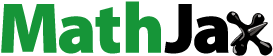
ABSTRACT
Impact of high-speed jet (HSJ) treatment on morphology, crystalline structure, retrogradation, thermal and rheological properties of Arenga pinnata (Wurmb.) Merr starch (APS) was investigated. Decreasing with the number of HSJ treatment passes were the turbidity values of pastes (long-term retrogradation), the gelatinization enthalpy, and the crystallinity. Scanning electron microscopy showed APS granule structure was degraded into tiny round particle and thin sheet structures. X-ray diffraction illustrated that the C-type X-ray pattern was changed toward a weak A-type X-ray pattern. With further increases in the number of passes, the weak A-type pattern was changed to amorphous pattern. Dynamic oscillation analysis revealed that HSJ treatment significantly altered the viscoelasticity of APS. During a dynamic frequency sweep, G′ values and G″ at 10 Hz decreased from 589.9 Pa (HSJ0) to 194.9 Pa (HSJ8) and from 101.6 Pa (HSJ0) to 24.5 Pa (HSJ8), respectively, implying that HSJ treatment had a great influence on the elasticity of the APS gel. The results indicated that degradation of APS granular and crystalline structure was mainly involved and HSJ treatment was a potential physical modification technique.
Introduction
Arenga pinnata (Wurmb.) Merr is an economically important feather palm native to tropical Asia, from eastern India to Malaysia, Indonesia, the Philippines in the east, and China in the southwest. Arenga pinnata (Wurmb.) Merr starch (APS) is an important source of starch in subtropical and tropical countries. It is usually prepared from the pith of Arenga (species A. pinnata (Wurmb.) Merr).[Citation1] Physicochemical properties of APS, including pasting properties, water absorption, solubility, swelling power, thermal properties, rheological properties, and crystalline and molecular structure, have been extensively researched by various researchers.[Citation1–Citation8]
Starches from various botanical origins differ in their physical and functional properties, depending on composition, the ratio of amylose to amylopectin, crystalline structure, molecular structure, and minor endogenous constituents such as lipids and proteins.[Citation9] Meanwhile, the applications of native starch are usually limited due to its drawbacks. Most starch is modified by physical modification, chemical modification, and biotechnological modification to alter its functionality that make them ideally suited for applications in food products.[Citation10,Citation11] However, researches on the APS modification were few, especially physical modification. It is reported that heat moisture treatment could reduce gelatinization enthalpy, swelling power, viscosity of APS pastes and increase gel point concentration and hardness of APS pastes.[Citation2]
High-speed jet (HSJ) was an ultrahigh velocity jet homogenizer and was model of liquid–solid impact, which was different from dynamic high-pressure microfluidization that was model of liquid–liquid impact. Meanwhile, HSJ was different from jet cooking and spray cooking that requires temperature.[Citation12] HSJ was shown to a good physical modification of starch and it could significantly alter solubility, rheological and thermal properties, morphology, and crystalline and molecular structure of starch.[Citation12–Citation18] Presently, the study subject of HSJ modification was focused on rice starch and tapioca starch, which were A-type starches. According to X-ray diffraction (XRD) patterns, starches can be classified into A-type, B-type, and C-type, respectively. In this work, APS had a C-type pattern crystalline structure and was chosen as the study subject. Degradation of APS and its rheological properties induced by HSJ were further investigated.
Materials and methods
Materials
APS, which had 59.2% amylose content, 0.26% lipid content, and 1.1% protein content, was prepared from A. pinnata (Wurmb.) Merr cultivated in Longzhou county, Guangxi province, China. All other reagents were analytical grade.
High-speed treatment
According to the published method with modifications,[Citation12] the starch slurry (1:5) was treated by HSJ at pressures of 200 MPa for different cycles (0, 2, 4, and 8 passes), which were labeled as HSJ0, HSJ2, HSJ4, and HSJ8, respectively. After HSJ treatment, the samples were freeze-dried and then ground for analysis.
Scanning electron microscopy
APS samples were simply deposited onto carbon tape stuck on a specimen holder using a silver plate and coated with a thin film of gold in a vacuum evaporator. The obtained specimens were observed in a scanning electron microscope (SEM; Phenom Pro 05, Phenom, Netherlands) at high voltage (10 kV) to confirm the morphology of APS samples.
X-ray diffraction
X-ray scattering measurements of native and digested APS samples were performed using an XRD DI SYSTEM (BEDE Group, UK) with Cu K a radiation at 40 kV and 30 mA. XRD data were collected for 2θ between 5° and 45° at a scanning rate of 0.5°/min at room temperature using a published method.[Citation12] Relative crystallinity was calculated as the area ratio of the crystalline sharp peak over the total area using Origin 8.6 software (OriginLab Corporation, Northampton, MA, USA).[Citation14] Duplicate analyses were performed on each sample
Fourier-transform infrared spectroscopy
The Fourier-transform infrared (FTIR) spectra of the APS samples were obtained using a FTIR spectrometer (Bruker TENSON II, Germany). For each spectrum, 16 scans were obtained at a resolution of 4 cm−1. A background spectrum was recorded against an empty cell. The ratios of absorbance height 1,045/1,151 and 1,047/1,022 cm−1 were calculated to represent the long-range and short-range ordered structure of starch.[Citation19,Citation20] Duplicate analyses were performed on each sample.
Differential scanning calorimetry analysis
The thermal properties of APS treated by HSJ treatment were investigated by a differential scanning calorimeter (DSC; Q2000 DSC; TA Instruments, New Castle, DE, USA). The HSJ treatment samples were weighted into a pan and pure water was added (starch to water ratio = 1:3 (w/w)). The pan was hermetically sealed and a sealed empty pan was used as a reference. Samples were heated from 20℃ to 110℃ at a heating rate of 10 C/min. Duplicate experiments were performed for each sample.[Citation12]
Dynamic oscillatory analysis
Rheological properties were measured with a Haake Mars III rheometer (Thermo-Fisher Scientific, Waltham, MA USA) using a parallel metal plates with a diameter of 35 mm and a gap of 1,000 μm. Hundred milligrams of starch sample (db) were added into 1.0 ml water and then vortex blended. Then, the experimental conditions were based on a previously published description.[Citation12] The frequency sweep data were modeled with the following equation:
where G′ is storage modulus (Pa), f is frequency (Hz), and KG′ is the slope.
Turbidity analysis
Turbidity determination followed a previously published description.[Citation21] Starch suspensions (0.125%, 0.05 g (db) + 40 ml water) were prepared in 50-mL centrifuge tubes. The screw-capped centrifuge tube was immersed in a boiling water bath for 60 min with continuous gentle stirring, then cooled for 20 min in a 25 ± 2°C water bath with frequently vortex mixing. Initial turbidity was determined by absorbance at 640 nm[Citation22] using a UV/visible spectrophotometer (Thermo Fisher Scientific, Waltham, MA USA). The centrifuge tubes containing the remaining starch pastes were stored in a refrigerator at 4°C. After 1, 3, 5, 7, 11, and 14 days at 4°C, the centrifuge tubes were vortex mixed, and A640 was measured. Duplicate analyses were performed on each sample.
Statistical analyses
Data processing and analysis were dealt with in Origin 8.5 (OriginLab Corporation, Northamptaon, MA, USA) and significant differences between the results were calculated by analysis of variance. Differences at p < 0.05 were considered to be significant.
Results and discussions
Starch granular structure
Physical modification could significantly influence starch structures, such as packing arrangements and the overall structures of starch granules.[Citation10] HSJ treatment as a nonthermal treatments and high-pressure physical treatment had significant impacts on APS structure.
The SEM pictures of APS treated by HSJ at 200 MPa for different numbers of passes (0, 2, 4, and 8 passes) were displayed in . The most of untreated APS granules (HSJ0) were oval and kidney shapes and the size from 10 to 75 µm in diameter (). After 2 passes, the bigger granules disappeared and the remaining granules were covered a thin film. Meanwhile, flake structures were formed (). After 4 passes, the APS granules were almost degraded. Small microparticle and some rod-like structures were observed. Abundant sheet structures were also observed (). With further increases in the number of passes, the native APS granules were completely out of sight. Some tiny round particles were formed and some holes were present to the thin sheet structures. The results showed that HSJ could significantly destroy and change the APS granule structure. Fu et al.[Citation14] reported that after HSJ treatment, the rice starch granules were disrupted and exhibited partial gelatinization. Xia et al. observed that size of tapioca starch increased with increasing the treated pressure.[Citation18] Fu et al.[Citation12] also indicated that rice starch granules significantly disrupted and more aggregation was apparent with increases in the number of treated passes. However, compared with the degradation of A-type starch granules (rice and tapioca starch) induced by HSJ treatment, the C-type starch granule (APS) displayed a different degradation manner. In general, C-type starches consist of A- and B-type polymorphs; meanwhile, the A-type crystallites and B-type crystallites had different structures.[Citation23,Citation24] APS granules had a mixture of A- and B-type polymorphs. Therefore, the differential degradation might be caused by the internal crystalline structure and molecular organization.
Starch crystallinity and molecular order
As the APS granules were destroyed, the starch crystal structure might be disrupted. The XRD patterns of native APS and treated samples were presented in . The native APS showed a C-type pattern as identified by strong peak at about 5.7°, 15.1°, 17.1°, 17.9°, 20.1°, and 23.0° 2θ, which was a mixture of A- and B-type patterns. After 2 passes, the peak at 5.7° was disappeared and the peak at 17.1° was weakened, which were the characteristic peaks of B-type X-ray pattern.[Citation24] Meanwhile, the peaks at 15.1°, 17.1°, and 17.9° were the characteristic peak of A-type X-ray pattern.[Citation24] XRD illustrated that the C-type X-ray pattern was changed toward a weak A-type X-ray pattern. With further increases in the number of passes, the weak A-type pattern was changed to amorphous pattern. After HSJ treatment, the intensity of these peaks decreased with increasing the number of treated passes. The relative crystallinity also decreased with increasing the number of treated passes. The results were in agreement with SEM analysis, which was also similar with our previous results.[Citation12] Other high-pressure treatment methods, such as high-pressure homogenization and high hydrostatic pressure, have also been observed to destroy and alter the starch crystalline structure. Liu et al.[Citation25] reported that corn starch treated by high-pressure homogenization underwent a clear transformation from B-type XRD pattern toward an amorphous pattern. It has been reported that native rice starch treated by high hydrostatic pressure underwent a clear transformation from an A-type toward a weak B-type pattern.[Citation26]
HSJ treatment induced significant changes of granular and crystal structure of APS. Thus, molecular order of APS was further analyzed by FTIR spectra. FTIR was sensitive to the changes of structure on a short-range molecular level.[Citation19] The absorbencies at 1,047 and 1,022 cm−1 were associated with the ordered short-range molecule and amorphous phase, respectively. So in general, the ratio of absorbance at 1,047 and 1,022 cm−1 was calculated to represent the short-range ordered starch.[Citation20] The absorbance ratios of the APS samples were displayed in . The absorbance ratios of the APS samples deceased with increasing of the treated passes and the values of the ratios were 0.673 (HSJ0), 0.506 (HSJ2), 0.348 (HSJ4), and 0.227 (HSJ8), respectively. The FTIR results were consistent with the analysis of XRD and SEM, indicating that HSJ treatment destroyed the crystal structure and decreased the molecular order of APS. The absorbance ratio could also be used as an index to characterize the short-range alignment of double helices.[Citation27] Thus, the results also implied that the HSJ treatment could disrupt the double helix structure of APS.
Table 1. Crystallinity, molecular order, and gelatinization enthalpy of APS samples treated by HSJ treatment
Thermal analysis
The disruptions of the overall and crystal structures of APS granules were further demonstrated by DSC analysis. The gelatinization enthalpies (ΔH) of the APS samples were displayed in . The values of ΔH were 21.06, 19.23, 11.90, and 2.08 J/g, respectively (). It decreased with increasing the treatment passes, exhibiting that HSJ treatment could reduce the ΔH of APS. It was also reported that HSJ treatment could decrease the ΔH of tapioca starch[Citation18] and rice starch.[Citation14] Fu et al.[Citation12] also further suggested that HSJ treatment might make the order structure transform to amorphous fraction, which was in agreement with the FTIR results.
Turbidity analysis
Changes in turbidity of native and HSJ-treated APS pastes were displayed in . The values of the turbidities of the APS suspensions increased gradually during storage at 4℃. At the initial storage stage, HSJ2 and HSJ4 samples had the higher turbidity values than native APS (HSJ0); the HSJ8 sample showed the lowest turbidity values. After 7 days, the native APS (HSJ0) had the highest turbidity values than that of the HSJ-treated samples. And the turbidity values decrease with increasing of the treated passes. Fu et al.[Citation13] reported a similar result and suggested that degradation of starch polymer structures was responsible for the changes in turbidity. Turbidity changes during storage were also affected by swollen granules, granule fragments, soluble amylose, and amylopectin molecules and their structures, interaction between amylose and amylopectin.[Citation21,Citation28]
Rheological properties
Rheological analysis is applied to non-destructively study the starch structural information.[Citation29] Dynamic oscillatory curves of APS treated by HSJ for different cycles were showed in . During controlled heating stage, storage modules (G′) of samples (HSJ0 and HSJ2) increased significantly after 60℃ due to the starch samples gelatinization; however, G′ values of HSJ4 and HSJ8 samples decreased slightly (). Because the granules and crystalline structures of HSJ4 and HSJ8 samples were destroyed and almost changed into amorphous structure, they were easy to hydrate to form gel. Thus, at the initial temperature, the HSJ8 had the highest G′ value. As temperature increased, the molecular mobility increased and resulted in decrease of G′ mildly. At the end temperature, the HSJ0 showed the highest G′ value and G′ values decreased with the number of passes increased, which was due to the degradation of APS treated by HSJ treatment. The loss modulus (G″) had the same trend of G′ (). During controlled cooling from 95℃ to 25℃, G′ of all samples increased slightly. G′ of native APS (HSJ0) at 25℃ was higher than that of the HSJ-treated samples (). Meanwhile, the G′ values were higher than that of G″ (), indicating that the all sample gels were more solid-like than liquid-like.[Citation30]
Figure 4. Dynamic oscillatory curves of APS treated by HSJ for different cycles. (a, c) Controlled heating; (b, d) controlled cooling; and (e, f) frequency sweeping
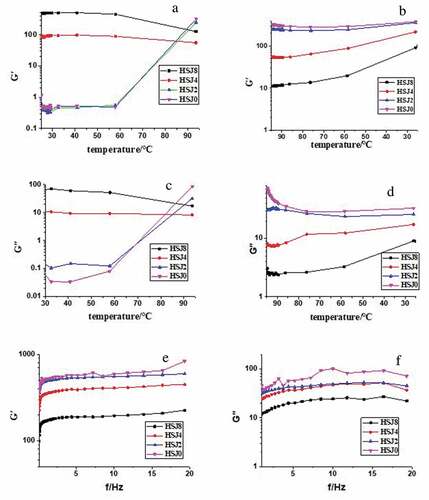
A dynamic frequency sweep is frequently used to gain further insights on the structure of bio-macromaterials.[Citation12,Citation31] A dynamic frequency sweep (0.1–20 Hz) was applied to measure the viscoelastic properties of the gels (–f). G′ and G″ decreased as the number of passes increased. Meanwhile, G′ values were always higher than the G″ values. G′ and G″ values at 10 Hz decreased from 589.9 Pa (HSJ0) to 194.9 Pa (HSJ8) and from 101.6 Pa (HSJ0) to 24.5 Pa (HSJ8), respectively, as the number of passes increased. However, the loss tangent (tan δ) increased from 0.0876 (HSJ0) to 0.0994 (HSJ8) as the number of passes increased. The results suggested that HSJ treatment made the resulting APS starch gels less elastic and more liquid-like. Fu et al.[Citation12] also reported similar result that HSJ treatment made the resulting rice starch gels less elastic and more liquid-like. The data of dynamic frequency sweep were modeled by EquationEq. (1(1)
(1) ). The coefficients of determination were 0.918 (HSJ0), 0.967 (HSJ2), 0.956 (HSJ4), and 0.922 (HSJ8), respectively (R2 > 0.92). And the slopes (KG′) were 0.094 (HSJ0), 0.073 (HSJ2), 0.0966 (HSJ4), and 0.0993 (HSJ8), respectively. The results suggested that the rheological properties of the gel of the samples treated by HSJ became more frequency-dependent as the number of passes increased.
Rheological properties of native starches depended on granular structure, molecular structure, and crystalline structure.[Citation32,Citation33] Meanwhile, degradation was also responsible for the change of viscoelasticity.[Citation13,Citation34] Therefore, the change and degradation of the APS structure might be resulted in the changes in rheological properties.
Furthermore, it has been previously found the amylose and amylopectin molecules of starch, especially the amylopectin molecules, were degraded by HSJ treatment.[Citation13] The larger starch (amylopectin) molecules were gradually degraded into smaller molecules as the number of treated passes increased.[Citation13] The extent of the degradation increased with an increase in the number of HSJ treatment passes and was accompanied by a decrease in paste viscosity, gelatinization enthalpy, and paste turbidity and the changes in rheological properties.[Citation13] Also, Further investigations are needed to clarify the mechanism of HSJ degradation of APS molecules.
Conclusion
HSJ treatment significantly changed the retrogradation and rheological properties of APS due the degradation of granular and crystalline structures. The turbidity values of pastes (long-term retrogradation), the G′ values and G″ of APS gel, and the crystallinity were decreased with increasing of the number of HSJ treatment passes. APS granule structure induced by HSJ treatment was degraded into thin sheet structures and the C-type X-ray pattern of APS was changed toward a weak A-type X-ray pattern. With further increases in the number of passes, the weak A-type pattern was changed to amorphous pattern. HSJ treatment significantly transformed the viscoelasticity of APS and had a great influence on the elasticity of the resulting APS gel. The results indicated that degradation of APS granular and crystalline structures was mainly involved and HSJ treatment was a potential physical modification technique, which might expand the potential application of APS after modified using HSJ in preparing food colloidal system and gelatinizing agent. Digestibility of APS and changes in starch molecular structure treated by HSJ will be further researched.
Additional information
Funding
References
- Adawiyah, D. R.; Sasaki, T.; Kohyama, K. Characterization of Arenga Starch in Comparison with Sago Starch. Carbohydr. Polym. 2013, 92(2), 2306–2313. DOI: 10.1016/j.carbpol.2012.12.014.
- Adawiyah, D. R.; Akuzawa, S.; Sasaki, T.; Kohyama, K. A Comparison of the Effects of Heat Moisture Treatment (HMT) on Rheological Properties and Amylopectin Structure in Sago (Metroxylon Sago) and Arenga (Arenga Pinnata) Starches. J. Food. Sci. Tech. Mys. 2017, 54(11), 3404–3410. DOI: 10.1007/s13197-017-2787-1.
- Jumaidin, R.; Sapuan, S. M.; Jawaid, M.; Ishak, M. R.; Sahari, J. Effect of Agar on Flexural, Impact, and Thermogravimetric Properties of Thermoplastic Sugar Palm Starch. Curr. Org. Synth. 2017, 14(2), 200–205. DOI: 10.2174/1570179413666160921110732.
- Sahari, J.; Sapuan, S. M.; Zainudin, E. S.; Maleque, M. A. Thermo-Mechanical Behaviors of Thermoplastic Starch Derived from Sugar Palm Tree (Arenga Pinnata). Carbohydr. Polym. 2013, 92(2), 1711–1716. DOI: 10.1016/j.carbpol.2012.11.031.
- Sahari, J.; Sapuan, S. M.; Zainudin, E. S.; Maleque, M. A. Physico-Chemical and Thermal Properties of Starch Derived from Sugar Palm Tree (Arenga Pinnata). Asian J. Chem. 2014, 26(4), 955–959. DOI: 10.14233/ajchem.
- Sanyang, M. L.; Sapuan, S. M.; Jawaid, M.; Ishak, M. R.; Sahari, J. Effect of Plasticizer Type and Concentration on Physical Properties of Biodegradable Films Based on Sugar Palm (Arenga Pinnata) Starch for Food Packaging. J Food Sci Tech MYS. 2016, 53(1), 326–336. DOI: 10.1007/s13197-015-2009-7.
- Shen, Z.; Chen, Q.; Chen, D.; Zhang, X. Study on Physical and Chemical Characteristics of Arenga Pinnota Starch. Food Sci. 2004, 25(9), 46–49.
- Ilyas, R. A.; Sapuan, S. M.; Ishak, M. R.; Zainudin, E. S. Development and Characterization of Sugar Palm Nanocrystalline Cellulose Reinforced Sugar Palm Starch Bionanocomposites. Carbohydr. Polym. 2018, 202, 186–202. DOI: 10.1016/j.carbpol.2018.09.002.
- BeMiller, J. N. Preface to the Third Edition. In Starch, 3rd ed.; Academic Press: San Diego, 2009. pp. 18-19.
- BeMiller, J. N.; Huber, K. C. Physical Modification of Food Starch Functionalities. In Annual Review of Food Science and Technology, Vol 6; Doyle, M. P., Klaenhammer, T. R., Eds.; Annual Reviews: Palo Alto, USA. 2015; 6, 19–69.
- Fu, Z.; Chen, J.; Luo, S. J.; Liu, C. M.; Liu, W. Effect of Food Additives on Starch Retrogradation: A Review. Starch-Starke. 2015, 67(1–2), 69–78. DOI: 10.1002/star.201300278.
- Fu, Z.; Luo, S. J.; BeMiller, J. N.; Liu, W.; Liu, C. M. Influence of High-Speed Jet on Solubility, Rheological Properties, Morphology and Crystalline Structure of Rice Starch. Starch-Starke. 2015, 67(7–8), 595–603. DOI: 10.1002/star.201400256.
- Fu, Z.; Luo, S. J.; BeMiller, J. N.; Liu, W.; Liu, C. M. Effect of High-Speed Jet on Flow Behavior, Retrogradation, and Molecular Weight of Rice Starch. Carbohydr. Polym. 2015, 133, 61–66. DOI: 10.1016/j.carbpol.2015.07.006.
- Fu, Z.; Luo, S.-J.; Liu, W.; Liu, C.-M.; Zhan, L.-J. Structural Changes Induced by High Speed Jet on in Vitro Digestibility and Hydroxypropylation of Rice Starch. Int. J. Food Sci. Technol. 2016, 51(4), 1034–1040. DOI: 10.1111/ijfs.13046.
- Hu, Y.; Xia, W.; Li, J.; Liu, Y.; Wang, F.; Wei, X.; Lin, Y. Effect of High Speed Jet on Structure and Properties of Tapioca Starch. Sci. Technol. Food Ind. 2018, 39(2), 56–60.
- Xia, W.; He, D.-N.; Fu, Y.-F.; Wei, X.-Y.; Liu, H.-C.; Ye, J.-P.; Liu, Y.-F.; Li, J.-H. Advanced Technology for Nanostarches Preparation by High Speed Jet and Its Mechanism Analysis. Carbohydr. Polym. 2017, 176, 127–134. DOI: 10.1016/j.carbpol.2017.08.072.
- Xia, W.; Li, J.-H.; Wang, F.; Wei, X.-Y.; Lin, -Y.-Y. Iop, Preparation and Physicochemical Properties of Nanostarches Produced by High Speed Jet. In 2017 2nd International Seminar on Advances in Materials Science and Engineering; IOP Publishing LTD: Bristol, England. 2017; Vol. 231.
- Xia, W.; Wang, F.; Li, J.; Wei, X.; Fu, T.; Cui, L.; Li, T.; Liu, Y. Effect of High Speed Jet on the Physical Properties of Tapioca Starch. Food Hydrocolloids. 2015, 49, 35–41. DOI: 10.1016/j.foodhyd.2015.03.010.
- Zhang, Y.; Liu, W.; Liu, C.; Luo, S.; Li, T.; Liu, Y.; Wu, D.; Zuo, Y. Retrogradation Behaviour of High-Amylose Rice Starch Prepared by Improved Extrusion Cooking Technology. Food Chem. 2014, 158, 255–261. DOI: 10.1016/j.foodchem.2014.02.072.
- Sevenou, O.; Hill, S. E.; Farhat, I. A.; Mitchell, J. R. Organisation of the External Region of the Starch Granule as Determined by Infrared Spectroscopy. Int. J. Biol. Macromol. 2002, 31(1), 79–85.
- Jacobson, M. R.; Obanni, M.; Bemiller, J. N. Retrogradation of Starches from Different Botanical Sources. Cereal Chem. 1997, 74(5), 511–518. DOI: 10.1094/CCHEM.1997.74.5.511.
- Miles, M. J.; Morris, V. J.; Orford, P. D.; Ring, S. G. The Roles of Amylose and Amylopectin in the Gelation and Retrogradation of Starch. Carbohydr. Res. 1985, 135(2), 271–281. DOI: 10.1016/S0008-6215(00)90778-X.
- Bogracheva, T. Y.; Morris, V. J.; Ring, S. G.; Hedley, C. L. The Granular Structure of C-Type Pea Starch and Its Role in Gelatinization. Biopolymers. 1998, 45(4), 323–332. DOI: 10.1002/(ISSN)1097-0282.
- Hoover, R.;. Composition, Molecular Structure, and Physicochemical Properties of Tuber and Root Starches: A Review. Carbohydr. Polym. 2001, 45(3), 253–267. DOI: 10.1016/S0144-8617(00)00260-5.
- Liu, D.; Wu, Q.; Chen, H.; Chang, P. R. Transitional Properties of Starch Colloid with Particle Size Reduction from Micro- to Nanometer. J. Colloid Interface Sci. 2009, 339(1), 117–124. DOI: 10.1016/j.jcis.2009.07.035.
- Li, W.; Bai, Y.; Mousaa, S. A. S.; Zhang, Q.; Shen, Q. Effect of High Hydrostatic Pressure on Physicochemical and Structural Properties of Rice Starch. Food Bioprocess. Technol. 2012, 5(6), 2233–2241. DOI: 10.1007/s11947-011-0542-6.
- van Soest, J. J. G.; Tournois, H.; de Wit, D.; Vliegenthart, J. F. G. Short-Range Structure in (Partially) Crystalline Potato Starch Determined with Attenuated Total Reflectance Fourier-Transform IR Spectroscopy. Carbohydr. Res. 1995, 279, 201–214. DOI: 10.1016/0008-6215(95)00270-7.
- Perera, C.; Hoover, R. Influence of Hydroxypropylation on Retrogradation Properties of Native, Defatted and Heat-Moisture Treated Potato Starches. Food Chem. 1999, 64(3), 361–375. DOI: 10.1016/S0308-8146(98)00130-7.
- Miri, T.;. Viscosity and Oscillatory Rheology. In Practical Food Rheology; Norton, I. T., Spyropoulos, F., Cox, P., Eds.; John Wiley & Sons: Oxford, 2011; pp 7–26.
- Kim, H.-S.; Patel, B.; BeMiller, J. N. Effects of the Amylose–Amylopectin Ratio on Starch–Hydrocolloid Interactions. Carbohydr. Polym. 2013, 98(2), 1438–1448. DOI: 10.1016/j.carbpol.2013.07.035.
- Rao, M. A.; Mckenna, B. M. Phase Transitions, Food Texture and Structure. In Texture in Food; McKenna, B. M., Ed.; CRC Press: Cambridge England, 2003; Vol. 1, pp 36–62.
- Singh, J.; Singh, N. Studies on the Morphological, Thermal and Rheological Properties of Starch Separated from Some Indian Potato Cultivars. Food Chem. 2001, 75(1), 67–77. DOI: 10.1016/S0308-8146(01)00189-3.
- Conde-Petit, B.;. 4 - the Structure and Texture of Starch-Based Foods. In Texture in Food; McKenna, B. M., Ed.; Woodhead Publishing: Cambridge, England. 2003; pp 86–108.
- Iida, Y.; Tuziuti, T.; Yasui, K.; Towata, A.; Kozuka, T. Control of Viscosity in Starch and Polysaccharide Solutions with Ultrasound after Gelatinization. Innovative Food Sci. Emerg. Technol. 2008, 9(2), 140–146. DOI: 10.1016/j.ifset.2007.03.029.