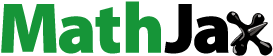
ABSTRACT
This study was designed to evaluate the antioxidant and cholinesterase inhibitory activities of sulfated polysaccharides from Gracilaria gracilis (PGCL) and Ulva lactuca (PULV). PGCL and PULV were extracted and characterized with Scanning electron microscope-energy dispersive x-ray (SEM-EDX), Fourier transform infrared spectroscopy (FTIR) and Gas chromatography-mass spectroscopy (GC-MS). The radical (2,2-azinobis-3-ethylbenzothiazoline-6-sulfonate [ABTS] 2,2-diphenyl-1- picrylhydrazyl [DPPH], and hydroxyl [OH]) scavenging and metal chelating activities as well as their inhibitory effects on acetylcholinesterase (AChE) and butyrylcholinesterase (BChE) activities were also determined. SEM-EDX analysis revealed the presence of elements such as carbon, magnesium, calcium, phosphorus, and sulfur. The FTIR analysis also confirmed the presence of sulfate group in the polysaccharide samples. Some sugars such as glucose, galactose, arabinose, xylose, rhamnose, and allose were also identified using GC-MS. PGCL and PULV scavenged ABTS, DPPH and OH radicals in a dose-dependent manner. Moreover, PULV exhibited higher scavenging activity compared to PGCL. PGCL and PULV also reduced the activity of AChE (IC50 = 132.73 and 106.93 µg/mL) and BChE (IC50 = 124.93 and 93.45 µg/mL) respectively. PGCL and PULV exhibited antioxidant activity and possess cholinesterase inhibitory activity, hence these polysaccharides could be explored as ingredients for the development of functional foods with neuroprotective potentials.
Introduction
Recently there has been a global demand and increase in the consumption of macroalgal foods due to their functional benefits and application in nutrition and health.[Citation1] Algal-derived food products possess nutritional benefits and have been considered as an effective approach for the management and treatment of hyperlipidemia, diabetes, and cardiovascular diseases.[Citation2,Citation3] This is due to the presence of biologically active compounds in algae which include Phlorotannins,[Citation4] polyunsaturated fatty acids,[Citation5] carotenoids,[Citation6] phenolic acids and flavonoids,[Citation7] sterols,[Citation1] and polysaccharides.[Citation8] Polysaccharides are polymers of monosaccharide units that are linked with glycosidic bonds. These polymers are present in the cell wall of macroalgae and differ in their monosaccharide constituents, degree of polymerization, glycosidic bonds and sequence of sugar residues. Some of these polysaccharides consist of sulfate esters linked with polymeric units of galactose, rhamnose, fucose and/or glucose. These polysaccharides exhibit several biological and chemical functions and as well perform protective and structural roles in plants.[Citation9] Polysaccharides that have been identified in brown and green macroalgae include alginate, fucoidans, agar, ulvans, laminaran and carrageenans.[Citation1,Citation9] Some of these polysaccharides including carrageenans, ulvans, and fucoidans exhibit some physiochemical characteristics and are used as thickeners, stabilizers and emulsifiers. Shao et al.[Citation10,Citation11] reported the stabilizing effects of polysaccharides extracted from Ulva fasciata in limonene and beta-carotene emulsions. Algal polysaccharides also exhibit biological features and are good ingredients for the production of nutraceutics, pharmaceutics, and agricultural products. Previous studies have shown that algal polysaccharides exhibit antioxidant,[Citation12], anticancer[Citation13] and anticoagulant activities.[Citation14] However, their neuroprotective effect has not been fully explored.
The use of cholinesterase inhibitors has been identified as an effective strategy to delay the progression of Alzheimer’s disease (AD). These inhibitors improve cholinergic deficit in AD via the inhibition of acetylcholinesterase (AChE) and butyrylcholinesterase (BChE) thereby increasing the levels of acetylcholine and improving its transmission across the neurons (Grossberg, 2003).[Citation15] Although some studies have shown that fucoidans and some other algal polysaccharides helps to improve memory function, there is a paucity of information on their effects on cholinesterases. Hence, the aim of this study is to characterize the polysaccharides in red (Gracilaria gracilis) and green algae (Ulva lactuca) and evaluate their antioxidant and cholinesterase inhibitory activities
Materials and methods
Collection and identification of macroalgal samples
Gracilaria gracilis (Stackhouse) M. Steentoft, L.M. Irvine & W. F. Farnham and Ulva lactuca Linnaeus were obtained from Wild Coast Abalone in East London, South Africa (32°45.048’S. 28°16.558ʹE) and were identified at the Department of Botany of Nelson Mandela University, South Africa. Specimens were deposited in the herbarium and voucher codes D1 and D2 were assigned for G. gracilis and U. lactuca, respectively.
Extraction of sulfated polysaccharides
The polysaccharides extracted from G. gracilis (PGCL), and U. lactuca (PULV) were prepared as described by Gara et al.[Citation16] Briefly 200 g of the dried powder of the seaweeds were de-pigmented with hexane (400 mL) and were allowed to dry. Extraction was done with water at 90–95ºC for 2h. The syrup formed was centrifuged at 4000 g at 25°C for 5 min. The supernatant was collected, allowed to cool and precipitated with three volumes of ethanol. The mixture was kept overnight and precipitate formed was collected by centrifugation and were lyophilized using a freeze dryer (CHRIST Alpha 1–2 LD plus, Germany). The dried samples were stored in vials at 4°C and used for further analysis.
Chemical composition of sulfated polysaccharides
The total sugar content of the sulfated polysaccharides was determined according to phenol-sulfuric acid method described by Chaplin and Kennedy[Citation17] using glucose as the standard. The total protein content of the samples was also determined according to the method of Bradford[Citation18] using bovine serum albumin (BSA) as the standard. Elemental composition of the samples was also determined using scanning electron microscope – energy dispersive x-ray (SEM-EDX) analysis.
Fourier transform infrared analysis
The functional groups in the structure of the sulfated polysaccharides were identified using a Fourier transform infrared spectrophotometer (Spectrum Two Perkin Elmer System, USA). The sulfated polysaccharides (PGCL and PULV) were pressed into a pellet for FTIR spectroscopy analysis over a wavenumber range of 4000–370 cm−1.
Determination of sugar constituents via gas chromatography-mass spectrometry
Sulfated polysaccharide samples (50 mg) were dissolved separately in 2 M trifluoroacetic acid and were incubated at 100°C for 2h. The tubes were allowed to cool down and the hydrolysates formed were dried and used for GC-MS analysis. The dried samples were silylated with pyridine hexamethyldisilazane-trimethylchlosilane mixture (9:3:1 v/v/v) using 50 µL per mg of dried sample. The trimethylsilyl sugar derivatives formed from each polysaccharide sample were analyzed on Gas chromatograph (7890B Agilent, China) equipped with a fused silica capillary column (30 m × 0.25 mm × 0.25 µm) with Mass spectrometry (MS 5977A) using the following conditions; the injected volume was 1 µL; the detector and the injector’s temperature were set at 320ºC, the column temperature was set at 100°C for 1 min and ramped from 100°C to 260°C at 4°C for 1 min and 10 min at 260°C. Helium was used as carrier gas at 1 mL/min.[Citation16] The compounds were identified by comparing the mass spectra with those in the National Institute of Standards and Technology (NIST 2014) library.
ABTS•+ (2,2-azinobis 3-ethylbenzothiazoline-6-sulfonate radical) assay
A modified method of Re et al.[Citation19] was used to determine the total radical scavenging activity of PGCL and PULV. ABTS (7 mM) and K2S2O8 (a final concentration of 2.45 mM) were mixed together and placed in the dark for 16 h to generate ABTS radical. After this, the absorbance of the solution was measured at 734 nm and subsequently adjusted to 0.700 with ethanol. One hundred and fifty microliters of the sulfated polysaccharides (83.33–33.33 μg/mL) were added to 150 μL ABTS•+ solution. The control experiment contained ABTS radical solution without the polysaccharides. After 15 min, the absorbance was measured with a microtiter plate reader at 734 nm. The antioxidant capacity of the polysaccharides was determined based on the decolorization of the ABTS solution. The radical scavenging activity was calculated as the percentage of control using the formula below;
where Abscon is the absorbance of the control experiment without the extracts and Abssam is the absorbance of the test solution containing extract.
DPPH (2,2-diphenyl −1- picrylhydrazyl) radical scavenging assay
One hundred and fifty microliters (150 μL) of the sulfated polysaccharides (83.33–333.33 μg/mL) were mixed with 150 μL of 0.1 mM of DPPH radicals (previously dissolved in methanol). The control contained only the DPPH solution without the test samples.[Citation20] The solution was placed in the dark and the absorbance was measured after 30 min. The radical scavenging activity of the polysaccharides was calculated as percentage of the control.
Hydroxyl radical scavenging assay
The sulfated polysaccharides (25–100 μg/mL) were added to a tube containing deoxyribose (20 mM, 120 μL), phosphate buffer (0.1 M, 400 μL), hydrogen peroxide (20 mM, 40 μL) and FeSO4 (500 μM, 40 μL). The volume of the solution was made up to 800 μL with distilled water and was incubated at 37°C for 30 min. Five hundred microliters of trichloroacetic acid (2.8%) and 400 μL thiobarbituric (0.6%) were added to the solution. The tubes were placed in a boiling water bath (100°C) for 20 min for color development.[Citation21] The absorbance of the solution was measured at 532 nm. The hydroxyl radical scavenging activity of the polysaccharides was calculated using the formula above.
Metal chelating assay
One hundred and fifty microliters of freshly prepared FeSO4 (500 µM) was introduced into a mixture of 168 µL 0.1 M Tris – HCl (pH 7.4), sodium chloride (0.8% (w/v), 218 µL) and the sulfated polysaccharides (20–100 µg/mL). A solution containing 0.25% of 1, 10-phenanthroline (3 µL) was added to the mixture.[Citation22] The absorbance was measured with a spectrophotometer at 510 nm wavelength. The Fe2+ chelating ability was subsequently calculated (Eq. 1).
Cholinesterase activity assay
The acetylcholinesterase (AChE) inhibitory activity of the polysaccharides was evaluated as described by Perry et al.[Citation23] Forty microliter of (0.28 U/mL) enzyme (AChE type V from Electric eel – Sigma Aldrich), 140 µL of 3.3 mM of 5,5-dithiobis-(2-nitrobenzoic) acid (prepared in 0.1 M phosphate buffered solution, pH 7.0, containing 6 mM NaHCO3), sulfated polysaccharides (33.33–133.33 µg/mL) and 80 µL of phosphate buffer (pH 8.0) were added to each well of microplate. The solution was incubated for 20 min at 25°C. Acetylthiocholine iodide (Sigma Aldrich Germany) (0.05 mM, 40 µL) was added to each well and the absorbance was determined in a microtitre plate reader (Synergy MX Biotech) at 412 nm for 3 min immediately after the addition of the substrate. The same experiment was used to determine the butyrylcholinesterase (BChE from Equine Serum-Sigma Aldrich) activity of the polysaccharides using butyrylcholine iodide. The enzyme inhibitory activities were expressed as percentage inhibition.
Results and discussion
Carbohydrate content and elemental composition
The carbohydrate and protein contents of PGCL and PULV are shown in . A higher carbohydrate content was observed in PGCL (33.52 g/100 g) compared to PULV (27.41 g/100 g). However, protein content of PULV (4.30%) was significantly (P < 0.05) higher than PGCL (1.49%). The protein content of PGCL and PULV is significantly higher compared to the sulfated polysaccharide isolated from Ulva clathrate (0.56%).[Citation24] shows the elemental composition of PGCL and PULV. The EDX spectral revealed the presence of carbon, calcium, magnesium, sodium, phosphorus, potassium and sulfur in PGCL and PULV (). Higher levels of carbon (29.49%), phosphorus (2.37%) and potassium (7.18%) were present in PGCL compared to PULV as shown in . However, PULV had significantly higher levels of oxygen (47.87%), sodium (4.56%), magnesium (2.21%), calcium (2.37) and sulfur (9.67%) compared to PGCL. Our findings revealed that both polysaccharide samples contain sulfuryl groups which suggest that they could be sulfated polysaccharides. Moreover, the sulfur content of PULV (9.67%) was similar to purified fucoidan (9.83%) obtained from Fucus vesiculosus.[Citation25] The carbon content of PGCL (29.49%) is significantly higher compared to purified fucoidan (26.12%) of F. vesiculosus.[Citation25]
Table 1. Chemical composition of polysaccharides
Table 2. Average relative values (% weight) for elemental distribution of polysaccharides
Functional group analysis
The functional groups present in PGCL and PULV were identified via FTIR analysis. The IR spectral of the sulfated polysaccharides are shown in . The peaks obtained at 840 cm−1 shows that PGCL and ULV contain compounds with C-S-O group. Both polysaccharides also showed intensity at 1225 cm−1 and 1235 cm−1 which indicates the presence of S = O. This result confirms the presence of sulfate groups in PGCL and PULV. Previous experiment on the chemical constituents of algal polysaccharides has shown that they contain sulfate groups which are formed during the biosynthesis of polysaccharides within the Golgi bodies of algae[Citation26] The presence of sulfate groups in algal polysaccharides have been attributed to their biological activities.[Citation9,Citation27] Peaks with similar intensity were observed at 1608 cm−1 and 1618 cm−1 for PULV and PGCL, respectively. Both wavenumbers indicate the presence of OH groups which is within the spectral range for uronic acid. The peaks observed at 3298 cm−1 and 3364 cm−1 falls within the intensity of signals observed for compounds with O-H groups. Furthermore, the intensity observed at 748 cm−1 for PULV is a typical signal for ulvans.[Citation24] This result suggests that PULV exhibit characteristic of ulvans which are polysaccharides from green algae.
Composition of sulfated polysaccharides
The sugar composition of the polysaccharide samples was analyzed using GC-MS. The presence of some monosaccharides such as glucose, arabinose, galactose, ribose, and xylose were identified in PGCL and PULV as shown in and and respectively. Rhamnose and mannose were present in PULV and were not detected in PGCL. Moreover, allose was also present in PGCL and was not detected in PULV (). The observed level of rhamnose (19.8%) in PULV is significantly higher than the result of Hernandez-Garibay et al.[Citation24] Furthermore, the levels of galactose (4.47%) and xylose (1.01%) were higher in PULV compared with PGCL. However, ribose (7.03%), arabinose (6.89%) and glucose (6.16%) levels were higher in PGCL compared with PULV. The content of galactose and xylose observed in PGCL and PULV were significantly lower compare to the report of Cong et al.[Citation28] in polysaccharide isolated from Sargassum fusiforme. Galacturonic acid, glycopyranuronic acid and arabinonic acid were detected in PGCL but were absent in PULV. The monosaccharide composition of the sulfated polysaccharides revealed that they may contain heterogeneous polymers due to the variation of monosaccharides. Furthermore, the polysaccharides also contain varying levels of sugars.
Table 3. Sugar composition (%) of polysaccharides
Radical scavenging and metal chelating activities
The radical scavenging and metal chelating activities of the sulfated polysaccharides are presented in . PGCL and PULV exhibited scavenging activity against ABTS radical as shown in . Although gallic acid showed higher scavenging activity than the polysaccharides (83.33–333.33 µg/mL), the percentage radical scavenging activity of PGCL (39.75–60.51%) and PULV (39.30–78.34%) were higher than 50% at the highest concentration (333.33 µg/mL) which shows they are potent scavengers of ABTS radical. Moreover, PULV showed higher radical scavenging activities than PGCL with percentage scavenging activity of 78.34% at the highest concentration (333.33 µg/mL). The ABTS radical scavenging activity of sulfated polysaccharides from Sargassum tenerrimum was lower than 50% at the highest concentration (125 µg/mL).[Citation29] Furthermore, depicts the DPPH radical scavenging activities of the polysaccharides. PGCL and PULV scavenged DPPH radicals; however, the activity of both polysaccharides was lower than 50% at the highest concentration (333.33 µg/mL). This result showed that PGCL and PULV showed weak scavenging activity against DPPH radicals compared with gallic acid. The DPPH radical scavenging activity of PGCL and PULV is significantly different from the activity of sulfated polysaccharides isolated from S. tenerrimum.[Citation29]
Figure 5. Radical scavenging and metal chelating activities of polysaccaharides from some seaweeds. PGCL: Gracilaria gracilis; PULV: Ulva lactuca; GAC: Gallic acid
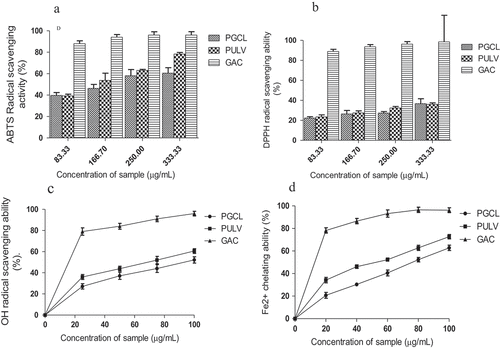
The OH radical scavenging activity of PGCL and PULV is shown in . Both polysaccharides scavenged OH radicals in a dose-dependent manner (25–100 µg/mL). The percentage radical scavenging activity in revealed that PULV (60.6%) had significantly higher scavenging activity against OH radicals compared with PGCL (52.1%) at the highest concentration (100 µg/mL). The OH radical scavenging activity of polysaccharides isolated from Sargassum fulvellum was lower than 50% at the highest concentration (10 mg/mL).[Citation30] The result of the metal chelating capacity of the polysaccharides is presented in . PULV and PGCL were able to chelate Fe2+ in a dose-dependent manner (20–100 µg/mL). The percentage metal chelating activity of both polysaccharides was above 50%. These results suggest that PULV and PGCL are potential metal chelators. Moreover, PULV (72.7%) exhibited significantly higher chelating activity then PGCL (62.8%) at the highest concentration (100 µg/mL) as shown by their percentage chelating activity in . However, previous report revealed that green seaweed (Udotea flabellum) exhibited low metal chelating capacity below 20%.[Citation31] The observed radical and metal chelating activities of PGCL and PULV suggest they are potent antioxidants. Antioxidants are radical scavengers and metal chelators with a capacity to donate an electron. Wang et al.[Citation9] also established that polysaccharides are reducing agent, radical scavengers and metal chelators. The OH radical scavenging and Fe2+- chelating activities of PULV and PGCL suggests their ability to protect cells and tissues from oxidative damage. OH radicals have been reported to be a harmful radical which induces lipid peroxidation in cells and tissues.[Citation32] The radical scavenging and metal chelating activities of PGCL and PULV could be attributed to their protein and sulfate contents. Previous investigations have shown that conjugated proteins to polysaccharides exhibit antioxidant activity via scavenging of OH and superoxide radicals.[Citation33] In another study, Liu et al.[Citation34] attributed the radical scavenging activity of crude polysaccharides from Athyrium multidentaum to its polysaccharide-protein complex. Furthermore, the presence of polyphenols which are conjugated to polysaccharides may also contribute to their antioxidant activity.[Citation35] There are also indications that the presence of sulfate group in the structure of the polysaccharides enhances antioxidant activity compared to non-sulfated polysaccharides.[Citation9]
Cholinesterase inhibitory activity
The effect of PGCL and PULV on acetylcholinesterase and butyrylcholinesterase activities are shown in . PGCL and PULV exhibited an inhibitory effect on acetylcholinesterase in a dose-dependent manner (33–133 µg/mL). Moreover, IC50 values in revealed that PULV (106.93 µg/mL) exhibited significantly higher acetylcholinesterase inhibitory effect compared to PGCL (132.73 µg/mL). Moreover, the acetylcholinesterase inhibitory activity of PGCL and PULV were significantly lower than galathanmine (IC50 = 54.41 µg/mL) (a known cholinesterase inhibitor). Inhibitors of acetylcholinesterase have been identified as an important therapeutic strategy for the management of Alzheimer’s disease. High acetylcholinesterase activity is associated with cholinergic dysfunction and memory impairment in AD.[Citation36] This is due to rapid hydrolysis of acetylcholine, an important neurotransmitter responsible for the transmission of nerve impulse from one neuron to another. Hence, inhibition of acetylcholinesterase increases the availability of acetylcholine which improves neuronal transmission and memory function.[Citation37] The observed acetylcholinesterase inhibitory effect of PGCL and PULV may be beneficial in the management of AD. Our findings agree with the report of Lin et al.[Citation38] which revealed that polysaccharides from a brown alga (Milletia pulchra) reduced acetylcholinesterase activity. An investigation by Park et al.[Citation39] revealed that acetylcholinesterase inhibitory activity of fucoidan extract from Ecklonia cava was higher than 50% at the highest concentration (2 mg/mL) with IC50 value of 1.31 mg/mL. Similarly, PGCL and PULV exhibited an inhibitory effect on butyrylcholinesterase as shown in . The butyrylcholinesterase inhibitory effect of PGCL (124.93 µg/mL) and PULV (93.45 µg/mL) were significantly lower than galathanmine (55.85 µg/mL) as revealed by their IC50 values in . Moreover, butyrylcholinesterase inhibitory activity of PULV was significantly higher than PGCL. High butyrylcholinesterase activity has been associated with formation of amyloid plaques and neurofibrillary tangles around the neurons thus causing neurodegeneration and memory loss.[Citation40] Selective inhibition of butyrylcholinesterase has also been identified as an effective treatment of cholinergic deficit in AD.[Citation41] The observed inhibitory effect of PULV and PGCL may be relevant for the management of AD.
Table 4. IC50 values (µg/mL) cholinesterase inhibitory activity of PGCL and PULV
Conclusion
PGCL and PULV contain monosaccharides such as glucose, galactose, ribose, and arabinose. Both polysaccharides exhibited radical scavenging and metal chelating activities which confirms their antioxidant properties. Furthermore, PGCL and PULV reduced acetylcholinesterase and butyrylcholinesterase activities which could be explored as cholinesterase inhibitors for the management of AD. Our findings suggest that sulfated polysaccharides from Gracilaria gracilis and Ulva lactuca hold promise as important resources of novel ingredients with neuroprotective potentials and could may used for the development of functional foods and nutraceuticals.
Additional information
Funding
References
- Wells, M. L.; Potin, P.; Craigie, J. S.; Raven, J. A.; Merchant, S. S.; Helliwell, K. E.; Smith, A. G.; Camire, M. E.; Brawley, S. H. Algae as Nutritional and Functional Food Sources: Revisiting Our Understanding. J. Appl. Phycol. 2017, 29, 949–982.
- Murray, M.; Dordevic, A. L.; Bonham, M. P.; Ryan, L. Do Marine Algal Polyphenols Have Antidiabetic, Antihyperlipidemic or Anti-Inflammatory Effects in Humans? A Systematic Review. Crit. Rev. Food Sci. Nutr. 2018, 58, 2039–2054. DOI: 10.1080/10408398.2017.1301876.
- Murray, M.; Dordevic, A. L.; Ryan, L.; Bonham, M. P. An Emerging Trend in Functional Foods for the Prevention of Cardiovascular Disease and Diabetes: Marine Algal Polyphenols. Crit. Rev. Food Sci. Nutr. 2018, 58, 1342–1358. DOI: 10.1080/10408398.2016.1259209.
- Thomas, N. V.; Kim, S. K. Potential Pharmacological Applications of Polyphenolic Derivatives from Marine Brown Algae. Environ. Toxicol. Pharmacol. 2011, 32, 325–335. DOI: 10.1016/j.etap.2011.09.004.
- Pereira, H.; Barreira, L.; Figueiredo, F.; Custódio, L.; Vizetto-Duarte, C.; Polo, C.; Rešek, E.; Engelen, A.; Varela, J. Polyunsaturated Fatty Acids of Marine Macroalgae: Potential for Nutritional and Pharmaceutical Applications. Mar. Drugs. 2012, 10, 1920–1935. DOI: 10.3390/md10091920.
- Borowitzka, M.;. Dunaliella: Biology, Production, and Markets. In Handbook of Microalgal Culture; Richmond, A., Hu, Q., Eds.; John Wiley & Sons, Ltd: Chichester, 2013; pp 359–368.
- Tibbetts, S. M.; Milley, J. E.; Lall, S. P. Nutritional Quality of Some Wild and Cultivated Seaweeds: Nutrient Composition, Total Phenolic Content and in Vitro Digestibility. J. Appl. Phycol. 2016, 26, 3575–3585. DOI: 10.1007/s10811-016-0863-y.
- Raposo, M. F. J.; Morais, A. M. B.; Morais, R. M. S. Marine Polysaccharides from Algae with Potential Biomedical Applications. Mar. Drugs. 2015, 13, 2967–3028. DOI: 10.3390/md13052967.
- Wang, J.; Hu, S.; Nie, S.; Yu, Q.; Xie, M. Reviews on Mechanisms of in Vitro Antioxidant Activity of Polysaccharides. Oxid. Med. Cell. Longev. 2016, 5692852, 13.
- Shao, P.; Ma, H.; Qiu, Q.; Jing, W. Physical Stability of R-(+)-Limonene Emulsions Stabilized by Ulva Fasciata Algae Polysaccharide. Int. J. Biol. Macromol. 2016, 92, 926–934. DOI: 10.1016/j.ijbiomac.2016.08.009.
- Shao, P.; Zhang, H.; Niu, B.; Jiang, L. Antibacterial Activities of R- (+)-Limonene Emulsion Stabilized by Ulva Fasciata Polysaccharide for Fruit Preservation. Int. J. Biol. Macromol. 2018, 111, 1273–1280. DOI: 10.1016/j.ijbiomac.2018.01.126.
- Fleita, D.; El-Sayed, M.; Rifaat, D. Evaluation of the Antioxidant Activity of Enzymatically-Hydrolyzed Sulfated Polysaccharides Extracted from Red Algae; Pterocladia Capillace. LWT - Food Sci. Technol. 2015, 63, 1236–1244. DOI: 10.1016/j.lwt.2015.04.024.
- Ahn, G.; Lee, W.; Kim, K.; Lee, J.; Heo, S.; Kang, N.; Lee, S.; Ahn, C.; Jeon, Y. A Sulfated Polysaccharide of Ecklonia Cava Inhibits the Growth of Colon Cancer Cells by Inducing Apoptosis. Excli J. 2015, 14, 294–306. DOI: 10.17179/excli2015-565.
- Faggio, C.; Pagano, M.; Dottore, A.; Genovese, G.; Morabito, M. Evaluation of Anticoagulant Activity of Two Algal Polysaccharides. Nat. Prod. Res. 2016, 30, 1934–1937. DOI: 10.1080/14786419.2015.1086347.
- Grossberg, G. T.;. Cholinesterase Inhibitors for the Treatment of Alzheimer’s Disease. Curr. Ther. Res. Clin. Exp. 2003, 64, 216–235. DOI: 10.1016/S0011-393X(03)00059-6.
- Gara, A. B.; Kolsi, R. B.; Jardak, N.; Chaaben, R.; El-Feki, A.; Fki, L.; Belghith, H.; Belghith, K. Inhibitory Activities of Cystoseira Crinita Sulfated Polysaccharide on Key Enzymes Related to Diabetes and Hypertension: In Vitro and Animal Study. Arch. Physiol. Biochem. 2016, 123(1), 31–42. DOI: 10.1080/13813455.2016.1232737.
- Chaplin, M. F.; Kennedy, J. F. Carbohydrate Analysis, 2nd ed.; Oxford University Press: New York, NY, USA, 1994.
- Bradford, M. M.;. A Rapid and Sensitive Method for the Quantitation of Microgram Quantities of Protein Utilizing the Principle of Protein-Dye Binding. Anal. Biochem. 1976, 72, 248–254. DOI: 10.1016/0003-2697(76)90527-3.
- Re, R.; Pellegrini, N.; Proteggente, A.; Pannala, A.; Yang, M.; Rice-Evans, C. Antioxidant Activity Applying an Improved ABTS Radical Cation Decolorisation Assay. Free Radical Biol. Med. 1999, 26, 1231–1237.
- Gyamfi, M. A.; Yonamine, M.; Aniya, Y. Free Radical Scavenging Action of Medicinal Herbs from Ghana: Thonningia Sanguinea on Experimentally Induced Liver Injuries. Gen. Pharmacol. 1999, 32, 661–667.
- Halliwell, B.; Gutteridge, J. M. C. Formation of Thiobarbituric-Acid-Reactive Substance from Deoxyribose in the Presence of Iron Salts: The Role of Superoxide and Hydroxyl Radicals. FEBS Lett. 1981, 128, 347–352.
- Puntel, R. L.; Nogueira, C. W.; Rocha, J. B. T. Krebs Cycle Intermediates Modulate Thiobarbituric Acid Reactive Species (TBARS) Production in Rat Brain in Vitro. Neurochem. Res. 2005, 30, 225–235.
- Perry, N. S.; Houghton, P. J.; Theobald, A.; Jenner, P.; Perry, E. K. In Vitro Activity of Slavandula Efolia (Spanish Sage) Relevant to Treatment of Alzheimer’s Disease. J. Pharm. Pharmacol. 2000, 52, 895–902.
- Hernández-Garibay, H.; Zertuche-González, J. A.; Pacheco-Ruíz, I. Isolation and Chemical Characterization of Algal Polysaccharides from the Green Seaweed Ulva Clathrata (Roth) C. Agardh. J. Appl. Phycol. 2011, 23, 537–542. DOI: 10.1007/s10811-010-9629-0.
- Zayed, A.; Muffler, K.; Hahn, T.; Rupp, S.; Finkelmeier, D.; Burger-Kentischer, A.; Ulber, R. Physicochemical and Biological Characterization of Fucoidan from Fucus Vesiculosus Purified by Dye Affinity Chromatography. Mar. Drugs. 2016, 14, 79. DOI: 10.3390/md14040075.
- McCandless, E.; Craigie, J. Sulfated Polysaccharides in Red and Brown Algae. Annu. Rev. Plant Biol. 1979, 30, 41–53. DOI: 10.1146/annurev.pp.30.060179.000353.
- Barahonaa, T.; Encinasa, M. V.; Imaraib, M.; Mansillac, A.; Matsuhiroa, N. B.; Torresa, R.; Valenzuela, B. Bioactive Polysaccharides from Marine Algae. Bioact. Carbohydr. Diet. Fibre. 2014, 125–138. DOI: 10.1016/j.bcdf.2014.09.002.
- Cong, Q.; Chen, H.; Liao, W.; Xiao, F.; Wang, P.; Qin, Y.; Dong, Q.; Ding, K. Structural Characterization and Effect on Anti-Angiogenic Activity of a Fucoidan from Sargassum Fusiforme. Carbohydr. Polym. 2016, 136, 899–907. DOI: 10.1016/j.carbpol.2015.09.087.
- Raguraman, V.; Abraham, S.; Jyotsna, J.; Palaniappan, S.; Gopal, S.; Thirugnanasambandam, R.; Kirubagaran, R. Sulfated Polysaccharide from Sargassum Tenerrimum Attenuates Oxidative Stress Induced Reactive Oxygen Species Production in In Vitro and in Zebrafish Model. Carbohydr. Polym. 2019, 203, 441–449. DOI: 10.1016/j.carbpol.2018.09.056.
- Kim, S.; Choi, D.; Athukorala, Y.; Jeon, Y.; Senevirathne, M.; Rha, C. K. Antioxidant Activity of Sulfated Polysaccharides Isolated from Sargassum Fulvellum. Preventive Nutr. Food Sci. 2007, 12, 65–73. DOI: 10.3746/jfn.2007.12.2.065.
- Presa, F. B.; Marques, M. L. M.; Viana, R. L. S.; Nobre, L. T. D. B.; Costa, L. S.; Rocha, H. A. O. The Protective Role of Sulfated Polysaccharides from Green Seaweed Udotea Flabellum in Cells Exposed to Oxidative Damage. Mar. Drugs. 2018, 16, 135. DOI: 10.3390/md16040135.
- Valko, M.; Lebfritz, D.; Moncol, J.; Cronin, M. T.; Mazur, M.; Telser, J. Free Radicals and Antioxidants in Normal Physiological Functions and Human Disease. Int. J. Biochem. Cell Biol. 2007, 39, 44–84. DOI: 10.1016/j.biocel.2006.07.001.
- Liu, F.; Ooi, V. E. C.; Chang, S. T. Free Radical Scavenging Activities of Mushroom Polysaccharide Extracts. Life Sci. 1997, 60, 763–771. DOI: 10.1016/S0024-3205(97)00004-0.
- Liu, D.; Sheng, J.; Li, Z.; Qi, H.; Sun, Y.; Duan, Y.; Zhang, W. Antioxidant Activity of Polysaccharide Fractions Extracted from Athyrium Multidentatum (Doll.) Ching. Int. J. Biol. Macromol. 2013, 56, 1–5. DOI: 10.1016/j.ijbiomac.2013.02.014.
- Renard, C. M.; Baron, A.; Guyot, S.; Drilleau, J. F. “Interactions between Apple Cell Walls and Native Apple Polyphenols: Quantifcation and Some Consequences. Int. J. Biol. Macromol. 2001, 29, 115–125. DOI: 10.1016/S0141-8130(01)00155-6.
- García-Ayllón, M.; Riba-Llena, I.; Serra-Basante, C.; Alom, J.; Boopathy, R.; Sáez-Valero, J. Altered Levels of Acetylcholinesterase in Alzheimer Plasma. PLoS One. 2010, 5, e8701. DOI: 10.1371/journal.pone.0008701.
- Čolović, M. B.; Krstić, D. J.; Lazarević-Pašti, T. D.; Bondžić, A. M.; Vasi, V. M. Acetylcholinesterase Inhibitors: Pharmacology and Toxicology. Curr. Neuropharmacol. 2013, 315–335. DOI: 10.2174/1570159X11311030006.
- Lin, X.; Huang, Z. S.; Chen, X. Y.; Rong, Y. P.; Zhang, S. J.; Jiao, Y.; Huang, Q. F.; Huang, R. B. Protective Effect of Millettia Pulchra Polysaccharide on Cognitive Impairment Induced by D-Galactose in Mice. Carbohydr. Polym. 2014, 101, 533–543. DOI: 10.1016/j.carbpol.2013.09.037.
- Park, S. K.; Kang, J. Y.; Kim, J. M.; Park, S. H.; Kwon, B. K.; Kim, G.; Heo, H. J. Protective Effect of Fucoidan Extract from Ecklonia Cava on Hydrogen Peroxide-Induced Neurotoxicity. J. Microbiol. Biotechnol. 2018, 28, 40–49.
- Greig, N. H.; Lahiri, D. K.; Sambamurti, K. Butyrylcholinesterase: An Important New Target in Alzheimer’s Disease Therapy. Int. Psychogeriatr. 2002, 14, 77–91. DOI: 10.1017/S1041610203008676.
- Greig, N. H.; Utsuki, T.; Ingram, D. K.; Wang, Y.; Pepeu, G.; Scali, C.; Yu, Q.; Mamczarz, J.; Holloway, H. W.; Giordano, T.; et al. Selective Butyrylcholinesterase Inhibition Elevates Brain Acetylcholine, Augments Learning and Lowers Alzheimer β-amyloid Peptide in Rodent. Proc. Natl. Acad. Sci. U S A. 2005, 102, 17213–17218. DOI: 10.1073/pnas.0508575102.