitary manner. Prior to use, crab shells (legs, claws, and cephalothorax shells) were ground and then stored at −20°C until further analysis. The crude protein content of crab shells was 21.8% which was measured by the Kjeldahl method (Liu et al., 2007).[Citation11] Escherichia coli (ATCC25922), Staphylococcus aureus (ATCC25923), Listeria monocytogenes (ATCC19115), and Vibrio parahaemolyticus (ATCC17802) were used in this study.
Preparation of crab shell bioactive peptides
For the production of CSBPs by protease, the following method was used.[Citation12] Ten grams of crab shell powders were mixed with 80 mL of distilled water and homogenized. The homogenate was first hydrolyzed with Protamex and then hydrolyzed with Flavourzyme under the enzyme’s optimum conditions. The enzyme/substrate ratio was 1:1,000 (w/w). The pH of the slurry was first adjusted to 8.0 by the addition of 1 M NaOH. The pH of the slurry was constantly maintained during hydrolysis by addition of 1 M NaOH. After hydrolysis for 10 h at 45°C, the enzymes were inactivated at 85°C for 10 min. Then, the mixture was cooled to 45°C and pH adjusted to 5.0 by 1 M HCl. Then, the mixture hydrolyzed by Flavourzyme for 10 h. The pH of the slurry was constantly maintained during hydrolysis by addition of 1 M HCl. The enzymes were inactivated at 85°C for 10 min, and the hydrolysate was centrifuged (gravity force of approximately 11,000 × g) in order to separate suspended insoluble solids and lipids from the hydrolysate. The final fractions (soluble peptides) were freeze-dried. These peptide powders were CSBPs and were stored at 4°C.
Maillard reaction and thermal degradation
MRPs were prepared according to the following procedure: 100 mL CSBPs (solid content 10 mg/mL) and 100 mL fructose (5, 10, 20, and 40 mg/mL) were mixed and heated at 100°C. The pH of the mixture was adjusted to 8–12 with NaOH. After reacting for 1 h, the reaction was stopped by cooling the products immediately, and the pH of the mixtures was adjusted to approximately 7.0. The products were freeze-dried and maintained at −20°C until further use. To prepare the thermal degradation products (TDPs), the same methods as described above were used without the addition of fructose.
Browning index
The degree of the Maillard reaction could be shown by the absorbance of the product at 420 nm (Browning index).[Citation13] The higher the browning index was, the more extensive the Maillard reaction is.[Citation14] The absorbance at 420 nm of the MRPs generated under different conditions was measured. Furthermore, the absorbance at 420 nm of the CSBPs (without heating or the addition of fructose) and TDPs was measured as a control.
Antioxidant activity assay
The total antioxidant activities (T-AOC) were measured using a T-AOC kit according to the manufacturer’s instructions. One unit of T-AOC was defined as 1 mg of samples that resulted in an absorbance increase of 0.01 min−1 at 520 nm (with 1 cm optical path length). The T-AOC capacity was calculated as follows:
where n is the dilution ratio of the sample, Acontrol is the absorbance of the control reaction containing all reagents except the sample, Asample is the absorbance of sample reaction with all reagents, Vtotal is the total volume of the reaction, and V is the volume of sample. Thirty is the reaction time. Csample is the concentration of the sample. Ascorbic acid was used as positive control.
The DPPH radical scavenging activity assay was carried out based on a method described by Zhu et al.[Citation15] A volume of 75 μL of sample was added to 150 μL of DPPH solution (0.03% in ethanol) as a free radical source. The mixtures were shaken and then incubated for 15 min in a darkroom at room temperature. Scavenging capacity was measured by monitoring the decrease in absorbance at 517 nm. Ascorbic acid was used as positive control. DPPH radical-scavenging capacity was calculated as follows:
where Acontrol is the absorbance of the control reaction containing all reagents except the sample and Asample is the absorbance of the sample with the DPPH solution.
The hydroxyl radical scavenging assay was carried out based on a method described by Girgih et al.[Citation16] The reaction mixture without any antioxidant was used as the negative control, and the mixture without H2O2 was used as the blank. The hydroxyl radical scavenging activity was calculated as follows based on absorbance change (△A):
The reducing power was determined based on a method described by Ahmadi et al.[Citation17] The samples were dissolved in distilled water to obtain a concentration of 40 mg/mL. An aliquot (0.5 mL) was mixed with 0.5 mL of phosphate buffer (pH 6.6, 0.2 M) and 0.5 mL of 10 mg mL−1 potassium ferricyanide. The mixture was incubated at 50°C for 20 min. Then, 0.5 mL of 10% trichloroacetic acid was added. After centrifugation at 1,500 × g for 10 min, 1.0 mL of the supernatant was collected and mixed with 1.0 mL of distilled water and 0.2 mL of 0.1% (w/v) ferric chloride. After standing at room temperature for 10 min, the absorbance was measured at 700 nm. An equivalent volume of distilled water instead of the sample was used for the blank. An increased absorbance of the mixture indicated the increased reducing power.
Antibacterial effect
Cell suspensions (1 × 107 cfu/mL, 50 μL) were added to agar plates before paper disks (6.0 mm in diameter) containing test solutions were placed on the plates.[Citation18] After incubation at 37°C for 24 h or 48 h, the inhibition zones were measured. E. coli (ATCC25922), S. aureus (ATCC25923), L. monocytogenes (ATCC19115), and V. parahaemolyticus (ATCC17802) were used in this study. The culture media for E. coli and S. aureus was nutrient agar (NA). The culture media for L. monocytogenes was trypticase soy agar with 0.6% yeast extract (TSA-YE). The culture media for V. parahaemolyticus was NA+3% NaOH.
Total free amino acid assay
The total free amino acid concentration was determined by a free amino acid kit according to the manufacturer’s instructions. The absorbance of the reaction was measured at 650 nm. Amino acid mixtures at 50 μM were using as the standard. The reaction mixture without any antioxidant was used as the blank. The hydroxyl radical scavenging activity was calculated as follows:
Molecular weight distribution and antioxidant activity analysis
An HPLC method was used to measure the molecular weight distributions of the samples on a TSKgel G2000SWXL column (7.8 × 300 mm, TOSOH, Japan).[Citation19] Sodium phosphate buffer (0.02 M, pH 7.2) containing 0.25 M NaCl was used as the mobile phase. BSA (66.4 kDa), RNase A (13.7 kDa), aprotinin (6.5 kDa), vitamin B12 (1355 Da), and reduced glutathione (307 Da) (Sigma Co., St. Louis, MO, USA) were used as standards.
The MRPs which had the highest antioxidant activity was passed through TSKgel G2000SWXL column and collected the fractions according to the molecular weight. Fraction Ⅰ was the 2–5 kDa products. Fraction Ⅱ was the 1–2 kDa products. Fraction Ⅲ was the 0.5–1 kDa products. And Fraction Ⅳ was the <0.5 kDa products. These fractions were lyophilized and dissolved in water at a concentrate of 0.1 mg/mL. The DPPH radical scavenging activity of these fractions was analyzed as described above.
Statistical analysis
The data were analyzed using SPSS software (version 18.0). Differences were considered significant at p < 0.05. All experiments were carried out in triplicate, and the results were expressed as the mean ± standard deviation.
Results and discussion
Selection of the optimum reducing sugar
The T-AOC activities of the MRPs generated from the reaction between the CSBPs and different kinds of reducing sugars (xylose, arabinose, glucose, lactose, fructose, galactose, and maltose) at 100°C for 1 h were analyzed. As shown in , after modified by reducing sugars, the T-AOC activity of the MRPs was higher than that of the CSBPs. Moreover, as the kinds of reducing sugars were different, the T-AOC activity-enhancing effect was different. This result was consistent with previous research, which suggested that the Maillard reaction can improve the antioxidant activity of bioactive peptides.[Citation20,Citation21] In addition, the T-AOC activity of the TDPs was decreased, which suggested that the high temperature destroyed the antioxidant component of the CSBPs and reduced the antioxidant activity. In this study, the T-AOC activity of the CSBPs was significantly increased after modification by xylose, arabinose, fructose, and sucrose. As CSBPs modified by fructose had the highest T-AOC activity, fructose was selected for the modification of the CSBPs, and the relationships between antibacterial/antioxidant activity and reaction pH and fructose concentration were further studied.
Influence of the Maillard reaction on the browning index
The browning index of the MRPs generated from the reaction between the CSBPs and different concentrations of fructose at different pH values was analyzed. The results are shown in . As the reaction pH and fructose concentration increased, the browning index of the MRPs was increased. The browning index was a positive correlation with the fructose concentration and reaction pH ( and ). Similar results were also reported by Nie et al.[Citation22] and Hwang et al.,[Citation23] which reported that the browning index also increased as the fructose concentration increased. In addition, it had been reported that the mass ratio of reactants involved in the Maillard reaction was significantly influenced the production of final brown pigments.[Citation24] Luo et al.[Citation25] noted that the UV absorbance of the MRPs from the reaction of chitosan and xylan in a 1:3 mass ratio displayed the sharpest increase at 6 h of heating. In our study, it was to note that the rise of fructose concentration affects the rate and extent of the Maillard reaction, which was consistent with the result of Luo et al.[Citation25]
Figure 2. Browning index of the MRPs generated from CSBPs and fructose. All samples were diluted eight times with demonized water and measured at 420 nm. Bars represent the standard deviation.
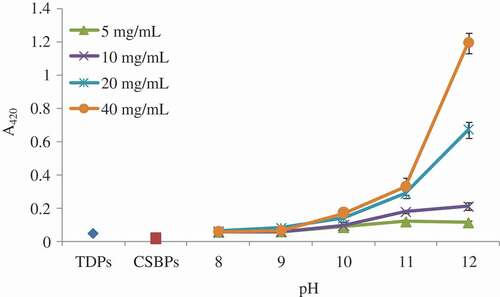
In addition, the browning index of the TDPs was similar to that of the MRPs generated from the CSBPs and fructose at pH 8.0. Browning index is often used to reflect the degree of the Maillard reaction between a protein/peptide and a sugar.[Citation14] The increase in the browning index of the TDPs indicated that the CSBPs might contain reducing sugars. And the Maillard reaction could occur when heating the CSBPs, leading to an increase in the browning index.
Influence of Maillard reaction on antioxidant activities
There are two main methods for evaluating the antioxidant activity of bioactive substances in vitro. One is measuring the ability of the samples to inhibit lipid oxidation. The other is measuring the ability of the samples to remove free radicals.[Citation26] In this study, the antioxidant activities of the CSBPs and MRPs were analyzed using DPPH radical scavenging activity, hydroxyl radical scavenging activity, and reducing power assays. The results are shown in .
Figure 3. Antioxidant activities of the MRPs generated from CSBPs and fructose. Bars represent the standard deviation.
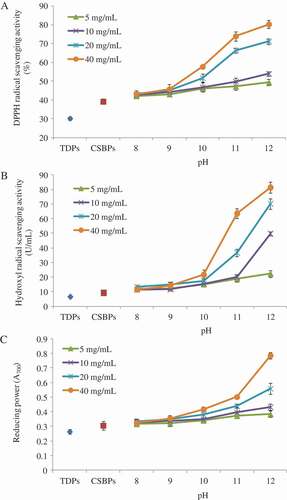
DPPH is a stable free radical which is purple and has a specific absorption peak at 517 nm. DPPH accepts electrons or hydrogen radicals to become a stable diamagnetic molecule and can be reduced by an antioxidant. Removing DPPH can cause decolorization and reduction in the absorbance at 517 nm. The rate of the decrease in color indicates the DPPH scavenging capacity.[Citation27] Thus, the DPPH radical scavenging activity assay is extensively used to evaluate the primary antioxidant activity of antioxidants. This method can accommodate many samples in a short period and is sensitive enough to measure the loss of absorbance at low concentrations.[Citation28] As shown in , the DPPH radical scavenging activity of the MRPs was increased as the fructose concentration and reaction pH increased and was higher than the CSBPs. At pH 8–9, the fructose concentration and reaction pH had little effect on DPPH radical scavenging activity. However, when the reaction pH was higher than 10, the fructose concentration and reaction pH significantly affected the DPPH radical scavenging activity of the MRPs. As the reaction pH increased, the DPPH radical scavenging activity of the MRPs was increased from 46.1% to 80.3%, which was comparable to that of chicken bone peptide MRPs.[Citation22] The results suggested that the Maillard reaction between the CSBPs and fructose could improve the DPPH radical scavenging activity of the CSBPs. And the DPPH radical scavenging activity increasing effect was positively correlated with the fructose concentration and reaction pH ( and ). In addition, the DPPH radical scavenging activity of the MRPs was higher than that of ascorbic acid. In this study, the DPPH radical scavenging activity of 0.1 mg/mL ascorbic acid was 40.7% and that of 0.1 mg/mL CSBPs was 39.2%. The antioxidant activity of CSBPs was similar to the ascorbic acid. Other researchers also reported the similar results.[Citation29] However, at pH 12 and a fructose concentration of 40 mg/mL, the DPPH radical scavenging activity of 0.1 mg/mL MRPs was 80.3%, which was significantly higher than that of ascorbic acid.
Table 2. The correlation coefficient between MRP properties and pH at different fructose concentration.
Table 3. The correlation coefficient between MRP properties and fructose concentration at different pH.
Hydroxyl radicals are the most active and detrimental reactive oxygen species in biological systems.[Citation30] Hydroxyl radicals can easily react with biological molecules such as proteins, amino acids, and deoxyribonucleic acid as well as induce lipid peroxidation.[Citation31] Therefore, hydroxyl radical scavenging is probably one of the most powerful defense mechanisms of living organisms. As shown in , the changing trends in the hydroxyl radical scavenging activity of the MRPs were similar to that of the DPPH radical scavenging activity. The hydroxyl radical scavenging activity of the MRPs increased as the fructose concentration and reaction pH increased and was higher than that of the CSBPs. Similar results were described in the D-xylose-glycine reaction systems.[Citation32] Compared with the DPPH radical scavenging activity, the hydroxyl radical scavenging activity of the CSBPs was relatively low (only 9.1 U/mL). The Maillard reaction greatly enhanced the hydroxyl radical scavenging activity of the CSBPs. The highest hydroxyl radical scavenging activity of the MRPs was 81.3 U/mL, which was nine times higher than that of the CSBPs and significantly higher than that of ascorbic acid (9.8 U/mL).
The reducing power assay is often used to evaluate the ability of an antioxidant to donate an electron or hydrogen. Reducing power serves as a reflection of antioxidant activity, and high reducing power indicates strong antioxidant activity. Many reports have revealed that there is a direct correlation between the antioxidant activity and reducing the power of bioactive compounds.[Citation33] In this study, the ability of CSBPs to reduce Fe3+ to Fe2+ was determined. As shown in , the reducing power changing trends of the MRPs were similar to that of the DPPH radical scavenging activity. The reducing power of the MRPs increased as the fructose concentration and reaction pH increase and was higher than that of the CSBPs. Liu et al.[Citation24] reported that the MRP samples containing a higher level of galactose had a greater reducing power than those with a lower level. The results of this study were consistent with these conclusions. In our study, the reducing power of 0.1 mg/mL ascorbic acid was 0.58, while that of the MRPs was 0.79 (at pH 12 and a fructose concentration of 40 mg/mL). The reducing power of the MRPs was significantly higher than that of ascorbic acid.
In sum, considering all of the abovementioned results together, fructose modification can significantly increase the antioxidant activities of CSBPs. In addition, the antioxidant activity of the MRPs increased as the reaction pH and fructose concentration increasing ( and ). At pH 12 and 40 mg/mL fructose, the antioxidant activities of the MRPs were higher than that of ascorbic acid. Comprehensive analysis of these three kinds of antioxidant activities showed that the MRPs were more sensitive to hydroxyl radicals and has an activity that was 9.3 times higher than that of ascorbic acid. The results suggested that the antioxidant activity of MRPs (fructose-modified CSBPs) was higher than the ascorbic acid, and the MRPs could be used as alternative antioxidants in food production.
Influence of Maillard reaction on antibacterial activity
It has been shown that MRPs generated from peptides and reducing sugars can produce substances with antibacterial activity.[Citation21] In this study, the antibacterial activities of MRPs (at a fructose concentration of 40 mg/mL and in the pH range of 8–12) were analyzed by the inhibition zone method. The antibacterial activities of the CSBPs, TDPs, and MRPs on four common pathogens of aquatic products were detected. As shown in , the CSBPs and TDPs had no obvious antibacterial activity against the four kinds of bacteria. However, the MRPs had an inhibitory effect on all four kinds of bacteria. The antibacterial activity of the MRPs was increased as the reaction pH increased, which meant that the antibacterial activity was a positive correlation with the reaction pH (). Furthermore, the antibacterial activity of the MRPs against gram-negative bacteria was significantly higher than that of the gram-positive bacteria. As the reaction pH increased, the antibacterial activity of the MRPs against gram-positive bacteria was only slightly increased. However, the antibacterial activity against gram-negative bacteria was significantly increased. At pH 12, the inhibition zone of the MRPs for E. coli and V. parahaemolyticus was larger than 15 mm, which was similar to the 20 μg/mL ampicillin. At present, there was little research on the antibacterial activity of Maillard reaction-modified peptide. Song et al.[Citation34] reported that half-fin anchovy hydrolysate-glucose MRPs had strong antibacterial activities on the spoilages from shrimp and crab, which suggested that Maillard reaction could endow peptide antibacterial activity. The results of this study were consistent with these conclusions. In general, Maillard reaction modification could endow the CSBPs with a relatively high antibacterial activity, and the MRPs could inhibit the growth of both gram-positive and gram-negative bacteria.
Table 1. Antibacterial activity of CSBPs, TDPs, and MRPs.
Changes in total free amino acid concentration
It has been reported that, to some extent, the change in free amino acid content reflects the degree of the Maillard reaction.[Citation3] The more free amino acids are consumed, the greater the degree of the Maillard reaction.[Citation4] In this study, as the reaction pH increased, the content of free amino acids showed a trend of rising first and then decreasing (). It might be two possible reasons. First, the CSBPs might be hydrolyzed under alkaline conditions, which would result in an increase in free amino acid content. Second, as the reaction pH increased, the degree of the Maillard reaction was increased, which would lead to the consumption of free amino acids.[Citation35] Considering these two aspects, the free amino acid content increased first and then decreased as the reaction pH increased. The results of the total free amino acid content suggested that the increase in reaction pH and fructose concentration was conducive to the Maillard reaction between the CSBPs and fructose. The result was consistent with that of the browning index.
Molecular weight distributions
The molecular weight distributions of the CSBPs, TDPs, and MRPs are presented in . A typical HPLC chromatogram for the molecular weight distribution of the MRPs and the calibration curve were shown in Supplementary Figure S2. The results showed that the main component of CSBPs was 0.5–1 kDa fractions (46.1%). As the fructose concentration and reaction pH increased, the amount of the 0.5–1 kDa fractions in the CSBPs reduced, and the amount of 2–5 kDa fractions in the MRPs increased, which suggested that the main reaction components in the CSBPs were 0.5–1 kDa fractions. When the pH was 12 and fructose concentration was 40 mg/mL, the 0.5–1 kDa fractions in the CSBPs were almost completely consumed.
Figure 5. The molecular weight distribution of the MRPs generated from CSBPs and fructose. Size exclusion chromatograms using a TSKgel G2000SWXL column at a flow rate of 1.0 mL/min of pH 7.2 sodium phosphate buffer (0.02 M) containing 0.25 M NaCl. Molecular weight distributions of the CSBPs, TDPs, and MRPs were calculated according to the calibration curve. Bars represent the standard deviation.
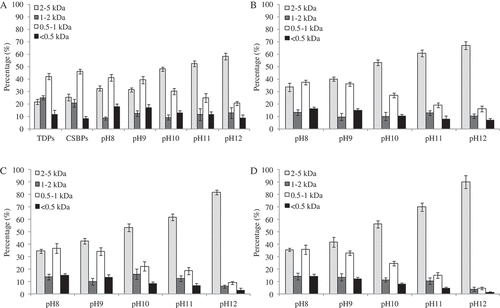
Compared to the CSBPs, the MRPs with molecular weights less than 0.5 kDa showed a tendency to increase first and then decrease with the increase of reaction pH. It might be two possible reasons. First, the CSBPs were hydrolyzed at high temperature, and fractions with molecular weight less than 0.5 kDa were produced. Second, as the reaction pH was low, the degree of Maillard reaction was low, and the consumption of CSBPs fractions with molecular weight less than 0.5 kDa was slow.[Citation36] These two factors led to the result that molecular weights less than 0.5 kDa fractions in MRPs showing a tendency to increase first and then decrease with the increase in reaction pH. For the 1–2 kDa fractions of the MRPs, there was no obvious regularity. Either an increase or decrease was seen as the fructose concentration and reaction pH increased. When the CSBPs reacted with fructose, the CSBPs might hydrolyze, and the macromolecules in the CSBPs would further hydrolyze into small molecules. At the same time, the small molecules in the CSBPs would form products with larger molecular weights. Therefore, there was no obvious regularity of the 1–2 kDa fractions.
In sum, the content of 2–5 kDa fractions in the MRPs was a positive correlation with the pH and fructose concentration ( and ). The content of 0.5–1 kDa and <0.5 kDa fractions in the MRPs was a negative correlation with the pH and fructose concentration ( and ). The results showed that degradation and cross-linking processes simultaneously existed during the Maillard reaction of high-molecular-weight peptides, but the cross-linking process had a leading role under the experimental conditions. It has been reported that owing to peptide cross-linking in the Maillard reaction, the content of high-molecular-weight products tends to increase.[Citation37] The results of this study were consistent with this observation.
Relationship between the antioxidant activity and molecular weight
In this study, the relationship between the antioxidant activity and molecular weight of MRPs was analyzed. The MRPs which had the highest antioxidant activity (at a fructose concentration of 40 mg/mL and pH 12) were passed through a TSKgel G2000SWXL column and collected the fractions according to the molecular weight. FractionⅠ was the 2–5 kDa products. Fraction Ⅱ was the 1–2 kDa products. Fraction Ⅲ was the 0.5–1 kDa products. And Fraction Ⅳ was the <0.5 kDa products. As shown in , the DPPH radical scavenging activities of the fraction Ⅰ, Ⅱ, Ⅲ, and Ⅳ at 0.1 mg/mL were 92.4%, 33.6%, 20.4%, and 53.2%, respectively. Combining the molecular weight distribution results, it could be deduced that the 2–5 kDa fraction in the MRPs was the main active component. As the content of 2–5 kDa fractions was higher, the antioxidant activity of MRPs was higher.
During Maillard reactions, macromolecules are produced due to the cross-linking of reducing sugars with peptides or amino acids. At the same time, proteins or peptides can be degraded into small molecule peptides or free amino acids. The Maillard reaction conditions determine the molecular mass distribution of the final MRPs. The results of this study suggested that peptide degradation and peptide/amino acid cross-linking properties are highly correlated with the molecular weight of the substrates. In addition, these results are in agreement with the findings of Lan et al.[Citation38] and Liu et al.[Citation39] Song et al.[Citation34] found that the highest antibacterial activity of half-fin anchovy hydrolysate-glucose MRPs was found in the 0.5–5 kDa fractions. In this study, the antioxidant and antibacterial activities of the MRPs generated from CSBPs and fructose were increased as the degree of the Maillard reaction increased (). The greater the degree of the Maillard reaction was, the higher the antioxidant and antibacterial activities of the products. According to the correlation analysis, the antioxidant and antibacterial activities of the MRPs were a positive correlation with the browning index and the content of 2–5 kDa fractions (). When the pH was 12 and fructose concentration was 40 mg/mL, the antioxidant and antibacterial components in the MRPs might be the 2–5 kDa fractions. The difference in substrate and reaction conditions of the Maillard reaction determined the complexity of the MRPs. Therefore, the separation and purification of effective antioxidant and antibacterial components in CSBP-fructose MRPs need to be further studied.
Table 4. Correlation analysis among different properties of MRPs at a fructose concentration of 40 mg/mL.
Conclusion
In the present study, the effect of Maillard reaction modification on the CSBPs was investigated. The findings showed that the Maillard reaction modified was helpful in increasing the antioxidant activity of the CSBPs and brought strong antibacterial activity to the CSBPs which had no antibacterial activity originally. In addition, the effects of the Maillard reaction on antioxidant and antibacterial activities were a positive correlation with the fructose concentration and reaction pH. The higher the concentration of fructose and the reaction pH were, the higher the antioxidant and antibacterial activities of the MRPs. In addition, the antioxidant activities of the MRPs were mainly found in the 2–5 kDa fractions. The results indicated that the Maillard reaction was a very effective method for improving the antioxidant and antibacterial activities of bioactive peptides. Due to the complexity of the Maillard reaction and the multiple factors that need to be considered, the antioxidant and antibacterial mechanisms of MRPs need to be further studied.
Supplemental Material
Download ()Supplementary Material
Supplemental data for this article can be accessed here
Additional information
Funding
References
- Beaulieu, L.; Thibodeau, J.; Bonnet, C.; Bryl, P.; Carbonneau, M. É. Detection of Antibacterial Activity in an Enzymatic Hydrolysate Fraction Obtained from Processing of Atlantic Rock Crab (Cancer irroratus) By-Products. Pharmanutrition. 2013, 1, 149–157. DOI: 10.1016/j.phanu.2013.05.004.
- Beaulieu, L.; Thibodeau, J.; Bryl, P.; Carbonneau, M. É. Characterization of Enzymatic Hydrolyzed Snow Crab (Chionoecetes opilio) By-Product Fractions: A Source of High-Valued Biomolecules. Bioresour. Technol. 2009, 100(13), 3332–3342. DOI: 10.1016/j.biortech.2009.01.073.
- Nie, X.; Xu, D.; Zhao, L.; Meng, L. Antioxidant Activities of Chicken Bone Peptide Fractions and Their Maillard Reaction Products: Effects of Different Molecular Weight Distributions. Int. J. Food Prop. 2017, 20(sup1), S457–S466. DOI: 10.1080/10942912.2017.1299176.
- Wang, L.; Jiang, Z.; Tian, B.; Bai, L. N.; Shi, X. H.; Zhang, X. N. Effects of Galactose Concentration on Characteristics of Angiotensin-I-Converting Enzyme Inhibitory Peptides Derived from Bovine Casein in Maillard Reaction. Int. J. Food Prop. 2015, 19(10), 2238–2250. DOI: 10.1080/10942912.2015.1121397.
- Małgorzata, W.; Konrad, P. M.; Zieliński, H. Effect of Roasting Time of Buckwheat Groats on the Formation of Maillard Reaction Products and Antioxidant Capacity. Food Chem. 2016, 196, 355–358. DOI: 10.1016/j.foodchem.2015.09.064.
- Yamabe, N.; Kim, Y. J.; Lee, S. Y.; Cho, E. J.; Park, S. H.; Ham, J.; Kim, H. Y.; Kang, K. S. Increase in Antioxidant and Anticancer Effects of Ginsenoside Re-Lysine Mixture by Maillard Reaction. Food Chem. 2016, 138, 876–883. DOI: 10.1016/j.foodchem.2012.12.004.
- Zhang, R.; Zhang, B. L.; He, T.; Yi, T.; Ynag, T. Y.; He, B. Increase of Rutin Antioxidant Activity by Generating Maillard Reaction Products with Lysine. Bioorg. Med. Chem. Lett. 2016, 26, 2680–2684. DOI: 10.1016/j.bmcl.2016.04.008.
- Wu, S. P.; Hu, J.; Wei, L. T.; Du, L. T.; Shi, X. W. Antioxidant and Antimicrobial Activity of Maillard Reaction Products from Xylan with Chitosan/Chitooligomer/Glucosamine Hydrochloride/Taurine Model Systems. Food Chem. 2014, 148(3), 196–203. DOI: 10.1016/j.foodchem.2013.10.044.
- Li, F. J.; Liu, W. L.; Yamaki, K.; Liu, Y. H.; Fang, Y. Y.; Li, Z. J.; Chen, M. H.; Wang, C. L. Angiotensin I-Converting Enzyme Inhibitory Effect of Chinese Soypaste along Fermentation and Ripening: Contribution of Early Soybean Protein Borne Peptides and Late Maillard Reaction Products. Int. J. Food Prop. 2016, 19(12), 2805–2816. DOI: 10.1080/10942912.2015.1136941.
- Karnjanapratum, S.; Benjakul, S.; Nora, O. Production of Antioxidative Maillard Reaction Product from Gelatin Hydrolysate of Unicorn Leatherjacket Skin. J. Aquat. Food Prod. Technol. 2016, 26(2), 148–162.
- Liu, C.; Wang, H.; Cui, Z.; He, X.; Wang, X.; Zeng, X.; Ma, H. Optimization of Extraction and Isolation for 11S and 7S Globulins of Soybean Seed Storage Protein. Food Chem. 2007, 102, 1310–1316. DOI: 10.1016/j.foodchem.2006.07.017.
- Barkia, A.; Bougatef, A.; Khaled, H. B.; Nasri, M. Antioxidant Activities of Sardinelle Heads and/or Viscera Protein Hydrolysates Prepared by Enzymatic Treatment. J. Food Biochem. 2010, 34, 303–320. DOI: 10.1111/jfbc.2010.34.issue-s1.
- Sun, W. Z.; Zhao, M. M.; Cui, C.; Zhao, Q. Z.; Yang, B. Effect of Maillard Reaction Products Derived from the Hydrolysate of Mechanically Deboned Chicken Residue on the Antioxidant, Textural and Sensory Properties of Cantonese Sausages. Meat Sci. 2010, 86(2), 276–282. DOI: 10.1016/j.meatsci.2010.04.014.
- Ajandouz, E. H.; Tschiape, L. S.; Dalle, O. F.; Benajiba, A.; Puigserver, A. Effects of pH on Caramelization and Maillard Reaction Kinetics in Fructose-Lysine Model Systems. J. Food Sci. 2001, 66(7), 926–931. DOI: 10.1111/j.1365-2621.2001.tb08213.x.
- Zhu, L.; Chen, J.; Tang, X.; Xiong, Y. L. Reducing, Redical Scavenging, and Chelation Properties of in Vitro Digests of Alcalase-Treated Zein Hydrolysate. J. Agric Food Chemi. 2008, 56, 2714–2721. DOI: 10.1021/jf703697e.
- Girgih, A. T.; Udenigwe, C. C.; Aluko, R. E. In Vitro Antioxidant Properties of Hemp Seed (Cannabis sativa L.) Protein Hydrolysate Fractions. J. Am. Oil Chem. Soc. 2011, 88, 381–389. DOI: 10.1007/s11746-010-1686-7.
- Ahmadi, F.; Kadivar, M.; Shahedi, M. Antioxidant Activity of Kelussia Odoratissima Mozaff. In Model and Food Systems. Food Chem. 2007, 105, 57–64.
- Wu, S. P.; Hu, J.; Wei, L. T.; Du, Y. M.; Shi, X. W.; Zhang, L. N. Antioxidant and Antimicrobial Activity of Maillard Reaction Products from Xylan with Chitosan/Chitooligomer/Glucosamine Hydrochloride/Taurine Model Systems. Food Chem. 2014, 148(3), 196–203. DOI: 10.1016/j.foodchem.2013.10.044.
- Jiang, W.; Hu, S. W.; Li, S. J.; Liu, Y. Biochemical and Antioxidant Properties of Peptidic Fraction Generated from Crab (Portunus trituberculatus) Shells by Enzymatic Hydrolysis. Int. J. Food Sci. Technol. 2017, 52(11), 2479–2488. DOI: 10.1111/ijfs.13533.
- Liu, Q.; Kong, B.; Han, J.; Sun, C.; Li, P. Structure and Antioxidant Activity of Whey Protein Isolate Conjugated with Glucose via the Maillard Reaction under Dry-Heating Conditions. Food Struct. 2014, 1(2), 145–154. DOI: 10.1016/j.foostr.2013.11.004.
- Sun, W.; Zhao, M.; Cui, C.; Zhao, Q.; Yang, B. Effect of Maillard Reaction Products Derived from the Hydrolysate of Mechanically Deboned Chicken Residue on the Antioxidant, Textural and Sensory Properties of Cantonese Sausages. Meat Sci. 2010, 86(2), 276–282. DOI: 10.1016/j.meatsci.2010.04.014.
- Nie, X. H.; Xu, D.; Zhao, L. M.; Meng, X. H. Antioxidant Activities of Chicken Bone Peptide Fractions and Their Maillard Reaction Products: Effects of Different Molecular Weight Distributions. Int. J. Food Prop. 2017, 20(31), S457–S466. DOI: 10.1080/10942912.2017.1299176.
- Hwang, I. G.; Kim, H. Y.; Woo, K. S.; Lee, J.; Jeong, H. S. Biological Activities of Maillard Reaction Products (Mrps) in a Sugar-Amino Acid Model System. Food Chem. 2011, 126(1), 221–227. DOI: 10.1016/j.foodchem.2010.10.103.
- Liu, Q.; Niu, H. L.; Zhao, J. Y.; Han, J. C.; Kong, B. H. Effect of the Reactant Ratio on the Characteristics and Antioxidant Activities of Maillard Reaction Products in a Porcine Plasma Protein Hydrolysate-Galactose Model System. Int. J. Food Prop. 2016, 19(1), 99–110. DOI: 10.1080/10942912.2015.1017048.
- Luo, Y. Q.; Ling, Y. Z.; Wang, X. Y.; Han, Y.; Zeng, X. J.; Sun, R. C. Maillard Reaction Products from Chitosan-Xylan Ionic Liquid Solution. Carbohydr. Polym. 2013, 98(1), 835–841. DOI: 10.1016/j.carbpol.2013.06.023.
- Vhangani, L. N.; Van, W. J. Antioxidant Activity of Maillard Reaction Products (Mrps) in a Lipid-Rich Model System. Food Chem. 2016, 208, 301–308. DOI: 10.1016/j.foodchem.2016.03.100.
- Yang, B.; Zhao, M. M.; Shi, J.; Yang, N.; Jiang, Y. M. Effect of Ultrasonic Treatment on the Recovery and DPPH Radical Scavenging Activity of Polysaccharides from Longan Fruit Pericarp. Food Chem. 2008, 106(2), 685–690. DOI: 10.1016/j.foodchem.2007.06.031.
- Brand-Williams, W. M.; Cuvelier, M. E.; Berset, C. Use of Free Radical Method to Evaluate Antioxidant Activity. LWT Food Sci. Technol. 1995, 28, 25–30. DOI: 10.1016/S0023-6438(95)80008-5.
- Yu, M.; He, S.; Tang, M.; Zhang, Z.; Zhu, Y.; Sun, H. Antioxidant Activity and Sensory Characteristics of Maillard Reaction Products Derived from Different Peptide Fractions of Soybean Meal Hydrolysate. Food Chem. 2017, 243, 249–257. DOI: 10.1016/j.foodchem.2017.09.139.
- Li, J. B.; Liang, L.; Cheng, J. R.; Huang, Y. N.; Zhu, M. J. Extraction of Pigment from Sugarcane Juice Alcohol Wastewater and Evaluation of Its Antioxidant and Free Radical Scavenging Activities. Food Sci. Biotechnol. 2012, 21(5), 1489–1496. DOI: 10.1007/s10068-012-0197-8.
- Xie, Z.; Huang, J.; Xu, X.; Jin, Z. Antioxidant Activity of Peptides Isolated from Alfalfa Leaf Protein Hydrolysate. Food Chem. 2008, 111(2), 370–376. DOI: 10.1016/j.foodchem.2008.03.078.
- Shizuuchi, S.; Hayase, F. Antioxidative Activity of the Blue Pigment Formed in a D-Xylose-Glycine Reaction System. J. Agric. Chem. Soc. Jpn. 2003, 67(1), 54–59.
- Guerard, F.; Sumaya-Martinez, M. T.; Laroque, D.; Chabeaud, A.; Dufossé, L. Optimization of Free Radical Scavenging Activity by Response Surface Methodology in the Hydrolysis of Shrimp Processing Discards. Process Biochem. 2007, 42(11), 1486–1491. DOI: 10.1016/j.procbio.2007.07.016.
- Song, R.; Shi, Q.; Yang, P.; Wei, R. Identification of Antibacterial Peptides from Maillard Reaction Products of Half-Fin Anchovy Hydrolysates/Glucose Via, LC-ESI-QTOF-MS Analysis. J. Funct. Foods. 2017, 36, 387–395. DOI: 10.1016/j.jff.2017.07.026.
- Mondacanavarro, B. A.; Ávilavilla, A.; Gonzalezcórdova, A. F.; López-Cervantes, J.; Sánchez-Machado, D. I.; Campas-Baypoli, O. N.; Rodríguez-Ramírez, R. Antioxidant and Chelating Capacity of Maillard Reaction Products in Amino Acid-Sugar Model Systems: Applications for Food Processing. J. Sci. Food Agric. 2017, 97(11), 3522–3529. DOI: 10.1002/jsfa.8206.
- Kim, J. S. Influence of the pH and Enantiomer on the Antioxidant Activity of Maillard Reaction Mixture Solution in the Model Systems. Preventive Nutr. Food Sci. 2010, 15(4), 287–296. DOI: 10.3746/jfn.2010.15.4.287.
- Zamora, R.; Hidalgo, F. J. Coordinate Contribution of Lipid Oxidation and Maillard Reaction to the Nonenzymatic Food Browning. Crit. Rev. Food Sci. Nutr. 2005, 45(1), 49–59. DOI: 10.1080/10408690590900117.
- Lan, X. H.; Liu, P.; Xia, S. Q.; Jia, C. S.; Mukunzi, D; Zhang, X. M.; Xia, W. S.; Tian, H. X.; Xiao, Z. B. Temperature Effect on the Non-Volatile Compounds of Maillard Reaction Products Derived from Xylose-Soybean Peptide System: Further Insights into Thermal Degradation and Cross-Linking. Food Chem. 2010, 120(4), 967–972. DOI: 10.1016/j.foodchem.2009.11.033.
- Liu, P.; Huang, M. G.; Song, S. Q.; Hayat, K.; Zhang, X. M.; Xia, S. Q.; Jia, C. S. Sensory Characteristics and Antioxidant Activities of Maillard Reaction Products from Soy Protein Hydrolysates with Different Molecular Weight Distribution. Food Bioprocess Technol. 2012, 5(5), 1775–1789. DOI: 10.1007/s11947-010-0440-3.