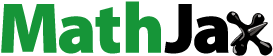
ABSTRACT
Two plant enzymes, namely actinidin (A) and papain (P), were used to pretreat bovine skin at the respective optimum pH and temperature of the enzymes for 48 h at the level of 0, 5, 10, 15, 20, and 25 unit/g of skin, and gelatin extraction was done at 60°C for 6 h. Gelatin yield from actinidin at level 20 (A20) (22.67%) and papain at level 20 (P20) (23.59%) were significantly (P < 0.05) higher than control (17.90%). The gel strength values for gelatin extracted using actinidin enzyme (GEA) were significantly (P < 0.05) higher than the control (283.35 g), and the gel strength for A20 was 366.39 g. However, gelatin extracted using papain enzyme (GEP) showed relatively lower gel strength. The GEA sample viscosities were significantly (P < 0.05) higher than control (12.10 mPa.s). GEA samples revealed overall degradation of β chains and presence of α chains and lower molecular weight peptides, whereas β and α chains were completely absent and lower molecular weight peptides were seen in all the GEP samples. Fourier-transform infrared (FTIR) spectra indicated a greater loss of molecular order and more disruption in the α helical structure of P20 compared to A20 and control gelatins. Scanning electron microscopy (SEM) revealed interconnected bigger particle size and denser structure with least number of voids in A20 than P20 and control gelatin samples. Thus, it was concluded that actinidin, particularly at level 20 unit/g of skin, could be used to improve the yield and properties of gelatin from bovine skin.
Introduction
Gelatin being derived from collagen by its limited denaturation possesses high-molecular-weight biopolymer.[Citation1] It shows various functional properties including film-forming ability, water-binding capacity, foaming, and emulsifying properties, qualifying it as an important constituent in food, medicine, pharmacy, photography, and cosmetic.[Citation2,Citation3] Collagen is made up of three intertwined α-chains to form a very stable right-handed triple-helix structural protein[Citation4] and the hydrogen bonding between the chains provide the required stability to the triple-helical collagen structure.[Citation5,Citation6]
A pretreatment process using either acid or alkali is required to convert insoluble collagen into soluble form. This results in insoluble swollen collagen with lost native collagen structure.[Citation7] During the gelatin extraction process, heat destroys the hydrogen and covalent bonds, thereby changing the triple-helical structure of collagen chains to random coil structure (helix-to-coil transition) resulting in gelatin production.[Citation8] Noncovalent and covalent bonds are broken down to enable to release free oligomers as well as α chains.[Citation5] In addition to it, some amide bonds are cleaved in the native collagen structure during hydrolysis.[Citation9] Therefore, the resultant gelatin contains a combination of lower molecular weight polypeptides, showing a molecular weight between 16 and 150 kDa.
Crosslink bonds found in collagen triple-helical structure are resistant to heat and acid,[,Citation11] and therefore, normally a low gelatin yield is obtained.[Citation12] Earlier, few protease enzymes known to cleave crosslinked bonds of collagen were used to enhance the yield of gelatin.[Citation12] Proctase extracted from Aspergillus niger and pepsin were employed for gelatin extraction from bovine skin, but the yield was comparatively low and the gelatin recovered so exhibited low gel strength and viscosity.[Citation13]
Plant enzymes like papain and bromelain have been employed for gelatin extraction from the collagenous source. The gelatin source materials were initially pretreated with various proteases prior to gelatin extraction.[Citation12–Citation16]On the other hand, Pitpreecha and Damrongsakkul[Citation17] and Damrongsakkul et al.[Citation18]extracted gelatin from bovine skin without pretreating the skin with papain enzyme. Pitpreecha and Damrongsakkul[Citation17] used papaya latex-derived crude proteolytic enzyme and commercial papain enzyme to extract gelatin from the rawhide at the optimum conditions (75°C and pH 7) for the activities of both the enzymes and higher gelatin recovery was achieved, but both gelatin samples revealed absolutely degraded α and β chains possessing relatively low gel strength. Damrongsakkul et al.[Citation18] used papain to extract gelatin from rawhide splits and found low gel strength and viscosity of the obtained gelatin. Norziah et al.[Citation19] pretreated the surimi processing waste from ribbon fish (Lepturacanthus savel) before extracting gelatin with bromelain at 4°C which was not the optimum activity temperature of the enzyme. Under optimum condition for gelatin extraction, the gelatin yield increased by nearly 50%, but the gel strength and viscosity were low. Faint presence of β-chain (α-chain dimmers) band was observed and α1 and α2 chains regions were revealed scarce. Lower molecular weight regions were also visible.
It is evident from the above examples that the functional characteristics of the gelatin extracted using proteases were lowered, although high gelatin yield was obtained. Less degraded gelatin proteins having relatively high-molecular-weight polypeptides have been shown to exhibit better functional qualities.[Citation16,Citation20–Citation22] This situation demands for some novel enzymes cleaving the long chains of collagen at very few sites so that long-chain gelatin possessing better quality characteristics could be produced.[Citation23] Additionally, actinidin enzyme derived from kiwi fruit was found to hydrolyze myofibril proteins of meat most effectively among enzymes actinidin, bromelain, papain, and zingibain.[Citation24] Published research articles on gelatin extraction using actinidin and zingibain enzymes are not available to the best of the authors’ knowledge. Therefore, in this study, a new enzyme actinidin and papain were used at their optimum temperature and pH to extract gelatin from bovine skin and analyze the extraction yield and characteristics of the extracted gelatin.
Materials and methods
Chemicals
N,N,Nʹ,Nʹ-tetramethyl ethylenediamine (TEMED), sodium dodecyl sulfate (SDS), acrylamide, 2-mercaptoethanol, and coomassie brilliant blue R-250 were acquired from Merck (Darmstadt, Germany). Kiwi fruit (Actinidia deliciosa)-derived actinidin enzyme (>30 casein units/g) was provided by Ingredient Resources Pty Ltd, Warriewood, NSW, Australia. Papain enzyme (EC 3.4.22.2 having activity ≥30,000 USP-U/mg) obtained from papaya (Carica papaya) was procured from Merck. Hydroxyproline standard was purchased from Agilent Technologies (CA, USA). For amino acid analysis, amino acid standards, chromatographic column, reagents, and mobile phase were procured from Waters Corporation (MA, USA). In all other cases, analytical grade reagents and chemicals were used.
Skin preparation
Neck region skin of female Brahma of age about 3–4 years was procured from Shah Alam Abattoir (Selangor, Malaysia) and kept under ice during transportation and stored at −20°C in the laboratory. The subcutaneous fat removal was done by scrapping, and thereafter, it was cleaned carefully. The skin was kept at −20°C until further use. Overnight thawing at 4°C was done before using it.
Gelatin extraction from skin using actinidin and papain enzyme-assisted process
Noncollagenous protein removal
Noncollagenous proteins were removed by treating the skin with 0.1 M NaOH (w/v) solution for 6 h maintained at 25 ± 1°C room temperature. The ratio of skin to solution was 1:5 (w/v), and the mixture was continuously stirred and the old solution was replaced at every 2-h interval with fresh solution. After this, skin hairs were removed by scraping and incised into a small size of approximately 1 cm × 2 cm. Distilled water was used to wash the skin thoroughly till neutral pH was achieved from the wash water.
Actinidin and papain enzyme-assisted gelatin extraction
HCl solution (1%) was used at a ratio of 10:1 (v/w) to soak the skin for 20 h at discontinuous stirring. After soaking, skin samples were washed using distilled water until wash water became neutral. The swollen skins were incubated for 48 h in enzyme solutions (1: 3 (w/v) skin to solution ratio) with actinidin and papain at the level of 0, 5, 10, 15, 20, and 25 unit/g of wet skin at their corresponding optimum temperature and pH (20°C and pH 7.5 for papain and 40°C and pH 6.0 for actinidin as per manufacturers’ specifications) for the maximum activities of the enzymes. To maintain the required temperatures, the mixture was put in the water bath under stirring. Upon completion of incubation time, the enzymatic action was destroyed by keeping the mixture in a water bath for 15 min at 90°C. Thereafter, gelatin was extracted in a water bath maintained at 60°C for 6 h with continuous stirring. The mixture so obtained was passed through cheesecloth and subsequently clarified by Beckman Coulter Avanti J-26 XPI centrifugation carried out for 20 min at 12,800 × g. Finally, gelatin was obtained by freeze-drying (Labconco FreeZone18, KS, USA) the resulting supernatant. Control gelatin was extracted without any enzymatic pretreatment from the HCl-treated skin as stated above. Triplicate determination was carried out for the gelatin extraction.
Yield
The gelatin yield was determined on the basis of the weight of the wet skin and the weight of the gelatin extracted out of it.[Citation14,Citation15,Citation25,Citation26]
Determination of pH
Gelatin pH was measured with Mettler Toledo, AG 8603 (Switzerland) pH meter. Distilled water (20 mL) was used to dissolve gelatin (0.2 g) to prepare 1% (w/v) gelatin solution and the solution temperature was brought down to room temperature (about 25ºC). Two buffer solutions of pH 4.0 and 7.0 were used to standardize the pH meter and determination was performed in triplicates.
Amino acid analysis
High-performance liquid chromatography (HPLC) from Waters Corporation (Milford, MA, USA) was used to analyze the amino acid profile of the recovered gelatins.[Citation27] 0.1 to 0.2 g of gelatin was hydrolyzed at 110°C for a duration of 24 h with 5 mL of 6 N HCl. After cooling, 4 mL of L–amino-N-butyric acid (AABA) used as internal standard was mixed to the hydrolysate, and thereafter, it was paper and syringe filtered. Twenty microlitres of ACCQ reagent was added to 70 µL borate buffer (Waters Corporation) and 10 µL of the filtered hydrolysate was added to it. Hydroxyproline (Agilent Technologies, CA, USA) was added to spike the internal standard and amino acid standard H mixture (Waters Corporation) was used to quantify all the AA excluding cysteine, tryptophan, and methionine. The peaks were separated with the aid of AccQ Tag 3.9 150 mm AA column (Waters Corporation). Triplicate determination was done, and the presented data represent the mean values. Standard deviations were observed to be less than 2% in all cases.
Electrophoretic analysis
SDS-PAGE (Sodium dodecyl sulphate polyacrylamide gel electrophoresis) was employed to establish the molecular weight distributions of the protein chains found in the extracted gelatins.[Citation28] Ten milligrams per milliliter solution of dry gelatin was prepared by dissolving 10 mg gelatin in 1 mL deionized water at 60°C. Loading buffer (fivefold-concentrated) having β-mercaptoethanol was mixed with the sample solution in a 2:1 (v/v) ratio. The mixture was kept in a water bath at 95°C for 5 min and loaded onto stacking gels (4%) and resolving gels (7.5%). The gels were run using Mini-PROTEAN Tetra System (Bio-Rad Laboratories, CA, USA) at 15 mA/gel (constant current) for 15 min followed by 25 mA/gel for the time duration the bromophenol blue dye touched the base of the gel. The gels were stained with 0.1% (w/v) solution of coomassie blue. The molecular weights of the gelatin polypeptides were estimated using prestained protein ladder (BLUeye, GeneDireX, Taiwan).
Turbidity
A slightly modified method of Cho et al.[Citation29] was followed to measure gelatin turbidity. Gelatin solution of concentration 0.5% (w/w) was prepared by adding 0.025 g gelatin in 5 mL distilled water at 60°C. The absorbance of the solution at 660 nm was measured using Shimadzu UV Spectrophotometer, Model UV-1800, Kyoto, Japan.
Determination of gel strength
Determination of the gel strength was done following the slightly modified method of Fernandez-Dıaz et al.[Citation30] Two grams of gelatin was weighed and transferred to 50 mL beaker (Schott Duran, Germany) and 30 mL of distilled water heated to 60°C was added to prepare a gelatin solution of 6.67% (w/v). The solution was stored at 7°C for 16–18 h for gel maturation. Cylindrical teflon plunger having a flat face (P/0.5R) measuring 1.27 cm in diameter was fitted to Texture Analyzer Model TA-XT2i (Stable Micro Systems, Surrey, UK) to measure the gel strength of gelatin samples. The sample diameter and height were 3.8 and 2.7 cm, respectively. The plunger speed was fixed at 0.5 mm/s, and the gel strength was recorded (in grams) when the probe entered 4 mm inside the sample. Means of three determinations were presented as the final value.
Determination of viscosity
Gelatin (1.34 g) was dissolved in distilled water (20 mL) to prepare a gelatin solution of 6.67% concentration and heated to 60°C. Viscosity was measured using RheolabQC viscometer purchased from Anton Paar, Graz, Austria. Triplicate determination was done to arrive at the mean.
Fourier-transform infrared (FTIR) spectroscopy
Spectrometer from Perkin Elmer Ltd., Model: Spectrum 100, AZ, USA was used to acquire the FTIR spectra of different gelatin samples. CsI internal reflection crystal and deuterated L-alanine triglycine sulfate (DLATGS) detector were used. Spectra were acquired in the mid-IR region of 4000–500 cm−1 at room temperature with the resolution of 4 cm−1. Automatic signals were collected using 16 scans. The signals were normalized using a background spectrum emitted from a clean and empty cell at 25°C.
Microstructure analysis of gelatin
Scanning electron microscopy (SEM) was used to elaborate the microstructures of gelatin samples with JEOL JSM-IT100 InTouchScope, Tokyo, Japan. Bronze stub was used to mount 2–3 mm-thick dried gelatin samples and sputter-coated with gold (BAL-TEC SCD 005 sputter coater, Schalksmühle, Germany). Samples were observed at 30⨰ at an accelerated voltage of 10 kV.
Statistical analysis
Statistical Analysis System (SAS) Version 9.4 software (Statistical Analysis System, SAS Institute Inc., Cary, NC, USA) was used to analyze all statistical data. Data were subjected to ANOVA using the GLM procedure where the different enzymes and their different levels were fixed as the main effects. Duncan’s multiple range test (P < 0.05) was used to determine differences among the treatment means.
Results and discussion
Yield
The stabilizing hydrogen bonds of collagen are destroyed by heating at a higher temperature resulting in the conversion of native helix to coil structure, which leads to the transformation of collagen to gelatin.[Citation31] Moreover, some peptide bonds are broken down.[Citation32] The effects of different levels (0, 5, 10, 15, 20, and 25 unit/g of skin) of actinidin and papain on gelatin yield are shown in . In both enzyme groups, the yield of gelatin was significantly (P < 0.05) increased with increasing level of enzyme. Nonetheless, using a level of 25 unit/g of skin led to a significant decrease in the yield of gelatin in both enzyme groups. Yield of gelatin at A20 (22.67%) was significantly higher (P < 0.05) than all other treatment levels of actinidin and control (17.90%). Except for P0, the yield of gelatin obtained from papain at P20 (23.59%) was significantly (P < 0.05) higher compared to the control and other papain levels. The results are supported by the previous findings of Balti et al.[Citation14] and Lassoued et al.[Citation15] who found higher gelatin yield with the proteolytic enzymes. Gelatin yield of 7.84% on wet weight basis was obtained from cuttlefish (Sepia officinalis) skin using crude acid protease extracted from smooth hound at level 15 unit/g.[Citation14] They reported increased gelatin yield as enzyme level was increased. Treating thornback ray skin with pepsin yielded 30% gelatin.[Citation15] Nalinanon et al.[Citation12] also reported similar findings. They obtained noticeably enhanced gelatin yield with proteases compared to when the proteases were not used.
Table 1. Yield and pH of the bovine skin gelatin extracted using enzymes actinidin and papain at different levels. Values are given as mean±SE from triplicate determination
pH
There were significant (P < 0.05) differences in pH between the control (2.16) and the treatments of GEA and GEP groups (). No significant differences were found among GEA samples. Low pH as observed for the samples might be attributed to the acidic agent (HCl) used for skin pretreatment. Bovine gelatin pH was found to be 5.48.[Citation1] There are lack of studies on the effect of gelatin processing method on the pH of extracted gelatin.[Citation33]
Amino acid analysis of gelatin
Primarily, various gelatin characteristics are controlled by the molecular weight distribution and composition of amino acid.[Citation34] Glycine is the most abundant amino acid in gelatin.[Citation35] According to Asghar and Henrickson[Citation10], 50–60% of α chains comprise triple peptides having the repeating chains of Gly-X-Y, where X and Y mostly represent proline and hydroxyproline, respectively. High imino acid (proline and hydroxyproline) content, especially hydroxyproline, was reported to be found in gelatin obtained from mammalian animals.[Citation36]
In the present study, GEA showed that the glycine, proline, and hydroxyproline values varied from 21.00% to 15.97%, 11.08% to 8.31% and 15.17% to 10.93%, respectively, whereas the corresponding values for GEP were 21.74% to 19.16%, 10.96% to 9.79%, and 14.82% to 12.40%, respectively (). Glycine (Gly), proline (Pro), and hydroxyproline (Hyp) content of commercial gelatin obtained from calf skin and ox hide and calf skin gelatin were 26.9%, 14.0%, and 14.6% and 27.6%, 16.5%, and 13.4%, respectively.[Citation37] Furthermore, Balti et al.[Citation14] and Lassoued et al.[Citation15] reported glycine to be 34.1% and 34.48%, proline to be 12.3% and 13.39%, and hydroxyproline to be 9.6% and 9.54%, respectively, of the total amino acids in edible halal bovine gelatin. Amino acid contents in the present study were expressed as mg of amino acid per 100 mg of sample (percentage of sample weight). This gave rise to differences between this study and earlier reported studies with regard to the content of different amino acids. Additionally, the differences in the amino acid content might arise due to variation in the skin source and gelatin manufacturing process.[Citation38]
Table 2. Amino acid composition (%) of control gelatin, gelatin extracted using actinidin, and papain enzymes from the bovine skin
The imino acid (Pro+Hyp) content in GEA and GEP varied from 26.25% to 19.25% and 26.34% to 21.27%, respectively. The bovine gelatin contained 21.90%[Citation14,Citation15] or 23.3%[Citation39] imino acid. Hyp content in GEA and GEP (varied from 15.17% to 10.93% and 14.96% to 12.40%, respectively) was higher than the Hyp content (9.6% and 9.54%, respectively) in food grade bovine gelatin as reported by Lassoued et al.[Citation15] and Balti et al.[Citation14] The stability of the triple-helical structure of the renatured gelatins is directly dependent on the amount of imino acids as nucleation zones are believed to be formed in the area high in Pro + Hyp content.[Citation40] Specifically, Hyp is thought to provide strength to the triple-helical structure of collagen by forming hydrogen bonds between the different strands through its hydroxyl group.[Citation40–Citation42] The viscoelastic properties of gelatin are not only dependent on amino acid composition[Citation43] but also on the molecular weight distribution. The high gel strength and viscosity of the GEA compared to GEP might be due to the presence of high-molecular-weight polypeptides in GEA.
SDS-PAGE analysis of gelatin
The functional characteristics of gelatin are affected by the molecular weight distribution, subunit structure and its composition, as well as the composition of amino acids.[Citation14] Pretreated skin (PS) samples, GEA and GEP samples were subjected to SDS-PAGE analysis (). The molecular distribution pattern of PS samples revealed α1 and α2 chains along with one covalently bound α-chain dimers known as β chain. High-intensity bands at the top of the gels were observed in both types of gelatin samples including the PS sample which were very high-molecular-weight components produced as a result of residual heat-stable crosslinks.[Citation14,Citation44] All GEA samples showed complete degradation of β chains and presence of α1 and α2 chains (slightly degraded) and lower molecular weight peptides (due to degradation of β chains), whereas GEP samples revealed a complete breakdown of β and α chains leading to the formation of low-molecular-weight peptides. Peptides starting from around less than 100 and 60 kDa were observed in the obtained GEA and GEP, respectively. Mohtar et al.[Citation1] reportedly observed protein bands in commercial bovine gelatin corresponding to 100 and 40 kDa. Intensities of the bands were clearly more in GEA than GEP. Smear band noticed more in case of GEP indicated overhydrolysis by papain.
Figure 1. SDS-PAGE pattern of pretreated skin (PS) sample along with gelatin samples extracted using different levels of enzyme actinidin (A) and papain (P). A0/P0, A5/P5, A10, A15/P15, A20/P20, and A25/P25 refer to corresponding actinidin/papain enzyme level of 0, 5, 10, 15, 20, and 25 unit/g of skin, respectively. M: high-molecular-weight marker. C: control gelatin
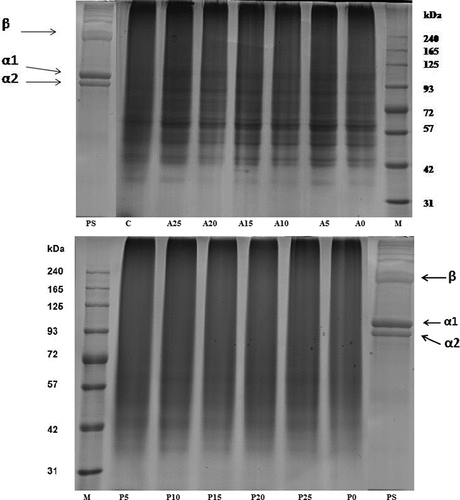
Gelatin samples extracted from rawhide with the aid of crude protease derived from papaya latex and commercial papain exhibited only low-molecular-weight protein polypeptides, indicating that α and β chains of the recovered gelatin were entirely degraded.[Citation17] β-chain band was faintly observed and α1 and α2-chains regions were scarcely observed for fish gelatin extracted from surimi processing waste extracted using bromelain enzyme.[Citation19] The formation of short-chain peptides in the present case was in agreement with the findings of Nalinanon et al.[Citation12] who found a complete absence of α and β chains in the gelatin extracted by pretreating the bigeye snapper (Priacanthus tayenus) skin with bigeye snapper pepsin. Similarly, a complete absence of β components was found in gelatin samples extracted from bigeye snapper (Priacanthus macraacanthus) skin at a temperature higher than 40°C and polypeptide protein chains further underwent degradation along with α1 and α2-chains at a temperature higher than 45°C.[Citation45]
Turbidity
Montero et al.[Citation46] posited that the presence of more aggregates in gelatin of high- molecular weight resulted in high turbidity. High turbidity values for GEA compared to GEP could be attributed to α1 and very slightly degraded α2 chains present in all GEA samples (). All GEP revealed complete degradation of β and α chains, resulting in failure to form more aggregates of high molecular weight. There was a significant increase (P < 0.05) in the turbidity of the GEA samples as the level of enzymes increased. This might be due to the imparting of turbidity by the enzyme.
Table 3. Turbidity, gel strength, and viscosity of the bovine skin gelatin extracted using enzymes actinidin and papain at different levels. Values are given as mean±SE from triplicate determination
Gel strength
Gel strength is the most important functional characteristics of gelatin, and it is influenced by the intricate functional interactions between the composition of amino acid and amount of β chains and α chain ratio.[Citation14] The gelling strength of industrial gelatins varies from 100 to 300 g, and gelatins having gel strength values of 250–260 g are mostly preferred.[Citation47] Generally, gelatins having low-molecular-weight distribution show low gel strength than the high-molecular-weight gelatin[Citation20] because peptides of low molecular weight might be unable to form interjunction zones efficiently, jeopardizing the aggregation of gelatin chains resulting in low gelling property. The gelatin gelling properties were also affected by the imino acid, proline, and hydroxyproline[Citation48] as hydrogen bonds are formed between the different strands of gelatin by imino acid resulting in highly stable gel possessing high gel strength.
The gel strength values of all the GEA samples were high (). The gel strength of gelatin extracted using actinidin enzyme decreased significantly (P < 0.05) as the level of the enzymes increased. The gel strength for A20 was 366.39 g and the least recorded gel strength was for A25 (348.56 g). All the bloom values were significantly higher (P < 0.05) than the control (283.35 g). The gel strength of the commercial bovine gelatins as reported by Lassoued et al.[Citation15], Mohtar et al.,[Citation1] and Ahmad and Benjakul[Citation32] were 259.65, 273, and 293.22 g, respectively. The higher bloom values for extracted gelatin using actinidin could be explained in terms of formation of peptides of uniform length, leading to better physical crosslinking of the gelatin resulting in higher gel strength.[Citation18] The presence of peptide chains α1 and slightly degraded α2 chains of high molecular weight in the GEA samples might be held responsible for such high values of gel strength as these chains enable the formation of triple-helical structure during maturation process (cooling) and lead to helix growth.[Citation14]
Meanwhile, papain-treated samples showed low gel strength (). The highest gel strength of 119.34 g was recorded for P10. Damrongsakkul et al.[Citation18] and Pitpreecha and Damrongsakkul[Citation17] reported low gel strength gelatins obtained from rawhide splits and rawhide using papain and crude and commercial papain, respectively. The enzyme cleaved the peptide bonds more severely than by acid and lime process.[Citation49] Low strength gelatin resulted from the enzymatic hydrolysis of the collagen by crude protease enzyme from papaya latex compared to typical hydrolysis by acid and lime.[Citation17] Papain pretreatment caused severe cleavage of collagen chains due to overhydrolysis leading to the formation of much shorter chains,[Citation19] resulting in the lower gel strength of GEP in comparison to GEA. The shorter chain peptides had low gel-forming ability resulting in a weaker gel network.[Citation12] Despite having imino acid-rich composition, the squid gelatin had very low gel strength (less than 25 g) (Norziah et al., 2014) due to the presence of protein degradation fragments.[Citation21] Generally, gelatins consisting of low-molecular-weight distribution peptides show low gelling properties than gelatins possessing high-molecular-weight polymers[Citation20,Citation50] because smaller polypeptide gelatin chains fail to create the junction zone resulting in failure to establish strong chain arrangement which reflected as low gel strength.[Citation51]
Viscosity
Commercially, the second most significant functional property of gelatin is viscosity.[Citation37] Cleavage of hydrogen and possibly electrostatic bonds causes collagen to denature in hot water, and this degrades the triple-helical (three α chains) structure of native collage generating gelatin containing random polypeptides consisting of one, two , or three chains that give high viscosity when dissolved in water.[Citation20] Molecular weight and polydispersity of the gelatin polypeptides partially control viscosity,[Citation52] indicating that viscosity would increase if higher molecular weight peptides are present but based on the molecular weight distribution, polydispersity can have a variable effect.[Citation53]
Viscosity was 12.10 mPa.s for the control, which was significantly lower (P < 0.05) than GEA samples but was significantly higher than GEP (P < 0.05) (). The highest viscosities were exhibited by A20 and A25 samples (13.53 and 13.97 mPa.s, respectively), whereas P25 showed the highest viscosity of 10.70 mPa.s. Viscosity of the commercial bovine gelatin was reported to be 9.80 cP.[Citation1] Viscosities of pepsin and proctase added gelatin extracted from bovine hide were 3.43 and 1.11 cP, respectively.[Citation13] Gelatin obtained from rawhide by papain hydrolysis at 70°C after 90 min extraction at pH 6, 7, and 8 exhibited the viscosities of 3.5, 4.5, and 5.6 Pa.s−1, respectively.[Citation18]
FTIR spectra
Gelatin samples A20 and P20 were selected for FTIR study based on comparatively better yield, gel strength, and viscosity. Usually, FTIR spectroscopy is employed to elaborate the functional groups and secondary structure of gelatins, and the amide I band detected between 1600 and 1700 cm−1 is the most crucial for secondary structure study of the protein.[Citation54] Amide I shows C = O stretching vibration hydrogen bonding coupled with COO, coupled to contributions from the CN stretch, CCN deformation, and in-plane NH bending mode.[Citation55] FTIR spectra of control, A20, and P20 have been depicted in . Major peaks were observed to fall in the amide regions but exhibited minor variations in the spectra but were concomitant with earlier results of Muyonga et al.[Citation54] It was observed that control, A20, and P20 displayed their amide I bands at the wave numbers of 1631.78, 1633.71, and 1629.85 cm−1, respectively. Detection of absorption peak at 1633 cm−1 shows a coiled structure of gelatin[Citation56] indicating towards the maintained triple helical strand arrangement of A20 gelatin due to little deviation from the characteristics amide A band wavenumber compared to P20 and control. The amide I peak for P20 was noticed at lowest wavenumber (greater deviation from the characteristic amide I wavenumber) followed by control, which suggested a greater loss of molecular order in P20 gelatin sample.
Figure 2. Fourier-transform infrared spectra of control (C), GEA, and GEP gelatin samples extracted at the level of 20 units of enzyme/g of wet skin
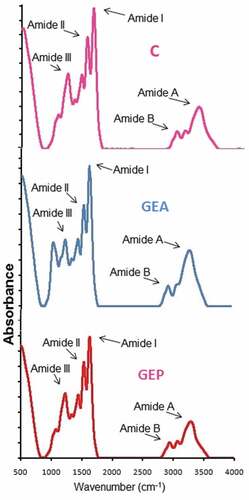
An out-of-phase combination of CN stretch and in-plane NH deformation modes of the peptide group are mainly reflected by amide II vibration modes.[Citation32,Citation57] Dry collagen exhibited its characteristic amide II absorption spectra in between the range of 1530 and 1540 cm−1.[Citation58] The specific absorption bands of control, A20, and P20 gelatin in amide II region were displayed at the wavenumbers of 1537.27, 1539.2, and 1546.91 cm−1, respectively. The lower wavenumber of control and A20 than P20 indicated higher NH group engagement in hydrogen bond formation.[Citation59]
Absorption spectra between 1200 and 1400 cm−1 shows amide III band resulting from wagging vibrations of CH2 groups from the proline side chains and glycine backbone and C-N stretching and N-H in-plane bending resulting from amide linkages.[Citation60] Amide III was detected around the wavenumbers of 1234.44, 1234.44, and 1240.23 cm−1 for control, A20, and P20, respectively. The relatively higher wavenumber for P20 indicated higher disruption than A20 in the α helical structure of the native state to unsystematic coiled arrangement related with the lost triple-helical strand structure during collagen transformation into gelatin.[Citation54] Additionally, some more peaks for control were detected at wavenumber of 1029.99, 1078.21, 1336.67, and 1448.54 cm−1 for A20 at 858.323, 921.974, 1026.13, 1153.43, 1336.67, and 1448.54 cm−1 and for P20 at 528.5, 1033.85, 1080.14, 1338.6, and 1452.4 cm−1. C ̶ O stretching vibrations of the short peptide chains gave rise to these bands.[Citation60]
Amide A band, displayed around wavenumber 3400–3440 cm−1, results from NH-stretching coupled with hydrogen,[Citation54] and it is moved to lower wavenumbers, generally close to 3300 cm−1, due to H bonding by the N-H group of the peptide chain.[Citation58] The amide A of the triple-helix polymer is shifted to lower wavenumber due to the formation of H bond by the N-H group of a peptide.[Citation44,Citation61] Amide A appeared at 3294.42, 3311.78, and 3305.99 cm−1 for control, A20, and P20 gelatins, respectively. The lower wavenumber of control and P20 compared to A20 showed that the N-H group in α chains was more engaged in hydrogen bonding due to a higher degradation of protein chains in these two samples. Amide B bands show asymmetric stretching vibration of = C-H and NH3+.[Citation32] The amide B for control, A20, and P20 was observed at 2931.80, 2953.02, and 2939.52 cm−1, respectively. The lower wavenumbers of amide B band for control and P20 compared to A20 implied the interaction of -NH3 group between peptide chains in those gelatin samples.[Citation44,Citation59] Therefore, it can be summarized that the enzyme pretreatment affected the secondary structures and functional groups of the extracted gelatin.
Microstructure of gelatin
Based on comparatively better yield and functional properties, the microstructure of A20 and P20 along with control was analyzed. The microstructures of control, A20, and P20 gelatin samples as revealed by SEM are illustrated in . Gelatin microstructures reflect the physical characteristics of the gelatin. The severity of pores and irregularity were more evident in the P20 sample compared to control and A20 samples. A20 sample demonstrated interconnected bigger particle size and denser structure with least number of voids compared to the other two samples, and this observation was reflected in the higher gel strength () of A20 sample. The result was similar to Tu et al.[Citation58] and Wangtueai et al.[Citation62] who reported that higher gel strength gelatin had finer and denser gel network. P20 structure was disorganized, and control gelatin had honeycomb sheet structure. The result revealed that actinidin enzyme caused the least damage to gelatin polypeptides chains, and papain enzyme led to more severe degradation of the gelatin protein chains.
Conclusions
GEA samples particularly at A20 showed better yield, gelatin strength, and viscosity and revealed the presence of α-chains and low-molecular-weight peptides. In contrast, GEP samples showed low gel strength and comparatively less viscosity because of the presence of low-molecular-weight peptides due to highly degraded gelatin chains. The result indicated that actinidin enzyme, particularly at the level of 20 unit/g of wet skin, could be used to enhance the gelatin extraction yield and functional qualities from bovine skin.
Acknowledements
The authors extend their gratefulness to Universiti Putra Malaysia for providing the Putra Grant (UPM/700-2/1/GP-IPS/2015/9467000) to carry out the research works. The first author is indebted to ICAR, New Delhi, for providing ICAR International Fellowship and permission to pursue PhD in Universiti Putra Malaysia.
Additional information
Funding
References
- Mohtar, N. F.; Perera, C.; Quek, S.-Y. Optimisation of Gelatine Extraction from Hoki (Macruronus Novaezelandiae) Skins and Measurement of Gel Strength and SDS–PAGE. Food Chem. 2010, 122(1), 307–313. DOI: 10.1016/j.foodchem.2010.02.027.
- Giménez, B.; Gómez-Guillén, M.; Montero, P. The Role of Salt Washing of Fish Skins in Chemical and Rheological Properties of Gelatin Extracted. Food Hydrocolloids. 2005, 19(6), 951–957. DOI: 10.1016/j.foodhyd.2004.09.012.
- Gómez-Guillén, M. C.; Giménez, B.; López-Caballero, M. E.; Montero, M. P. Functional and Bioactive Properties of Collagen and Gelatin from Alternative Sources, A Review. Food Hydrocolloids. 2011, 25(8), 1813–1827. DOI: 10.1016/j.foodhyd.2011.02.007.
- Bailey, A. J.; Light, N. D. Connective Tissue in Meat and Meat Products: Elsevier Applied Science: London, 1989.
- Johnston-Banks, F. A. Gelatine. In Food Gels; Harris, P., Ed.; Elsevier Applied Sciences Publishers: New York, 1990; pp 233–289.
- Te Nijenhuis, K. Thermoreversible Networks: Viscoelastic Properties and Structure of Gels. In Advance Polymer Science; Springer: Berlin, Germany, 1997; pp 1–264.
- Stainsby, G. Gelatin Gels. In Collagen as a Food Advances in Meat Research; Pearson, A. M., Dutson, T. R., Bailey, A. J., Eds.; Van Nostrand Reinhold Company, Inc.: New York, 1987; Vol. 4, pp 209–222.
- Djabourov, M.; Lechaire, J.-P.; Gaill, F. Structure and Rheology of Gelatin and Collagen Gels. Biorheology. 1993, 30(3–4), 191–205.
- Bailey, A. Round Table Session 1–Structure of Collagen. Adv. Meat Res. 1985, 4, 131–143.
- Asghar, A.; Henrickson, R. L. Chemical, Biochemical, Functional, and Nutritional Characteristics of Collagen in Food Systems. In Advances in Food Research; Chischester, C. O., Mark, E. M., Stewart, G. F., Eds.; Academic Press: London, 1982; Vol. 28, pp 231–372.
- Galea, C. A.; Dalrymple, B. P.; Kuypers, R.; Blakeley, R. Modification of the Substrate Specificity of Porcine Pepsin for the Enzymatic Production of Bovine Hide Gelatin. Protein Sci. 2000, 9(10), 1947–1959. DOI: 10.1110/ps.9.10.1947.
- Nalinanon, S.; Benjakul, S.; Visessanguan, W.; Kishimura, H. Improvement of Gelatin Extraction from Bigeye Snapper Skin Using Pepsin-Aided Process in Combination with Protease Inhibitor. Food Hydrocolloids. 2008, 22(4), 615–622. DOI: 10.1016/j.foodhyd.2007.01.012.
- Chomarat, N.; Robert, L.; Seris, J. L.; Kern, P. Comparative Efficiency of Pepsin and Proctase for the Preparation of Bovine Skin Gelatin. Enzyme Microb. Technol. 1994, 16(9), 756–760. DOI: 10.1016/0141-0229(94)90032-9.
- Balti, R.; Jridi, M.; Sila, A.; Souissi, N.; Nedjar-Arroume, N.; Guillochon, D. Extraction and Functional Properties of Gelatin from the Skin of Cuttlefish (Sepia Officinalis) Using Smooth Hound Crude Acid Protease-Aided Process. Food Hydrocolloids. 2011, 25(5), 943–950. DOI: 10.1016/j.foodhyd.2010.09.005.
- Lassoued, I.; Jridi, M.; Nasri, R.; Dammak, A.; Hajji, M.; Nasri, M.; Barkia, A. Characteristics and Functional Properties of Gelatin from Thornback Ray Skin Obtained by Pepsin-Aided Process in Comparison with Commercial Halal Bovine Gelatin. Food Hydrocolloids. 2014, 41, 309–318. DOI: 10.1016/j.foodhyd.2014.04.029.
- Zhang, F.; Xu, S.; Wang, Z. Pre-Treatment Optimization and Properties of Gelatin from Freshwater Fish Scales. Food Bioprod. Process. 2011, 89(3), 185–193. DOI: 10.1016/j.fbp.2010.05.003.
- Pitpreecha, S.; Damrongsakkul, S. Hydrolysis of Raw Hide Using Proteolytic Enzyme Extracted from Papaya Latex. Korean J. Chem. Eng. 2006, 23(6), 972–976. DOI: 10.1007/s11814-006-0017-z.
- Damrongsakkul, S.; Ratanathammapan, K.; Komolpis, K.; Tanthapanichakoon, W. Enzymatic Hydrolysis of Rawhide Using Papain and Neutrase. J. Ind. Eng. Chem. 2008, 14(2), 202–206. DOI: 10.1016/j.jiec.2007.09.010.
- Norziah, M. H.; Kee, H. Y.; Norita, M. Response Surface Optimization of Bromelain-Assisted Gelatin Extraction from Surimi Processing Wastes. Food Biosci. 2014, 5, 9–18. DOI: 10.1016/j.fbio.2013.10.001.
- Badii, F.; Howell, N. K. Fish Gelatin: Structure, Gelling Properties and Interaction with Egg Albumen Proteins. Food Hydrocolloids. 2006, 20(5), 630–640. DOI: 10.1016/j.foodhyd.2005.06.006.
- Gómez-Guillén, M. C.; Turnay, J.; Fernández-Dıaz, M. D.; Ulmo, N.; Lizarbe, M. A.; Montero, P. Structural and Physical Properties of Gelatin Extracted from Different Marine Species: A Comparative Study. Food Hydrocolloids. 2002, 16(1), 25–34. DOI: 10.1016/S0268-005X(01)00035-2.
- Muyonga, J. H.; Cole, C. G. B.; Duodu, K. G. Extraction and Physico-Chemical Characterisation of Nile Perch (Lates Niloticus) Skin and Bone Gelatin. Food Hydrocolloids. 2004, 18(4), 581–592. DOI: 10.1016/j.foodhyd.2003.08.009.
- Ahmad, T.; Ismail, A.; Ahmad, S. A.; Khalil, K. A.; Kumar, Y.; Adeyemi, K. D.; Sazili, A. Q. Recent Advances on the Role of Process Variables Affecting Gelatin Yield and Characteristics with Special Reference to Enzymatic Extraction: A Review. Food Hydrocolloids. 2017, 63, 85–96. DOI: 10.1016/j.foodhyd.2016.08.007.
- Ha, M.; Bekhit, A. E.-D. A.; Carne, A.; Hopkins, D. L. Characterisation of Commercial Papain, Bromelain, Actinidin and Zingibain Protease Preparations and Their Activities toward Meat Proteins. Food Chem. 2012, 134(1), 95–105. DOI: 10.1016/j.foodchem.2012.02.071.
- Bougatef, A.; Balti, R.; Sila, A.; Nasri, R.; Graiaa, G.; Nasri, M. Recovery and Physicochemical Properties of Smooth Hound (Mustelus Mustelus) Skin Gelatin. LWT Food Sci. Technol. 2012, 48(2), 248–254. DOI: 10.1016/j.lwt.2012.03.021.
- Ktari, N.; Bkhairia, I.; Jridi, M.; Hamza, I.; Riadh, B. S.; Nasri, M. Digestive Acid Protease from Zebra Blenny (Salaria Basilisca): Characteristics and Application in Gelatin Extraction. Food Res. Int. 2014, 57, 218–224. DOI: 10.1016/j.foodres.2014.01.041.
- Awad, E. A.; Zulkifli, I.; Farjam, A. S.; Chwen, L. T. Amino Acids Fortification of Low-Protein Diet for Broilers under Tropical Climate. 2. Nonessential Amino Acids and Increasing Essential Amino Acids. Ital. J. Anim. Sci. 2014, 13(3), 631–636.
- Laemmli, U. K. Cleavage of Structural Proteins during the Assembly of the Head of Bacteriophage T4. Nature. 1970, 227(5259), 680–685.
- Cho, S. M.; Kwak, K. S.; Park, D. C.; Gu, Y. S.; Ji, C. I.; Jang, D. H.; Lee, Y. B.; Kim, S. B. Processing Optimization and Functional Properties of Gelatin from Shark (Isurus Oxyrinchus) Cartilage. Food Hydrocolloids. 2004, 18(4), 573–579. DOI: 10.1016/j.foodhyd.2003.10.001.
- Fernandez-Dıaz, M. D.; Montero, P.; Gomez-Guillen, M. C. Gel Properties of Collagens from Skins of Cod (Gadus Morhua) and Hake (Merluccius Merluccius) and Their Modification by the Coenhancers Magnesium Sulphate, Glycerol and Transglutaminase. Food Chem. 2001, 74(2), 161–167. DOI: 10.1016/S0308-8146(01)00110-8.
- Benjakul, S.; Oungbho, K.; Visessanguan, W.; Thiansilakul, Y.; Roytrakul, S. Characteristics of Gelatin from the Skins of Bigeye Snapper, Priacanthus Tayenus and Priacanthus Macracanthus. Food Chem. 2009, 116(2), 445–451. DOI: 10.1016/j.foodchem.2009.02.063.
- Ahmad, M.; Benjakul, S. Characteristics of Gelatin from the Skin of Unicorn Leatherjacket (Aluterus Monoceros) as Influenced by Acid Pretreatment and Extraction Time. Food Hydrocolloids. 2011, 25(3), 381–388. DOI: 10.1016/j.foodhyd.2010.07.004.
- Park, J.-H.; Choe, J.-H.; Kim, H.-W.; Hwang, K.-E.; Song, D.-H.; Yeo, E.-J.; Kim, H. Y.; Choi, Y. S.; Lee, S. H.; Kim, C. J. Effects of Various Extraction Methods on Quality Characteristics of Duck Feet Gelatin. Korean J. Food Sci. Animal Resour. 2013, 33(2), 162–169. DOI: 10.5851/kosfa.2013.33.2.162.
- Giménez, B.; Gómez-Estaca, J.; Alemán, A.; Gómez-Guillén, M. C.; Montero, M. P. Physico-Chemical and Film Forming Properties of Giant Squid (Dosidicus Gigas) Gelatin. Food Hydrocolloids. 2009, 23(3), 585–592. DOI: 10.1016/j.foodhyd.2008.07.003.
- Arnesen, J. A.; Gildberg, A. Preparation and Characterisation of Gelatine from the Skin of Harp Seal (Phoca Groendlandica). Bioresour. Technol. 2002, 82(2), 191–194.
- Norland, R. E. Fish Gelatin. In Advances in Fisheries Technology and Biotechnology for Increased Profitability; Voight, M. N., Botta, J. K., Eds.; Technomic Publishing Co.: Lancaster: PA, 1990; pp 325–333.
- Ward, A. G.; Courts, A. Science and Technology of Gelatin; Academic Press: London, 1977.
- Zhou, P.; Regenstein, J. M. Determination of Total Protein Content in Gelatin Solutions with the Lowry or Biuret Assay. J. Food Sci. 2006, 71(8), C474–C9. DOI: 10.1111/j.1750-3841.2006.00151.x.
- Kasankala, L. M.; Xue, Y.; Weilong, Y.; Hong, S. D.; He, Q. Optimization of Gelatine Extraction from Grass Carp (Catenopharyngodon Idella) Fish Skin by Response Surface Methodology. Bioresour. Technol. 2007, 98(17), 3338–3343. DOI: 10.1016/j.biortech.2006.03.019.
- Ledward, D. A. Gelation of Gelatin. In Functional Properties of Food Macromolecules; Mitchell, J. R., Ledward, D. A., Eds.; Elsevier Applied Science Publishers: London, 1986; pp 171–201.
- Burjanadze, T. V. Hydroxyproline Content and Location in Relation to Collagen Thermal Stability. Biopolymers. 1979, 18(4), 931–938. DOI: 10.1002/bip.1979.360180413.
- Mizuno, K.; Hayashi, T.; Bächinger, H. P. Hydroxylation-Induced Stabilization of the Collagen Triple helixFurther Characterization of Peptides with 4 (R)-Hydroxyproline in the Xaa Position. J. Biol. Chem. 2003, 278(34), 32373–32379. DOI: 10.1074/jbc.M304741200.
- Giménez, B.; Turnay, J.; Lizarbe, M. A.; Montero, P.; Gómez-Guillén, M. C. Use of Lactic Acid for Extraction of Fish Skin Gelatin. Food Hydrocolloids. 2005, 19(6), 941–950. DOI: 10.1016/j.foodhyd.2004.09.011.
- Nagarajan, M.; Benjakul, S.; Prodpran, T.; Songtipya, P.; Kishimura, H. Characteristics and Functional Properties of Gelatin from Splendid Squid (Loligo Formosana) Skin as Affected by Extraction Temperatures. Food Hydrocolloids. 2012, 29(2), 389–397. DOI: 10.1016/j.foodhyd.2012.04.001.
- Intarasirisawat, R.; Benjakul, S.; Visessanguan, W.; Prodpran, T.; Tanaka, M.; Howell, N. K. Autolysis Study of Bigeye Snapper (Priacanthus Macracanthus) Skin and Its Effect on Gelatin. Food Hydrocolloids. 2007, 21(4), 537–544. DOI: 10.1016/j.foodhyd.2006.05.012.
- Montero, P.; Fernández-Dı́az, M. D.; Gómez-Guillén, M. C. Characterization of Gelatin Gels Induced by High Pressure. Food Hydrocolloids. 2002, 16(3), 197–205. DOI: 10.1016/S0268-005X(01)00083-2.
- Holzer, D.; Gelatin Production. US patent 5,484,888. 1996.
- Jongjareonrak, A.; Benjakul, S.; Visessanguan, W.; Prodpran, T.; Tanaka, M. Characterization of Edible Films from Skin Gelatin of Brownstripe Red Snapper and Bigeye Snapper. Food Hydrocolloids. 2006, 20(4), 492–501. DOI: 10.1016/j.foodhyd.2005.04.007.
- Simeonova, L.; Dalev, P. Utilization of a Leather Industry Waste. Waste Manag. 1996, 16(8), 765–769. DOI: 10.1016/S0956-053X(97)00020-2.
- Jridi, M.; Nasri, R.; Lassoued, I.; Souissi, N.; Mbarek, A.; Barkia, A.; Nasri, M. Chemical and Biophysical Properties of Gelatins Extracted from Alkali-Pretreated Skin of Cuttlefish (Sepia Officinalis) Using Pepsin. Food Res. Int. 2013, 54(2), 1680–1687. DOI: 10.1016/j.foodres.2013.09.026.
- Kittiphattanabawon, P.; Benjakul, S.; Visessanguan, W.; Shahidi, F. Comparative Study on Characteristics of Gelatin from the Skins of Brownbanded Bamboo Shark and Blacktip Shark as Affected by Extraction Conditions. Food Hydrocolloids. 2010, 24(2), 164–171. DOI: 10.1016/j.foodhyd.2009.09.001.
- Jamilah, B.; Tan, K. W.; Umi Hartina, M. R.; Azizah, A. Gelatins from Three Cultured Freshwater Fish Skins Obtained by Liming Process. Food Hydrocolloids. 2011, 25(5), 1256–1260. DOI: 10.1016/j.foodhyd.2010.11.023.
- Gudmundsson, M.; Hafsteinsson, H. Gelatin from Cod Skins as Affected by Chemical Treatments. J. Food Sci. 1997, 62(1), 37–39. DOI: 10.1111/jfds.1997.62.issue-1.
- Muyonga, J. H.; Cole, C. G. B.; Duodu, K. G. Fourier Transform Infrared (FTIR) Spectroscopic Study of Acid Soluble Collagen and Gelatin from Skins and Bones of Young and Adult Nile Perch (Lates Niloticus). Food Chem. 2004, 86(3), 325–332. DOI: 10.1016/j.foodchem.2003.09.038.
- Bandekar, J. Amide Modes and Protein Conformation. Biochim. Biophys. Acta, Protein Struct. Mol. Enzymol. 1992, 1120(2), 123–143. DOI: 10.1016/0167-4838(92)90261-B.
- Yakimets, I.; Wellner, N.; Smith, A. C.; Wilson, R. H.; Farhat, I.; Mitchell, J. Mechanical Properties with respect to Water Content of Gelatin Films in Glassy State. Polymer. 2005, 46(26), 12577–12585. DOI: 10.1016/j.polymer.2005.10.090.
- Lavialle, F.; Adams, R. G.; Levin, I. W. Infrared Spectroscopic Study of the Secondary Structure of Melittin in Water, 2-Chloroethanol, and Phospholipid Bilayer Dispersions. Biochemistry. 1982, 21(10), 2305–2312.
- Tu, Z.-C.; Huang, T.; Wang, H.; Sha, X.-M.; Shi, Y.; Huang, X.-Q.; Man, Z. Z.; Li, D. J. Physico-Chemical Properties of Gelatin from Bighead Carp (Hypophthalmichthys Nobilis) Scales by Ultrasound-Assisted Extraction. J. Food Sci. Technol. 2015, 52(4), 2166. DOI: 10.1007/s13197-013-1239-9.
- Ahmad, M.; Benjakul, S.; Ovissipour, M.; Prodpran, T. Indigenous Proteases in the Skin of Unicorn Leatherjacket (Alutherus Monoceros) and Their Influence on Characteristic and Functional Properties of Gelatin. Food Chem. 2011, 127(2), 508–515. DOI: 10.1016/j.foodchem.2011.01.032.
- Jackson, M.; Watson, P. H.; Halliday, W. C.; Mantsch, H. H. Beware of Connective Tissue Proteins: Assignment and Implications of Collagen Absorptions in Infrared Spectra of Human Tissues. Biochim. Biophys. Acta-Mol. Basis Dis. 1995, 1270(1), 1–6. DOI: 10.1016/0925-4439(94)00056-V.
- Doyle, B. B.; Bendit, E. G.; Blout, E. R. Infrared Spectroscopy of Collagen and Collagen‐Like Polypeptides. Biopolymers. 1975, 14(5), 937–957. DOI: 10.1002/bip.1975.360140505.
- Wangtueai, S.; Noomhorm, A.; Regenstein, J. M. Effect of Microbial Transglutaminase on Gel Properties and Film Characteristics of Gelatin from Lizardfish (Saurida Spp.) Scales. J. Food Sci. 2010, 75(9), C731–C739. DOI: 10.1111/j.1750-3841.2010.01835.x.