ABSTRACT
Starches were isolated from bulbs of seven lily cultivars grown in China and their morphological, structural, and physicochemical properties were investigated. All the isolated starch samples showed triangular, elliptical, less scalloped, round, and irregular shaped granules. The starch of different lily cultivars exhibited B-type crystalline pattern. The median diameters (D50) were found to be in range from 17.79 μm to 45.06 μm and the amylose content in range from 23.63 to 30.38 g/100 g starch. The weight average molar mass (Mw) of amylopectin ranged from 4.03 × 107 g/mol to 11.00 × 107 g/mol and root-mean-square rotation radius (Rz) ranged from 203.90 nm to 264.20 nm. HPAEC-PAD chain distribution analysis revealed that the average chain length of amylopectin ranged from DP 23.19–25.29. Starch samples of different lily cultivars showed peak viscosity ranging from 1699.7 cP to 2963.0 cP, and pasting temperature varying from 63.83°C to 71.00°C. Furthermore, the transition temperatures of the starch samples isolated from different lily cultivars were found to be in range from 50.76°C to 57.59°C for onset temperature (To), 54.57°C to 64.30°C for peak temperature (Tp), and 68.07°C to 77.16°C for conclusion temperature (Tc). The gelatinization temperature range (ΔTr) was found to be from 16.66°C to 21.65°C and enthalpy (ΔH) was in range from 11.46 J/g to 16.29 J/g. These results indicated that lily starch properties were significantly different depending on lily variety, which could help in understanding the convenience of these starches for the application in different food and non-food industries.
Introduction
Lily belongs to the genus Lilium of the family Liliaceae, including approximately 100 species, which are distributed throughout cold and temperate regions in the northern hemisphere. China is known as an important distribution center for the genus Lilium.[Citation1] Lily bulbs are rich in nutrients and also contain varying bioactive compounds, including phenolics, polysaccharides, steroidal saponins, and alkaloids with multiple health-promoting properties, such as antioxidant effect, anti-inflammatory effect, and immune-enhancing effect.[Citation2–Citation6] Because of its high nutritional value and health benefits, lily bulbs are commonly consumed as a vegetable and also used as a Chinese traditional medicine.[Citation7]
Among chemical constituents of lily bulbs, starch was the major component and constituted approximately 53% to 69% of the lily bulb based on dry weight[Citation8,Citation9] and the amylose content in lily bulbs starch varied from 19 to 28 g/100 g starch depending on cultivars.[Citation8,Citation10] Starch is the major storage polysaccharide of higher plants and it is deposited in partially crystalline granules varying in morphology and molecular structure between and within plant species.[Citation11] Previous studies have revealed that the shape of lily bulb starch granules varied from triangular to cylindrical with B-type crystalline pattern.[Citation10] Yu et al.[Citation8] found that the starch granules of Lilium leucanthum and Lilium rosthornii were larger in size with spherical, oval, irregular, and shell-like shape; however, Lilium lancifolium starch granules were smaller in size with a spherical and oval shape. The bulb and bulbil starches differed in their amylose content and granule size, as well as their shape and surface morphological characteristics.[Citation9] Characteristics of Lilium bulb starches were also influenced by the growth and development stages, as well as the sedimentary location of tissue parts. Histological and transcriptomic analysis showed that starch synthesis and accumulation was vital to bulblet emergence and development in triploid Lilium lancifolium.[Citation12,Citation13] Zhang et al.[Citation14] reported that the starch content of interior scales were higher than that of exterior scales. Starches from different tissue parts differed in colors, sizes, structure, morphology, and texture characteristics, which could affect the functional properties and their suitability for different industrial applications. For example, lily bulb starches from Lilium lancifolium with high viscosity were suitable to thicken soup in cooking, improving the color and taste of dishes. Lilium rosthornii exhibited a higher hydrolysis resistance, indicating that it showed different edible and medical values.[Citation8]
To the best of our knowledge, the variations in physicochemical properties, including morphology, particle size distribution, chain length distribution, molecular weight, and pasting properties of starch isolated from different tissue parts of the main Lilium species grown in China have not been studied. Therefore, the aim of this study was to investigate the morphological, structural, and physicochemical of starch isolated from the interior and exterior bulb scales of seven lily cultivars widely grown in China.
Materials and methods
Materials
Seven lily cultivars, mainly grown in China, were chosen in this study and they were as follows: 1. Lilium brownie var. viridulum (PL); 2. Lilium davidii var. unicolor (LZ); 3. Lilium regale Wilson (MJ); 4. Longya lilium (LY); 5. Lilium leucanthum (Baker) (YC); 6. Lilium lancifolium Thunb. (JD); and 7. Lilium davidii Duchartre (C). These cultivars were the mainly planted cultivars in China. After harvesting, the lily bulbs were immediately transported to laboratory and stored at 4°C. The lily bulbs sectioned into two scales, namely, exterior scales, three layers ranging from the outside to the inside; and interior scales, three layers ranging from the inside to the outside. Afterward, the sectioned bulbs with uniformity of size, free from visible blemishes, disease and/or physical damage were selected, and washed with tap water prior to starch isolation. Starch from interior scales (IS) in seven lily bulbs was recorded as PLIS, LZIS, MJIS, LYIS, YCIS, JDIS, and CIS, respectively. Starch from exterior scales (ES) in seven lily bulbs were recorded as PLES, LZES, MJES, LYES, YCES, JDES, and CES, respectively.
Starch isolation
Lily bulb starches were isolated as described by Boukhelkhal and Moulai-Mostefa[Citation15] with some modifications. The pre-washed lily scales were homogenized in twofold of distilled water for 2 min with a pulping machine to obtain a uniform slurry. The slurry was then successively filtered through a 100-mesh nylon, and the residue was washed with distilled water until no more starch was released. The residue retained on the nylon cloth was discarded. The collected suspension was centrifuged with 5000 × g at 25°C for 10 min and the supernatant was discarded. The pellet was treated with 0.05 mol/L NaOH at a ratio of 1:3 (w/v) to remove residual proteins. The mixture was kept at room temperature for 4 h and stirred every 1 h interval. The mixture was then centrifuged with 5000 × g at room temperature for 10 min, the supernatant was discarded, and the sediment was collected. These procedures were repeated four to five times until the yellow protein-containing supernatant became colorless. The starch samples were freeze-dried and sieved through a 100-mesh sieve, vacuum packaged in dark polypropylene bags, and stored in a desiccator for further analysis.
Amylose content determination
Accurately weighed sample of 20 mg starch was mixed with 1 mL of dimethyl sulfoxide, gently stirred with a vortex mixer, and heated in a boiling water bath until dispersed. Then, 2 mL of 95% (v/v) ethanol solution was added and continuously stirred on a vortex mixer. A volume of 4 mL ethanol was added, and the tube was covered with a cap, inverted, mixed, and allowed to stand for 15 min. After the resulting mixture was centrifuged with 2000 × g for 5 min, the supernatant was discarded and the sediment was used for amylose determination. The ratio of amylose/amylopectin (AM/AP) of the lily starch was measured with a special amylose/amylopectin assay kit (K-AMYL 12/16, Megazyme International Ireland Ltd.).
X-ray diffraction analysis
Lily starch was subjected to X-ray diffraction (XRD) analysis with XRD-6000 (Shimadzu, Kyoto, Japan) under the following conditions: Cu-Kα radiation operating at 40 kV and 30 mA; angle of diffraction scanning from 3° to 45° with a step length of 0.015° and scanning speed of 8°/min. To avoid the influence of humidity on the crystallinity, the starch samples were poured into glass Petri dishes, and then stored in a closed container containing saturated NaCl solution and equilibrated at room temperature for 48 h before the XRD analysis. Crystallinity index was calculated as described by Hao et al.[Citation16]
Morphological analysis
Polarized light microscope
Starch sample of 10 mg was added to a mixture of glycerol: distilled water (1:1, v/v) to make a suspension, which was dropped on a microscope slide, covered with a cover glass, and placed under a LEICA DM4500P polarizing microscope. The morphological and polarization characteristics of the starch granules were analyzed under normal light and polarized light conditions, and images were captured at 200 × magnification.
Scanning electron microscopy
A starch sample was mounted on a scanning electron microscope (SEM) (EVO LS10, Carl Zeiss, Jena, Germany) stub with a double-sided adhesive tape, and coated with gold by using an EDT-2000 ion sputter (2 × 10−4 MPa, 25 mA) for 30 s. Then, the coated samples were put into the SEM chamber and scanning electron micrographs were taken at 500 × magnifications with the signal electron type of SE1 and an accelerating voltage of 10 kV.[Citation17]
Particle size determination
A laser diffraction particle size analyzer (LS-POP laser particle size analyzer, Omec Technology Co., Ltd., China) was used to determine the particle size distribution of starch granules.[Citation18] Approximately 30 mg of lily starch was added into a 50 mL measuring cup, 10 mL of distilled water was added, and the mixture was stirred magnetically for 1 min to prepare a starch suspension. The well-dispersed suspension was poured into a rotating container until the starch was completely immersed in water, and the starch particle size distribution was determined. The following measurement conditions were used. Refractive indices (dn/dc) of water and starch were set to 1.33 and 1.60, respectively. The analysis was performed with the following conditions: a shading ratio ranged from 8% to 15%, 0.20 μm of the cutoff lower limit, and 500.00 μm of the cutoff upper limit. The particle size distribution was characterized by median diameters (D50), particle diameter of volume (D(4, 3)), particle diameter of surface (D(3, 2)) and the dispersion ((D90 − D10)/D50) as described: Abebe, Collar and Ronda.[Citation19] D10, D50, and D90 represent the corresponding particle size which is smaller than 10%, 50%, and 90% of the sample particles, respectively.
Molecular weight analysis
The molecular weight of lily starch was determined by a gel chromatography-differential multi-angle laser light scattering (GPC-RI-MALLS) using Series 1500 Pump (Waters), Optilab Trex (Wyatt Technology, CA, USA), and DAWN HELEOS Ⅱ (Wyatt Technology, CA, USA) as described by Lin et al.[Citation20] Briefly, a sample of 10 mg starch was fully dissolved in 1.5 mL of NaNO3 solution with heating, centrifuged at 12,000 × g for 10 min, and passed through a 0.22 μm filter and 100 μL of the upper column was taken. The detailed experimental conditions were as follows: detector: RI, MALLS; sample loading: 100 μL; mobile phase: DMSO/50 mmol/L LiBr; flow rate: 0.4 mL/min; column temperature: 60°C; wavelength: 663.1 nm; reference refractive index: 1.479; dn/dc: 0.07 mL/g; analytical column model: Ohpak SB-806 HQ, Ohpak SB-806 HQ, Ohpak SB-804 HQ. The Astra version 6.1 software (Wyatt Technology, Santa Barbara, CA, USA) was used for data acquisition and analysis. A secondary Berry model was used for curve fitting and calculating the molecular weight, gyration radius, and polydispersity index.
Chain length distribution analysis
A high-performance anion exchange chromatography (HPAEC-PAD; Dionex-ICS 5000; Dionex Corporation, Sunnyvale, California, USA) coupled with a pulsed amperometric detector (PAD) and a Carbopac PA-1 column (Dionex Corporation, Sunnyvale, California, USA) was used to analyze the chain length distribution of lily starches in accordance with a previously described method.[Citation21] A starch sample of 2 mg was suspended in 500 μL of 95% (v/v) ethanol solution and 4.5 mL of distilled water, and then boiled for 60 min. A volume of 2.5 mL of gelatinized polyglucan was mixed with 50 mL of 600 mmol/L sodium acetate buffer (pH 4.4) and 10 mL of NaN3 (2%, w/v). Pseudomonas amyloderamosa isoamylase (10 μL, 1000 U/μL, I5284, Sigma Aldrich Corporation, St. Louis, USA) was then added to the mixture to hydrolyze polyglucan. The hydrolysate was poured into a 600 μL tube and dried under vacuum at room temperature. The sample was dissolved in 200 μL of 0.1 mol/L NaOH for further analysis using HPAEC-PAD. The mobile phase was a gradient eluent with 100 mmol/L NaOH and 500 mmol/L sodium acetate in 100 mmol/L NaOH, and the flow rate was set as 0.4 mL/min. The peak areas corresponding to different chain lengths were examined using PeakNet (Dionex, CA, USA).
Swelling power and solubility
Swelling power and solubility were determined as described by Li et al.[Citation22] Briefly, 30 mL of 1% starch suspension (w/v) was heated in a water bath at 50°C, 60°C, 70°C, 80°C, and 90°C for 30 min. Then, the suspensions were centrifuged at 3000 × g for 20 min, and the mixture was cooled to room temperature. The sediment was weighed, and the supernatant was dried to constant weight in an oven at 105°C. The swelling power (SP) and solubility (S) of the lily starch were calculated as follows: SP (g/g) = (weight of sediment × 100)/[weight of starch sample × (1 − S)], and S (%) = weight of dried supernatant/weight of starch sample × 100.
Pasting properties analysis
The test was performed as described by Guo et al.[Citation23] The pasting properties of starch were analyzed with a rapid visco-analyzer (RVA Super-4, Newport Scientific, Australia). A starch sample of 2.0 g was weighed directly into a RVA aluminum canister and 25 mL of distilled water was added and mixed well. Samples were held at 50°C for 1 min, heated to 95°C with 12°C/min, maintained at 95°C for 2 min, cooled to 50°C at the same rate, and held for 2 min. The speed was 960 rpm for the first 10 s and subsequently held at 160 rpm.
Thermal properties analysis
Thermal properties were determined with a differential scanning calorimeter (Q2000-DSC, TA instruments, New Castle, DE, USA) as described by Peng and Yao[Citation24] with some modifications. A starch sample of 5.0 mg was placed in an aluminum DSC pan and 9 µL of deionized water was added, and sealed in the pan. The moisture content was equilibrated at room temperature for 24 h before the test. The scanning temperature was from 30°C to 110°C with heating rate of 10°C/min and an empty pan was used as a reference. Parameters, including onset temperature (To), peak temperature (Tp), conclusion temperature (Tc), and gelatinization enthalpy (ΔH) were recorded.
Statistical analysis
Data were statistically analyzed and expressed as mean ± standard deviations. One-way analysis of variance (ANOVA) and Tukey’s pairwise comparisons were performed using SPSS 20.0 with statistical significance assigned at p < .05.
Results and discussion
Amylose content
The amylose content of starch is an important indicator since it affects the structure and functional properties of starch such as swelling, solubility, viscosity, gelatinization, and retrogradation. The amylose contents of the starch samples varied significantly (p < 0.05) depending on cultivars and parts of the lily bulbs (). The amylose content ranged from 27.54 (YCIS) to 30.38 g/100 g starch (LZIS) in interior scales and from 20.94 (PLES) to 29.71 g/100 g starch (LYES) in exterior scales. These obtained results corresponded well with previous reported results[Citation8,Citation9], ranging from 24.48% to 27.66% for three Lilium bulb starches and 24.42% for Lilium lancifolium bulb starches, respectively. The amylose contents of PLIS, LZIS, and JDIS were significantly higher than those of PLES, LZES, and JDES, respectively (p < 0.05). However, there was no significant difference in the content of amylose between interior scales and exterior scales of other tested varieties (p> 0.05). Previous studies reported that the amylose content of starch was affected by the maturity of plants, climatic conditions, soil compositions, and botanical sources.[Citation11] The bulb development was divided into three stages: appearance of bulblets, bulblet formation, and bulblet development. The third phase of bulblet development was involved in considerable enlargement and growth and showed a distinct and pronounced change in bulblet size.[Citation12] In this study, the interior scales of the lily bulbs were an immature state. In addition, the synthesis and accumulation of starches were not completely finished, which could affect the chain length, branches, and amylose content. Wu et al.[Citation25] found that the number of starch granules and lipid droplets of lily bulb increased dramatically after flowering, and finally the cells were filled with the above molecules.
Table 1. Amylose contents of starches in interior and exterior scales among different lily cultivars
Crystalline patterns
The diffraction patterns of starch samples isolated from the seven lily cultivars were analyzed by XRD, and the diffractograms were shown in . The relative crystallinity calculated from the ratio of the diffraction peak area to the total diffraction area,[Citation16] were also listed in . The diffractograms revealed that all of the starch granules were consisted of the crystalline and amorphous region.[Citation26] Lily starch exhibited a typical B-type crystallinity with reflection intensities at 2θ of ~ 6°, 15°, 17°, 19°, 22°, and 24°, and the diffraction peak at 17° was the strongest, which were consistent with previous findings for lily bulb starches.[Citation10] For the same variety, the crystallinity of the exterior scales starches were higher than that of the interior scales ones. It has been reported that the crystallinity of starch was negatively correlated with amylose content.[Citation27] This phenomenon was also confirmed by the results of amylose content showed in . The relative crystallinity of the lily starch granules ranged from 13.13% to 24.95% (), which was lower than that of previous studies (20–40%) for Lilium bulb starches,[Citation8] also lower than that of rice starch (26.8–31.0%).[Citation28] The highest relative crystallinity value was found for LYES (24.95%), indicating high consistency with the double-helix pattern. Conversely, the lowest relative crystallinity was found for MJIS (13.13%). Starch crystallinity was reported to be affected by many factors, including the AM/AP ratio, the amylopectin chain length distribution, the orientation of the double helices within the crystalline region, and the interactions between the double helices.[Citation9] The higher relative crystallinity of starch was, the more heat (∆H) it would be needed.[Citation29] This was consistent with the DSC results () that LYES showed a higher enthalpy value (15.42 J/g), and MJIS presented a lower enthalpy value (11.46 J/g). The starch with predominantly small B-type starch could be used as a fat replacer, paper coating agent, and carrier material in cosmetics.[Citation30]
Table 2. Characteristic figures of the particle size distribution of starch granules and degree of relative crystallinity
Table 3. Molecule, radius, and conformation of different varieties of lily starch
Table 4. Chain length distributions of lily starch samples (%) and their average chain lengths
Table 5. Pasting properties of lily starches
Table 6. Thermal characteristics of lily starches
Morphological properties
The microscopic images of the starch samples isolated from the interior and exterior scales of seven lily cultivars as obtained under normal light microscopy (NLM), polarized light microscopy (PLM), and scanning electron microscopy (SEM) were shown in . Almost all the starch samples showed triangular and elliptical shaped granules as well as some scalloped, round, and irregular granules. The result was in agreement with the previous reports in different lily varieties.[Citation8–Citation10] In this study, MJ and YC starch granules were 2–3 times larger than those of other cultivars starch and the size of the starch granules of the interior scales were smaller than that of exterior scales. The particle size of MJ starch varied greatly compared with other varieties. This observation was consistent with the results of the particle size distribution measurement ().
Figure 2. Morphological characteristics of the starch granules under normal light (NLM), polarized light (PLM), and scanning electron microscopes (SEM)
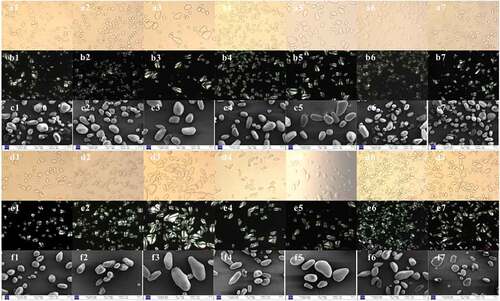
As shown in (a1–a7, d1–d7), under normal light, the ring structure of some starch granules could be detected, similar to the findings of potato and maize starch.[Citation31] Under polarized light ( b1–b7, e1–e7), all of the starch granules showed obvious birefringence patterns and a typical Maltese cross with the hila at the center of small spherical granules or at the smaller end of the starch granules. The starch granules of MJ showed a strong birefringence patterns ( b3, e3), while the granules of LZ and YC exhibited a relatively weak birefringence patterns ( b2, e2, b5, e5). The birefringence patterns of starch from exterior scales ( e1–e7) were stronger than those of interior scales ( b1–b7). It could be related to the relative crystallinity of starch, since the exterior scales, starches showed higher relative crystallinity than that of the interior scales ones as obtained by the XRD analysis (). This phenomenon was an indicator for the degree of starch granules arrangement and the organization of crystalline and amorphous regions in starch granules. Starch molecules were regularly organized in the crystallization region, so the internal crystals exhibited a certain direction. However, the starch molecules in the amorphous region were disorderly arranged.[Citation22] The difference in structures could resulted in different refractive index and density of starch. Therefore, a black polarized cross formed as the polarized light passed through the starch granules.
Particle size distribution
The particle size of starches in food crops was of importance to the food processor, since the particle size could influence the physicochemical properties of starches, such as gelatinization, pasting, enzyme susceptibility, crystallinity, and solubility, which could lead to different behaviors during food processing.[Citation29,Citation32,Citation33] The particle size distribution of lily starch granules was presented in . All the lily bulb starch samples exhibited bimodal size distribution with small peak values at 4.24 μm (IS) and 7.51 μm (ES) and large peak values in the range of 25.60 μm to 61.28 μm (). These results were different from previous findings obtained by Yu et al.[Citation8] who found unimodal distribution for lily bulb starches. This diversity could be ascribed to the different sampling methods since in this study lily bulbs were divided into interior and exterior scales while lily bulbs were sampled as a whole in the study reported by Yu et al.[Citation8]
Figure 3. Particle size distribution of seven kinds of lily starch granules, starch from interior scales (IS); starch from exterior scales (ES)
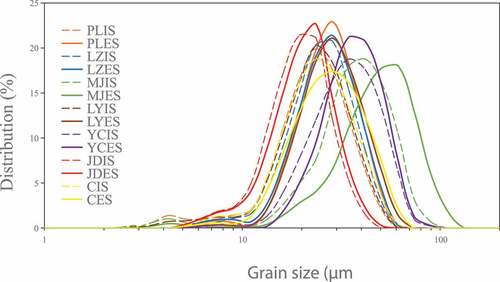
For particle size distribution of interior and exterior scales starch, it was found that two peaks of the particle size distribution of the interior scales starch shifted to the left as compared with those of particle size distribution of the exterior scales starch, respectively. It implied that the size of the interior scales starch was smaller than that of the exterior scales starch. Similarly, the median diameter (D50) of the exterior scales starch (18.56–45.06 μm) was larger than that of the interior scales starch (17.79–34.04 μm), which could be attributed to the differences in starch granule development in lily bulbs.[Citation12,Citation34]
As shown, the D50, D(4, 3), and D(3, 2) of starch from the interior and exterior scales of seven lily cultivars ranged from 17.79 μm to 45.06 μm, 18.34 μm to 46.76 μm, and 15.73 μm to 36.62 μm, respectively. The particle sizes significantly varied depending on cultivars (p< 0.05). MJES showed the highest D50 of 45.06 μm and the widest particle size range of 2.39–131.47 μm, while JDIS showed the smallest D50 values of 17.79 μm and the narrowest size distribution range of 5.13–45.06 μm. The D(4, 3) of YCIS and YCES was 33.87 μm and 32.86 μm, respectively, which was similar to the results of Lilium leucanthum (YC) starches reported by Yu et al.[Citation8] Yu et al.[Citation9] also reported that the average particle size and size distribution range were 18.44 μm and 2.47–48.41 μm for lily bulb starch originated from Lilium lancifolium (JD), respectively, which were in good agreement with the observation of this study. The size dispersion was used to characterize the uniformity of the particle size of the sample. The smaller the size dispersion was, the more uniform the particles showed. YCIS showed the lowest value of size dispersion (0.85), which was consistent with the relatively concentration of its particle size distribution (). The particle size of the lily bulb starch was larger than that of chestnut starch (6.82–8.69 μm)[Citation16] and jackfruit starch (4.28–12.59 μm).[Citation29] Starch of smaller particle size could be used as thickening agent in puddings and food formulation. The physicochemical properties and textural characteristics of food products incorporated with starch could be influenced by the particle size of starch.[Citation35]
Molecular weight
The molecular weight parameters as estimated from the GPC elution profile were presented in . Significant differences (p < 0.05) were found in the molecular weight and gyration radius of lily starches, however, no significant differences were found in the polydispersity of lily starch samples (p > 0.05). The weight average molar mass (Mw) of lily bulb starches were found to be in range of 4.03–11.00 × 107 g/mol, which was much lower than that of bean starch, 6.5–10.5 × 108 g/mol,[Citation36] and much higher than Mw of Antiaris africana seeds starch, 2.18 × 107 g/mol.[Citation37] The root-mean-square rotation radius (Rz) of amylopectin in lily starch, 203.90 nm–264.20 nm, were much lower than that of bean starch, 297 nm–345 nm.[Citation36]
The Mw was larger than the number average molar mass (Wn), and the highest Mw of 11.00 × 107 g/mol was found for JDES, and the lowest Mw of 4.03 × 107 g/mol was found for MJIS. The gyration radius (Rw) of JDES was also the highest, 221.1 nm, followed by LZES, 215.65 nm, and JDIS, 209.10 nm. However, MJIS showed the smallest Rw, 180.20 nm, which was much higher than that of Antiaris africana seeds starch, 95.10 nm.[Citation37]
The dispersity index (ÐM = Mw/Mn) was a measure of the distribution of the molecular mass in a given polymer such as starch and reflected the uniformity of polymers. The value of ÐM for the uniform polymers were approximately equal to 1, whereas it was approximately equal to 2 for the linear polymers with a high degree of polymerization and most-probable distribution.[Citation38,Citation39] In this study, the highest ÐM (2.00) was found for LZIS, whereas the smallest polydispersity (1.52) was found for MJIS, indicating heterogeneity of LZIS molecules with a higher degree of polymerization and relative uniformity of molar mass between MJIS molecules. This structural characteristic played an important role in the functionality and digestibility of lily bulb starches. The hydrodynamic coefficient (υG = LogM/LogR) reflected the molecular structure of a polymer. The values υG = 1 for a rod, υG = 0.5–0.6 for linear random coils, and υG ≈ 0.33 for a sphere attributed to high branched and dense packing of amylopectin chains.[Citation39,Citation40] The smaller υG-values, the denser the amylopectin shape was.[Citation40] The υG-values of lily bulb starches ranged from 0.15 to 0.23, which indicated that lily bulb starch molecules exhibited spherical conformation with highly branched amylopectin chains. The values for υG depended on polymer shape, temperature, and polymer-solvent interactions.[Citation40] The molecular weight and gyration radius can be affected by different planting environments, starch origins, and starch compositions.[Citation41] It also can be influenced by the method used for starch dispersion, solubilization, and the data fitting model.[Citation39]
Chain length distribution
The chain length distribution curves of the lily starch samples were shown in . The curve shapes exhibited two trough levels at the degree of polymerization (DP) 7 and DP 38 and two peaks at DP 11–14 and DP 45. These findings were consistent with distribution trends found for duckweeds starches.[Citation21] The percentage of the short-chain starch (DP 6–12) in the interior scales of lily bulb starch was higher than that in the exterior scales starch. In contrast, the percentage of the long-chain starch (DP≥37) in the exterior scales was higher than that of the interior scales starch, probably because of the difference in starch formation and development in different plant tissues.
Figure 4. Distributions of chain lengths of amylopectin starch. a: PL; b: LZ; c: MJ; d: LY; e: YC; f: JD; g: C. IS: starch from interior scales; ES: starch from exterior scales
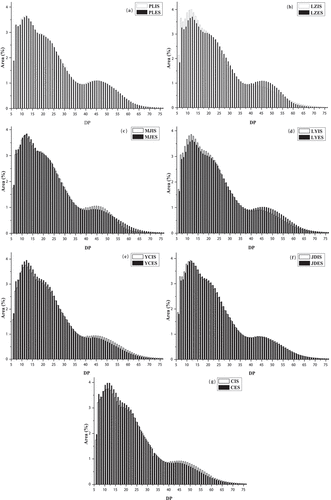
The DP and average branch chain length (DPa) of amylopectin were presented in . The values of DP were classified on the basis of the classification proposed by Hizukuri[Citation42] and the DPa of amylopectin was obtained by calculating the ratio of total glucose (DP 6–100 × their areas) to total areas of DP 6–100.[Citation30] All starches showed similar chain length distributions but differences were observed regarding botanical origin (). There were significant differences in percentages of chains with DP 6–12, DP 13–24, DP 25–36, and DP≥37, ranging from 21.26% to 24.60%, 36.17% to 39.14%, 19.06% to 20.62% and 16.89% to 22.06%, respectively (p < 0.05). LYES showed the lowest proportion of chains in DP 6–12 (21.26%), but the highest proportion of chains in DP≥37 (22.06) and value of DPa (25.29). In contrast, CES presented the highest proportion of chains in DP 6–12 (24.60%), whereas the lowest proportion of chains in DP≥37 (16.89%) and value of DPa (23.19).
More long chains (DP≥37) were detected in all tested starches, compared with those of jackfruit starches (A-type) or bean starches (C-type), ranging from 5.80% to 9.97% and 10.31% to 13.85%, respectively.[Citation37,Citation43] It suggested that lily bulb starch contained a more highly branched amylopectin than did in jackfruit starches and bean starches. This result was attributed to the fact that lily bulb starches belong to B-type starch. The proportions of short and long chains in A- and C-type starches were different from those of B-type starches. The proportions of long chains (DP>37) in B-type starches were larger than those in A- and C-type amylopectins.[Citation44] HPAEC-PAD chain distribution analysis revealed that the DPa of all lily starches were higher than 23 (), and the DP values ranged from 23.19 to 25.29, which was higher than that of jackfruit starches (DP 15.59–21.22) and bean starches (DP 19.60–21.25),[Citation36,Citation43] while lower than that of duckweed starches, DP 28.29–28.52.[Citation21] The DPa of lily starches were similar to that of some roots and tubers (waxy potato, yam, etc.), ranging from 25.8 to 26.3.[Citation39]
In this study, LYES starch showed the largest proportion of long chains, this was positively correlated with gelatinization temperature and ΔH as obtained by DSC. Branch chains with DP≥37 extended through multiple crystalline clusters, while short chains (DP < 12) were known to form short double helices. Crystalline regions packed by large proportions of longer amylopectin branch-chains were more stable, in which double helices of long branches required high temperatures for dissociation and large enthalpy changes, indicating that a high thermal energy was needed for their disruption.[Citation28,Citation36]
Swelling power and solubility
The swelling power and solubility of all the lily starches were determined at a temperature range of 50–90°C ( and ). The results showed that the swelling power and solubility of lily starch increased as temperature increased. The similar trend was found for purple sweet potato starches.[Citation44]
Figure 5. Swelling power of starch granules in the interior and exterior scales of seven kinds of lily bulbs. a: starch from interior scales (IS); b: starch from exterior scales (ES)
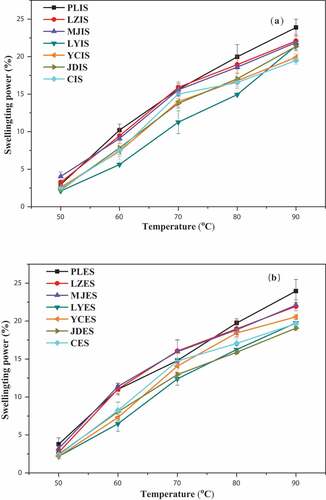
Figure 6. Solubility of starch granules in the interior and exterior scales of seven kinds of lily bulbs. a: starch from interior scales (IS); b: starch from exterior scales (ES)
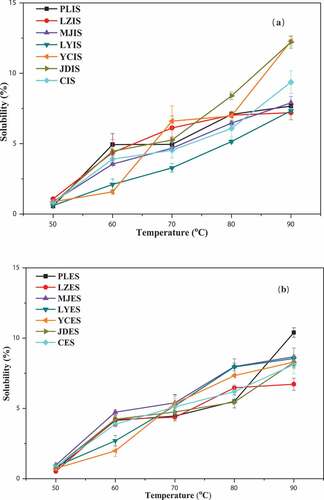
No significant difference was observed in the swelling power between the interior and exterior scales from the same lily cultivar bulb and a similar phenomenon was also shown for the solubility of the starches (p > 0.05). However, the different cultivars starches exhibited significantly different swelling power and solubility (p < 0.05), which could be attributed to the difference in the structure and genetic mapping of the starch granules.[Citation1] The starch isolated from the interior and exterior scales of PL showed higher swelling power, 2.96–23.96%, at 50–90°C, whereas the starch from the interior and exterior scales of LY showed lower swelling power, 2.09–21.43%, at 50–90°C. The difference in swelling power was not obvious at 50°C. However, PL starch showed the highest swelling power at 90°C, 23.88% for the interior scales and 23.96% for the exterior scales starch. On the other hand, the interior and exterior scales of C and JD starches showed the smallest swelling powers at 90°C, 19.46%, and 19.07%, respectively. Similar trend of results were found in swelling power of starch from 10 mung bean cultivars, 17.27–20.55%.[Citation45] The solubility of the interior and exterior scales starch of the seven lily bulbs increased as temperature increased from 50°C to 90°C, ranging from 0.52% to 12.28%. The solubility also varied among different lily cultivars at the same temperature. PLES showed the highest swelling power at 90°C, it would be explained the fact that starch of PLES contained the lowest amylose content (20.94%). However, JDES showed the smallest swelling powers (19.07%) at 90°C, which could be attributed to the relatively smaller of particle size (18.56 μm) as showed in . Both swelling power and solubility reflected the ability of lily starch granules to bind water molecules and the ability to interact with each other. When starch was heated in excessive water, the crystal structure was damaged due to the fracture of the hydrogen bond. The hydroxyl groups of the exposed amylose and amylopectin formed hydrogen bond association with water, causing an increase in swelling power and solubility.[Citation22] Swelling power and solubility were influenced by the particle size, molecular weight, lipid-amylose complexes, amylose-to-amylopectin ratio, and the interaction between starch chains within the amorphous and crystalline regions.[Citation22,Citation46]
Pasting properties
The pasting properties of the starches isolated from different parts and cultivars of lily as obtained by RVA were presented in , and the corresponding pasting RVA curves were shown in . Pasting parameters, including peak viscosity (PV), trough viscosity (TV), breakdown (BD), final viscosity (FV), setback (SB), pasting temperature (GT), and peak time (PT), of the lily starches varied significantly depending on lily cultivars and tissue parts (p < 0.05). These results were consistent with previous findings for Lilium bulb starches obtained by Yu et al.[Citation8] The PV, TV, and FV of the starches from the exterior scales of most lily cultivars were higher than those of the interior scales starch.
Figure 7. Rapid visco analyzer profile of starches isolated from different parts and cultivars of lily. a: starch from interior scales (IS); b: starch from exterior scales (ES)
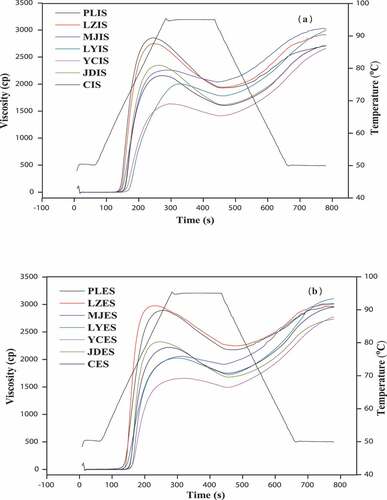
PV was correlated with the range of starch size distribution and indicated the resistance of swollen granules to shear and the performance of starches.[Citation18] The PV varied from 1699.67 to 2963.00 cp. The PV of LZES (2963.00 cp) and PLES (2934.33 cp) were significantly higher than other varieties (p < 0.05), while the PV of YCIS (1699.67 cp) was the lowest among all varieties, indicating lower resistance of YCIS to swelling and rupture than that of the other starches.[Citation47] This was highly consistent with the conclusion that PLES exhibited the highest swelling power ().The relatively lower PV of YC indicated that these starches could be suitable for high-temperature processed and as materials for delivery systems.[Citation21]
The BD was defined as the difference between PV and TV and could be used to evaluate the shear resistance of starches at high temperatures. The smaller the BD value was, the better the starch paste stability presented. In this study, BD varied significantly from 139.00 to 950.67 cp. The lowest BD value (139.00 cp) was found for YCES starch, indicating strong cohesive forces within its starch granules, high thermal stability, and low retrogradation tendency. The BD could be influenced by the presence of rigid non-fragmented swollen granules, the granule size, and the amount of leached amylose.[Citation22,Citation48]
FV indicated the stability of cooled-cooked paste under low shear. However, SB reflected the gelling stability and retrogradation tendency of starch and it was correlated with the rearrangement of amylose molecules.[Citation22] The FV and SB ranged from 2694.00 to 3152.00 cp and 760.00 to 1398.67 cp, respectively. LYES showed the highest FV value, whereas the lowest was observed for CIS. LZES showed the lowest SB value, indicating lower retrogradation tendencies and higher stability of its cooled paste. The differences in the aggregation of starch paste during cooling could be due to the different particle size and amylose content of lily starches.[Citation49]
The pasting temperature provides an indication of the minimum temperature required to cook, as well as the temperature at which the viscosity begins to increase during the heating process.[Citation50] As showed in and , all pasting temperatures differed significantly among the interior and exterior scales from the seven lily cultivars (p < .05) ranging from 63.83°C to 71.00°C. LYIS showed the highest pasting temperature, whereas the lowest was found for PLES. It indicated that LYIS showed higher resistance to swelling. This finding was in agreement with the SP results (). It was also reflected in () that LYIS presented higher transition temperatures but lower in PLES. These values were also in agreement with previous results.[Citation8,Citation10]
Thermal properties
The thermal parameters of lily starch as obtained by DSC were presented in . The transition temperatures (To, Tp, and Tc), gelatinization temperature range (ΔTr), and ΔH of starch varied significantly depending on lily cultivars and tissue parts (p < 0.05). To, Tp, and Tc of the starches from different lily cultivars ranged from 50.76°C to 57.59°C, 54.57°C to 64.30°C, and 68.07°C to 77.16°C, respectively. The difference in transition temperatures could be attributed to the particle size, morphological characteristics of the starch granules, the degree of crystallization, the distribution of amylopectin chain length, the ratio of amylose and amylopectin, the molecular weight of starch, and intermolecular interaction.[Citation51] ΔTr ranged from 16.66°C (MJES) to 21.65°C (LZES), and this range was correlated with the uniformity of granule size and the degree of crystalline organization in the starch molecules.[Citation52] In terms of the uniformity of particle size distribution of starch granules (), MJES showed the highest, whereas LZES showed the lowest particle size distribution. It has been reported that ΔH was mainly due to the disruption of double helices rather than the long-range disruption of crystallinity.[Citation21] ΔH of the starch samples varied from 11.46 J/g to 16.29 J/g. The JDES presented the highest ΔH, whereas the lowest was observed for MJES. The higher ΔH of JDES suggested that disruption of double helices during gelatinization was more pronounced than that of other lily bulb starches. This indicated that more energy could be needed to break the intermolecular bonds in starch granules of JDES to achieve gelatinization.
Conclusion
This study demonstrated the diversity in morphological, structural, and physicochemical properties of starches isolated from different lily cultivars and tissues. The structural and physicochemical properties of lily bulb starches varied significantly depending on lily cultivars and bulbs tissues parts, i.e. the interior and exterior scales of lily bulbs. The relative crystallinity, granule sizes, molecular weight, average chain length, peak viscosity, and transition temperatures of the starches from the exterior scales were found to be higher than those of the interior scales starch. However, the amylose content of the exterior scales starch was found to be lower than that of the interior scales starch. Lily starches were triangular and elliptical granules along with few scalloped, round, and irregular granules. All lily starches from seven cultivars exhibited B-type crystallinity, but showed some differences in physicochemical properties. The granule sizes were high in MJ and YC and low in JD, whereas MJ presented the lowest relative crystallinity and molecular weight. LY and PL starch showed high and low thermal temperature. PL starch showed a high peak and breakdown viscosities, and YC starch exhibited low peak and breakdown viscosities. These results could be used as a basis for the research and development of lily starch incorporated products.
Acknowledgments
The study was supported by National Natural Science Foundation of China (31501543), Hunan Provincial Key Research Project, China (2017NK2112), National Key Research Project of China (2017YFD0400902-2), and Agricultural Science and Technology Innovation Project of Hunan Province, China (2017GC03). We also appreciate the technical support of molecular weight analysis provided by Jiangsu Sanshu Boitechnology Co., Ltd.
Additional information
Funding
References
- Du, Y. P.; He, H. B.; Wang, Z. X.; Wei, C.; Li, S.; Jia, G. X. Investigation and Evaluation of the Genus Lilium Resources Native to China. Genet. Resour. Crop Evol. 2014, 61, 395–412. DOI: 10.1007/s10722-013-0045-6.
- Hong, X. X.; Luo, J. G.; Guo, C.; Kong, L. Y. New Steroidal Saponins from the Bulbs of Lilium Brownii Var. Viridulum. Carbohydr. Res. 2012, 361, 19–26. DOI: 10.1016/j.carres.2012.07.027.
- Jin, L.; Zhang, Y.; Yan, L.; Guo, Y.; Niu, L. Phenolic Compounds and Antioxidant Activity of Bulb Extracts of Six Lilium Species Native to China. Molecules. 2012, 17, 9361–9378. DOI: 10.3390/molecules17089361.
- Kwon, O. K.; Lee, M. Y.; Yuk, J. E.; Oh, S. R.; Chin, Y. W.; Lee, H. K.; Ahn, K. S. Anti-Inflammatory Effects of Methanol Extracts of the Root of Lilium Lancifolium on PLS-stimulated Raw264.7 Cells. J. Ethnopharmacol. 2010, 130, 28–34.
- Gao, J.; Zhang, T.; Jin, Z. Y.; Xu, X. M.; Wang, J. H.; Zha, X. Q.; Chen, H. Q. Structural Characterisation, Physicochemical Properties and Antioxidant Activity of Polysaccharide from Lilium Lancifolium Thunb. Food Chem. 2015, 169, 430–438. DOI: 10.1016/j.foodchem.2014.08.016.
- Pan, G. F.; Xie, Z. W.; Huang, S. X.; Tai, Y. L.; Cai, Q. S.; Jiang, W.; Sun, J.; Yuan, Y. Immune-Enhancing Effects of Polysaccharides Extracted from Lilium Lancifolium Thunb. Int. Immunopharmacol. 2017, 52, 119–126. DOI: 10.1016/j.intimp.2017.08.030.
- Huang, H.; Ge, Z.; Limwachiranon, J.; Li, L.; Li, W.; Luo, Z. UV-C Treatment Affects Browning and Starch Metabolism of Minimally Processed Lily Bulb. Postharvest. Biol. Technol. 2017, 128, 105–111. DOI: 10.1016/j.postharvbio.2017.02.010.
- Yu, X.; Zhang, J.; Li, A.; Wang, Z.; Xiong, F. Morphology and Physicochemical Properties of 3 Lilium Bulb Starches. J. Food Sci. 2015, 80, C1661–C1669. DOI: 10.1111/1750-3841.12969.
- Yu, X.; Zhang, J.; Shao, S.; Yu, H.; Xiong, F.; Wang, Z. Morphological and Physicochemical Properties of Bulb and Bulbil Starches from Lilium Lancifolium. Starch- Stärke. 2015, 67, 448–458. DOI: 10.1002/star.201400209.
- Li, X.; Gao, W.; Jiang, Q.; Xia, Y. Physicochemical, Morphological, and Thermal Properties of Starches Separated from Bulbs of Four Chinese Lily Cultivars. Starch-Stärke. 2012, 64, 545–551. DOI: 10.1002/star.v64.7.
- Sandhu, K. S.; Siroha, A. K. Relationships between Physicochemical, Thermal, Rheological and in Vitro, Digestibility Properties of Starches from Pearl Millet Cultivars. LWT Food Sci. Technol. 2017, 83, 213–224. DOI: 10.1016/j.lwt.2017.05.015.
- Li, X.; Wang, C.; Cheng, J.; Zhang, J.; Da, S. J.; Liu, X.; Xin, D.; Li, T.; Sun, H. Transcriptome Analysis of Carbohydrate Metabolism during Bulblet Formation and Development in Lilium Davidii Var. Unicolor. BMC Plant Biol. 2014, 14, 358–369. DOI: 10.1186/s12870-014-0358-4.
- Yang, P.; Xu, L.; Xu, H.; Tang, Y.; He, G.; Cao, Y.; Feng, Y.; Yuan, S.; Ming, J. Histological and Transcriptomic Analysis during Bulbil Formation in Lilium Lancifolium. Front. Plant Sci. 2017, 8, 1508. DOI: 10.3389/fpls.2017.01508.
- Zhang, Y.; Yong, Y. B.; Wang, Q.; Lu, Y. M. Physiological and Molecular Changes during Lily Underground Stem Axillary Bulbils Formation. Russ. J. Plant Physiol. 2018, 65, 372–383. DOI: 10.1134/S1021443718030172.
- Boukhelkhal, M.; Moulai-Mostefa, N. Physicochemical Characterization of Starch Isolated from Soft Acorns of Holm Oak (Quercus Ilex Subsp. Ballota (Desf.) Samp.) Grown in Algeria. J. Food Meas. Charact. 2017, 11, 1–11. DOI: 10.1007/s11694-017-9582-6.
- Hao, H.; Li, Q.; Bao, W.; Wu, Y.; Ouyang, J. Relationship between Physicochemical Characteristics and in Vitro, Digestibility of Chestnut (Castanea Mollissima) Starch. Food Hydrocolloids. 2018, 84, 193–199. DOI: 10.1016/j.foodhyd.2018.05.031.
- Li, W.; Cao, F.; Fan, J.; Ouyang, S.; Luo, Q.; Zheng, J.; Zhang, G. Physically Modified Common Buckwheat Starch and Their Physicochemical and Structural Properties. Food Hydrocolloids. 2014, 40, 237–244. DOI: 10.1016/j.foodhyd.2014.03.012.
- Li, W.; Shan, Y.; Xiao, X.; Luo, Q.; Zheng, J.; Ouyang, S.; Zhang, G. Physicochemical Properties of A- and B-Starch Granules Isolated from Hard Red and Soft Red Winter Wheat. J. Agric. Food Chem. 2013, 61, 6477–6484. DOI: 10.1021/jf400943h.
- Abebe, W.; Collar, C.; Ronda, F. Impact of Variety Type and Particle Size Distribution on Starch Enzymatic Hydrolysis and Functional Properties of Tef Flours. Carbohydr. Polym. 2015, 115, 260–268. DOI: 10.1016/j.carbpol.2014.08.080.
- Lin, P. C.; Wu, D. T.; Xie, J.; Zhao, J.; Li, S. P. Characterization and Comparison of Bioactive Polysaccharides from the Tubers of Gymnadenia Conopsea. Food Hydrocolloids. 2015, 43, 199–206. DOI: 10.1016/j.foodhyd.2014.05.015.
- Chen, L.; Yu, C.; Ma, Y.; Xu, H.; Wang, S.; Wang, Y.; Liu, X.; Zhou, G. Insights into the Structural and Physicochemical Properties of Small Granular Starches from Two Hydrophyte Duckweeds, Spirodela Oligorrhiza and Lemna Minor. Carbohydr. Res. 2016, 435, 208–214. DOI: 10.1016/j.carres.2016.10.010.
- Li, W.; Xiao, X.; Zhang, W.; Zheng, J.; Luo, Q.; Ouyang, S.; Zhang, G. Compositional, Morphological, Structural and Physicochemical Properties of Starches from Seven Naked Barley Cultivars Grown in China. Food Res. Int. 2014, 58, 7–14. DOI: 10.1016/j.foodres.2014.01.053.
- Guo, Z.; Zeng, S.; Lu, X.; Zhou, M.; Zheng, M.; Zheng, B. Structural and Physicochemical Properties of Lotus Seed Starch Treated with Ultra-High Pressure. Food Chem. 2015, 186, 223–230. DOI: 10.1016/j.foodchem.2015.03.069.
- Peng, X.; Yao, Y. Small-Granule Starches from Sweet Corn and Cow Cockle: Physical Properties and Amylopectin Branching Pattern. Food Hydrocolloids. 2017, 74, 349–357. DOI: 10.1016/j.foodhyd.2017.08.025.
- Wu, S. S.; Wu, J. D.; Jiao, X. H.; Zhang, Q. X.; Lv, Y. M. The Dynamics of Changes in Starch and Lipid Droplets and Sub-Cellular Localization of β-amylase during the Growth of Lily Bulbs. J. Integr. Agric. 2012, 11, 585–592. DOI: 10.1016/S2095-3119(12)60045-8.
- Zhu, B.; Ma, D.; Wang, J.; Zhang, S. Structure and Properties of Semi-Interpenetrating Network Hydrogel Based on Starch. Carbohydr. Polym. 2015, 133, 448–455. DOI: 10.1016/j.carbpol.2015.07.037.
- Lin, L.; Guo, D.; Zhao, L.; Zhang, X.; Wang, J.; Zhang, F.; Wei, C. Comparative Structure of Starches from High-Amylose Maize Inbred Lines and Their Hybrids. Food Hydrocolloids. 2016, 52, 19–28. DOI: 10.1016/j.foodhyd.2015.06.008.
- Zhu, D.; Zhang, H.; Guo, B.; Xu, K.; Dai, Q.; Wei, C.; Zhou, J.; Huo, Z. Physicochemical Properties of Indica-Japonica, Hybrid Rice Starch from Chinese Varieties. Food Hydrocolloids. 2017, 63, 356–363. DOI: 10.1016/j.foodhyd.2016.09.013.
- Zhang, Y.; Zhu, K.; He, S.; Tan, L.; Kong, X. Characterizations of High Purity Starches Isolated from Five Different Jackfruit Cultivars. Food Hydrocolloids 2016, 52, 785–794.
- Lin, L.; Cai, C.; Gilbert, R. G.; Li, E.; Wang, J.; Wei, C. Relationships between Amylopectin Molecular Structures and Functional Properties of Different-Sized Fractions of Normal and High-Amylose Maize Starches. Food Hydrocolloids. 2016, 52, 359–368. DOI: 10.1016/j.foodhyd.2015.07.019.
- Li, J. H.; Guiltinan, M. J.; Thompson, D. B. The Use of Laser Differential Interference Contrast Microscopy for the Characterization of Starch Granule Ring Structure. Starch-Stärke. 2006, 58, 1–5. DOI: 10.1002/star.200500432.
- Hera, E. D. L.; Gomez, M.; Rosell, C. M. Particle Size Distribution of Rice Flour Affecting the Starch Enzymatic Hydrolysis and Hydration Properties. Carbohydr. Polym. 2013, 98, 421–427. DOI: 10.1016/j.carbpol.2013.06.002.
- Zeng, J.; Gao, H.; Li, G. Functional Properties of Wheat Starch with Different Particle Size Distribution. J. Sci. Food Agric. 2013, 94, 57–62. DOI: 10.1002/jsfa.6186.
- Bechtel, D. B.; Wilson, J. D. Amyloplast Formation and Starch Granule Development in Hard Red Winter Wheat. Cereal Chem. 2003, 80, 175–183. DOI: 10.1094/CCHEM.2003.80.2.175.
- Abiodun, O.;. Effect of Pre-Cooking and Particle Size Distribution on the Pasting and Functional Properties of Trifoliate (Dioscorea Dumetorum) Yam Flour. Br. J. Appl. Sci. Technol. 2013, 3, 847–859. DOI: 10.9734/BJAST/2013/3299.
- Demiate, I. M.; Figueroa, A. M.; Santos, T. P. R. D.; Yangcheng, H.; Chang, F.; Jane, J. L. Physicochemical Characterization of Starches from Dry Beans Cultivated in Brazil. Food Hydrocolloids. 2016, 61, 812–820. DOI: 10.1016/j.foodhyd.2016.07.014.
- Nwokocha, L. M.; Nwokocha, K. E.; Williams, P. A. Physicochemical Properties of Starch Isolated from Antiaris Africana Seeds in Comparison with Maize Starch. Starch-Stärke. 2012, 64, 246–254. DOI: 10.1002/star.v64.3.
- Stepto, R. F. T.;. Dispersity in Polymer Science (IUPAC Recommendations 2009). Pure Appl. Chem. 2009, 81, 351–353. DOI: 10.1351/PAC-REC-08-05-02.
- Hoyos-Leyva, J. D.; Bello-Pérez, L. A.; Alvarez-Ramirez, J.; Agama-Acevedo, E. Structural Characterization of Aroid Starches by Means of Chromatographic Techniques. Food Hydrocolloids. 2017, 69, 97–102. DOI: 10.1016/j.foodhyd.2017.01.034.
- Rolland-Sabaté, A.; Sánchez, T.; Buléon, A.; Colonna, P.; Jaillais, B.; Ceballos, H.; Dufour, D. Structural Characterization of Novel Cassava Starches with Low and High-Amylose Contents in Comparison with Other Commercial Sources. Food Hydrocolloids. 2012, 27, 161–174. DOI: 10.1016/j.foodhyd.2011.07.008.
- Zhang, Y.; Zhang, Y.; Fei, X.; Li, S.; Tan, L. Structural Characterization of Starches from Chinese Jackfruit Seeds (Artocarpus Heterophyllus Lam). Food Hydrocolloids. 2018, 80, 141–148. DOI: 10.1016/j.foodhyd.2018.02.015.
- Hizukuri, S.;. Polymodal Distribution of the Chain Lengths of Amylopectins, and Its Significance. Carbohydr. Res. 1986, 147, 342–347. DOI: 10.1016/S0008-6215(00)90643-8.
- Zhang, Y.; Zhang, Y.; Xu, F.; Wu, G.; Tan, L. Molecular Structure of Starch Isolated from Jackfruit and Its Relationship with Physicochemical Properties. Sci. Rep. 2017, 7, 13423. DOI: 10.1038/s41598-017-13435-8.
- Zhang, L.; Zhao, L.; Bian, X.; Guo, K.; Zhou, L.; Wei, C. Characterization and Comparative Study of Starches from Seven Purple Sweet Potatoes. Food Hydrocolloids. 2018, 80, 168–176. DOI: 10.1016/j.foodhyd.2018.02.006.
- Li, W.; Chang, S.; Zhang, P.; Shen, Q. Properties of Starch Separated from Ten Mung Bean Varieties and Seeds Processing Characteristics. Food Bioprocess. Technol. 2011, 4, 814–821. DOI: 10.1007/s11947-010-0421-6.
- Yu, S.; Ma, Y.; Menager, L.; Sun, D. W. Physicochemical Properties of Starch and Flour from Different Rice Cultivars. Food Bioprocess. Technol. 2012, 5, 626–637. DOI: 10.1007/s11947-010-0330-8.
- Zhang, H.; Yin, L.; Zheng, Y.; Shen, J. Rheological, Textural, and Enzymatic Hydrolysis Properties of Chickpea Starch from a Chinese Cultivar. Food Hydrocolloids. 2016, 54, 23–29. DOI: 10.1016/j.foodhyd.2015.09.018.
- Sun, Q.; Han, Z.; Wang, L.; Xiong, L. Physicochemical Differences between Sorghum Starch and Sorghum Flour Modified by Heat-Moisture Treatment. Food Chem. 2014, 145, 756–764. DOI: 10.1016/j.foodchem.2013.08.129.
- Kim, Y. Y.; Woo, K. S.; Chung, H. J. Starch Characteristics of Cowpea and Mung Bean Cultivars Grown in Korea. Food Chem. 2018, 263, 104–111. DOI: 10.1016/j.foodchem.2018.04.114.
- Zhang, Y.; Hu, M.; Zhu, K.; Wu, G.; Tan, L. Functional Properties and Utilization of Artocarpus Heterophyllus Lam Seed Starch from New Species in China. Int. J. Biol. Macromol. 2018, 107, 1395–1405. DOI: 10.1016/j.ijbiomac.2017.10.001.
- Hoyos-Leyva, J. D.; Bello-Pérez, L. A.; Yee-Madeira, H.; Rodriguez-Garcia, M. E.; Aguirre-Cruz, A. Characterization of the Flour and Starch of Aroid Cultivars Grown in Mexico. Starch-Stärke. 2017, 69, 1600370. DOI: 10.1002/star.201600370.
- Lemos, A. M.; Abraão, A. S.; Cruz, B. R.; Morgado, M. L.; Rebelo, M.; Nunes, F. M. Effect of Granular Characteristics on the Viscoelastic and Mechanical Properties of Native Chestnut Starch (Castanea Sativa Mill). Food Hydrocolloids. 2015, 51, 305–317. DOI: 10.1016/j.foodhyd.2015.05.021.