ABSTRACT
The present study was conducted to evaluate the effects of storage time and temperature on physicochemical and sensory properties and to measure the spoilage of pasteurized fluid milk stored at different temperatures via nanoparticles on pasteurized fluid milk. The mean values for pH of milk samples decreased (from 6.81 to 6, 5.46, and 4.37), acidity increased (10%, 21%, and 35%), fat contents decreased (13%, 24%, and 32%), protein contents decreased (7%, 18%, and 24%), lactose contents decreased (5%, 7%, and 12%), and total free fatty acids increased (14%, 19%, and 27%) respectively at 4°C, 7°C, and 10°C. Similarly, the score of sensory parameters like aroma, taste, and overall acceptability decreased 16%, 23%, and 45%; 31%, 43%, and 52%; and 33%, 42%, and 53% respectively in linear pattern along with increasing the time and temperature. The L* values were non-significantly changed or decreased with mean values of 64.82 ± 0.08 to 64.13 ± 0.07 at 4°C and significantly with 64.81 ± 0.03 to 63.87 ± 0.07 and 64.77 ± 0.02 to 61.43 ± 0.05 at 7°C and 10°C respectively at 0–19th day of milk storage. During storage, the pasteurized milk stored at all temperatures 4°C, 7°C, and 10°C showed that a* value non-significantly decreased from 48.69 ± 0.18 to 48.63 ± 0.18, 48.74 ± 0.14 to 48.57 ± 0.11, and 48.68 ± 0.18 to 47.97 ± 0.07 respectively. Values of b* decreased non-significantly at 4°C and 7°C with the values of −17.56 ± 0.23 to −18.58 and −17.54 ± 0.25 to −19.85 ± 0.85 but significantly decreased at 10°C from −17.56 ± 0.23 to −43.22 ± 0.11. The results suggested that pasteurized milk can be kept for 17–19 days at 4°C but this shelf life drastically reduced at 7°C and 10°C. Color of nanoparticles was significantly changed from pink to dark blue from 14th day of storage onwards to the end at 10°C. Conclusively, nanoparticles can be used for the rapid and at the post-spoilage measurement of pasteurized milk.
Introduction
Physicochemical reactions during storage of food especially pasteurized milk may result in unwanted changes which undermine its sensory and nutritional qualities. Changes in the physicochemical properties on pasteurized milk may lead to deterioration of product quality and impart a negative cause of the shelf life of the product. Contamination of milk with psychrotrophic bacteria is actually considered to be the crucial control component impacting the preventing condition of pasteurized milk.[1] The ingredients that control shelf life of refrigerated pasteurized milk products are (1) time and temperature of pasteurization; (2) presence and actual post-pasteurization pollutants; (3) type and activity of pasteurization-resistant microorganisms; and (4) storage temperature after pasteurization.[Citation2] Although pasteurization is actually supposed to inactivate pathogenic bacteria in milk, it usually does not damage all of bacteria which are present in the product. As a result, extending sell by dates above 21 days is not recommended, even under the best of processing facilities.[Citation3] Because of improper refrigeration, low-quality raw material, and insufficient packaging system, the maintenance of milk quality is huge problem in many countries.[Citation4] Moreover, high temperature short time (HTST) pasteurized and refrigerated milk has a shelf life of 7–21 days.[Citation5] Bacterial spoilage is actually the key limiting factor in bringing the shelf life of general HTST pasteurized milk beyond 14 days.
Figure 8. Relationship of sensory scores with color variation of a* for spoilage detection of pasteurized milk stored at 7°C
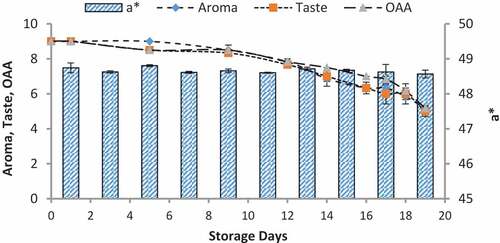
Figure 11. Relationship of sensory scores with color variation of b* for spoilage detection of pasteurized milk stored at 7°C
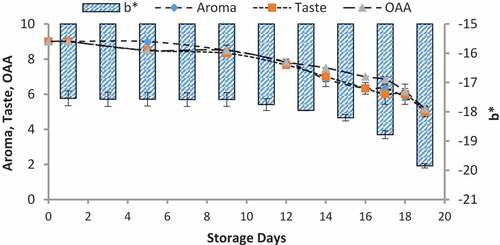
Microbial growth and metabolism shorten the shelf life of milk through creating undesirable changes in aroma and taste features which influence customer acceptability of the products.[Citation6] Factors limiting pasteurized milk stability are usually established as bacterial contamination, inadequate packaging system, and improper temperature control. Vulnerability of milk’s fat and protein to physicochemical changes can also result in deterioration, thus preventing its condition. Factors which influence the shelf life of pasteurized milk include the condition of raw material, the binomial temperatures/time pasteurization, the resistant microorganisms to pasteurization, the presence and activity of post-pasteurization pollutants, the packaging system, and the post-pasteurization storage temperature that had impact on the stability of the product.[Citation7] Food and dairy manufacturers, agricultural producers, and consumers could gain a more competitive position through application of nanotechnology. Nanotechnology will replace many fields with tremendous application potential in the area of dairy and food sectors.[Citation8] The current nanotechnology applications in food science provide the detection of food pathogens, through nano-sensors, which are quick, sensitive, and less labor-intensive procedures. With the increasing health consciousness among consumers, it is possible to use nano-sensors in plastic packaging to detect gases released due to food spoilage.[Citation9]
Milk undergoes spoilage with the production of different aldehydes, ketones, and alcohols by the hydrolysis of lipids, proteins, and certain vitamins of milk. This lipolysis and proteolysis may take place with several agents and factors like light, packing material, storage temperature and time, enzymatic activity, and certain psychotropic bacteria that encourage the photooxidation and other biochemical and enzymatic reactions that spoil the pasteurized milk during storage.[Citation10]
Different techniques and methods have been used for the detection of milk spoilage like pH and acidity measurement, microbial count, polymerase chain reaction (PCR), Fourier-transform infrared spectroscopy, gas chromatography, etc. Nanotechnology-based materials provide the potential for rapid detection and monitoring of contamination at various steps in food supply chains thereby reducing costly recalls and as a solution for the detection of many of food quality and contamination problems. Microbes cause the production of aldehydes, ketones, hydrocarbons, and alcohols during food spoilage as metabolic by-products. Detecting these important spoilage indicators in packaged foods would provide a means to predict remaining shelf life.
Materials and methods
The current study was conducted in Food Engineering Laboratory (II) of Prof. Dr Barbara Rasco and Food Engineering Laboratory (I) of Assistant Prof. Dr Sablani, School of Food Science, Washington State University, USA.
Procurement of samples
Commercially available pasteurized milk with 3.25% standardized fat with same date of production was procured from Wallmart Superstore of Darigold company brand, Inc. Seattle, Washington 98119, USA and transferred under sterilized conditions into 250-ml sterilized plastic vessel and a total of 30 samples were prepared and subjected for following conditions (Storage at 4°C, 7°C, and 10°C for 19 days with the time interval of 0, 1, 5, 9, 12, 14, 16, 17, 18, and 19 days). On the basis of these predetermined levels, we used the same amount of standards of these volatile compounds that reacted nanoparticles coated with reagent in this experiment.
Acidity, pH, protein, and lactose contents
The titratable acidity was estimated with titration method and pH was determined by digital pH meter. Similarly, the lactose contents and protein were estimated with titration.[Citation11]
Fat and free fatty acids
Milk fat % was determined by Gerber method by using the butyrometer[Citation11] and free fatty acids were determined by following the method number Ca 5a-40 of the American Oil Chemist Society.[Citation12]
Sensory analysis
In this study to monitor sensory changes during storage of milk, we adopt the method of descriptive analysis. In this method, the sensory panel comprised a team of ten members. Five out of them were males and five were females with age range from 25 to 35 years among postgraduate students and laboratory staff who were trained for sensory evaluation. Samples for testing were coded and served at room temperature. For this purpose, samples kept at 4°C, 7°C, and 10°C were withdrawn from storage and allowed to equilibrate at room temperature 1 h before serving. The panelists were asked to evaluate the sensory attributes of aroma, flavor, and overall appreciation by assigning a liking score on a 9-point hedonic scale as described by Meilgaard et al.[Citation13]
Selection and reaction of nanoparticles
Commercially available four different types of nanoparticles were selected in this study like silicon oxides, titanium oxides, aluminum oxides, and clay. These particles were reacted with 250 μl of standard aldehydes, alcohols, and ketones of Sigma-Aldrich Inc. It was observed whether these particles reacted with the standard compounds or not and showed some color variation.
Selection of reagents
Two commonly used reagents for the detection of aldehydes and ketones, 2,4-dinitrophenylehydrazine and Schiff’s reagent, were selected to observe the reaction at 4°C, 7°C, and 10°C storage temperatures.
Coating of nanoparticles with reagent
Ten grams of each type of nanoparticles were taken in four different petri dishes and 5 ml of reagent was added in it and mixed very well and smoothly. Then these petri dishes were incubated at 37°C for 4 days to evaporate the moisture and absorbed the reagent in coated form and it became dry. The color at the time of adding the reagent in the nanoparticles and after incubation was noted. Then these reagent coated-materials will again be converted into nanoparticles by ultrasonic apparatus.
Reaction of Schiff reagent-coated nanoparticles
Two 50 ml glass filter flasks were taken and connected each other with rubber pipe. Their upward opening was stopped with rubber cork. In one flask, 250 μl mixture of measured and calculated amount of aldehyde, ketones, and alcohols that can spoil the milk according to sensory in the experiment No. 2 was taken. In other flask, the 20 mg of coated reagent was taken and kept the flask inverted on the table so that the volatile compounds evaporated from the other flask can react properly with nanoparticles present. The aldehyde and ketones evaporated and through the rubber pipe went to the next flask and reacted with the nanoparticles coated with reagent and the change in color of the coated nanoparticles was observed. The nanoparticle which has shown prominent color would be selected for milk experiment.
Trial with milk
The main and ultimate object of this study was the observation of change in color of coated nanoparticles with off-flavoring compounds (aldehydes and ketones) produced during storage period of milk that are responsible for the spoilage of milk. So, the selected nanoparticles coated with reagent were used for pasteurized milk at different refrigeration temperatures.
Procurement and preparation of samples
Commercially available fresh pasteurized milk (2 gallons) of Darigold, Inc. Seattle, Washington 98119, USA, was procured from Wallmart Superstore. Then 200 ml milk was transferred under the sterilized conditions into 250-ml sterilized conical flasks. The flask was sealed with rubber cork with an airtight assembly of glass. A small glass tube inserted in rubber cork was fitted inside the milk flask. The upper end of the glass tube was contained with a filter paper just at the top of the cork. Through this tube, the volatile compounds were evaporated and reacted with the 200 μg of nanoparticles spread at the filter paper. A total of 30 samples were prepared and stored for 19 days. After preparing the apparatus and material, the flasks with airtight assembly of glass were kept at 4°C, 7°C, and 10°C of storage temperature.
Storage conditions
The samples were stored at 4°C, 7°C, and 10°C for 19 days with the time interval of 0, 1, 5, 9, 12, 14, 16, 17, 18, and 19 days. The samples were subjected to further analysis.
Measurement of color
The color was measured with the colorimeter by using the system CIE-LAB color space.[Citation11]
Sensory analysis
Sensory stability of the samples was estimated based on sensory results of a trained panel which consisted of ten members. The panelists was asked to evaluate the sensory attributes of aroma, flavor, and overall appreciation by assigning a liking score on a 9-point hedonic by Meilgaard et al.[Citation13]
Statistical analysis
This experimental design was a complete randomized design and data obtained were analyzed through two-way analysis of variance technique.[Citation14] The separation of means was made through Duncan’s Multiple Range Test. The whole analysis was carried out with the help of Mini-tab software package.
Results and discussions
Physicochemical parameters
The mean values of pH at all temperatures on 0 day were 6.81 and on 19th day, were 6.00, 5.45,and 4.36 at 4°C, 7°C, and 10°C, respectively. The values of acidity increased from 0.09% to 0.108%, 0.115%, and 0.126% at 4°C, 7°C, and 10°C which was 35°C at 10°C, 21°C (). Vyletelova et al.[Citation15] concluded that pH decreases and acidity increases by production of lactic acid and free fatty acids as a result of enzymatic hydrolysis of protein and fats during storage period and different temperatures. The results of present investigation are in line with the previous finding of Kuippers et al.[Citation16]
Table 1. Effect of time and temperature on the mean values of pH, acidity, and FFA of pasteurized milk
The mean values () of free fatty acids were increased up to 41.60, 43.80, and 48.50 from 35.40 mg/L at 0th day, but on the other hand the fat contents () were decreased to 2.82, 2.44 and 2.22 at 4°C, 7°C, and 10°C respectively at 19th day of storage. It is obvious from the results that fat decreases by production of free fatty acids as a result of its enzymatic hydrolysis during storage period and different temperatures. These enzymes are produced by the thermoduric psychotrophs or may be by the heat stable enzymes during pasteurization of milk. The present investigations are in line with the previous findings of Nagla et al.[Citation17] The results of present investigation are similar with the previous finding of Ali et al.[Citation18] Lactose and protein contents were less affected by storage time and temperature. The lactose contents decreased from 4.6% to 4.37%, 4.23%, and 4.06% and protein from 3.40% to 3.15%, 2.803%, and 2.56% at 4°C, 7°C, and 10°C respectively (). The results are similar with the findings and trends of Ali et al.[Citation18] These results are in close agreement with the previous findings of Burdova et al.[Citation19]
Table 2. Effect of time and temperature on the means values of fat, protein, and lactose of pasteurized milk
The protein decreases by production of amino acids as a result of its proteolytic hydrolysis during storage period and different temperatures. These proteases enzymes are produced by the thermoduric psychotrophs[Citation20] or may be by the heat stable enzymes during pasteurization of milk. The lipases are responsible for the enzymatic breakdown of fat and for the increase of quantity of free fatty acids. The lipolytic enzymes that were produced by the thermoduric psychotrophs during storage indicate that lactose decreases by production of lactic acids as a result of its enzymatic hydrolysis during storage period and different temperatures.[Citation21]
Sensory evaluation
The mean scores of aroma at 0th day at all temperatures were 8.8 and at 19th day were 5.67, 5.43, and 4.50 at 4°C, 7°C, and 10°C. The aroma of fresh pasteurized milk was clean and pleasant at 0th day storage at all temperatures (). According to the panelist score, it decreases as the time intervals were increased and similarly as the temperature increases it was also decreased and maximum decrease was observed at 10°C on the 19th day of storage. The aroma is related to volatile compounds so it is affected with production of different aldehydes, ketones, and alcohols by the breakdown of fatty acids and enzymatic fermentation of lactose.[Citation1] The results of present investigation are in line with the previous finding of Ziarno et al.[Citation22]
Table 3. Effect of time and temperature on the means score of aroma, taste, and OAA of pasteurized milk
The taste of the pasteurized milk was significantly influenced by storage period and temperature. According to table, the mean score at 0 day was 8.8 which decreased to 6, 5, and 4.17 at 4°C, 7°C, and 10°C (). The taste was affected with production of different compounds like fatty acids,[Citation23] aldehyde, ketones, and alcohols by enzymatic fermentation of fat, protein, and lactose.[Citation24] showed similar trends of overall acceptability of pasteurized milk. The score decreased from 9 to 6, 5.17, and 4.00 at 4°C, 7°C, and 10°C, respectively which were 33%, 42%, and 54% decrease in overall acceptability, respectively. The overall acceptability is the combination of appearance, aroma, and taste and it was clean and pleasant of fresh pasteurized milk at 0th day storage at all temperatures. Craven and Macauley[Citation1] reported that the overall acceptability was affected with production of different compounds like fatty acids, aldehyde, ketones, and alcohols by enzymatic fermentation of fat, protein, and lactose. The results are in close agreement with the results of Petrus et al.[Citation25]
Second phase
According to initial trial on the reaction and selection of nanoparticles, the particles did not react with the standard compounds and did not show any color variation. By using 2,4-dinitrophenylehydrazine and Schiff’s reagents, the Schiff’s reagents showed good reaction at refrigeration temperature. Among the coated nanoparticles with Schiff’s reagents reaction, following colors appeared as silicon oxides showed light pink, titanium oxides showed gray, aluminum oxides showed reddish, and clay showed chocolate (). After the reaction of these coated particles with aldehydes and ketones, following colors were shown by particles as silicon oxides turned from pink to black, titanium oxides turned from gray light to dark sky blue, aluminum oxides did not change the reddish, and clay showed light milky chocolate (). So the silicon oxide was selected for further analysis on pasteurized milk study. Silica nanoparticles SiO2 were typically 5–100 nm and were available in ultra high purity with a high specific surface area in the 25–50 m2/g range ().
Color produced by volatiles with the reaction of silicon oxide nanoparticles
The volatiles produced by psychrotrophic bacteria of pasteurized milk stored at different temperatures reacted with the coated silica nanoparticles resulting in their color at relatively low detection levels. The results of colorimeter were expressed as LAB that represented the color as the decrease or increase in L*values indicated the dullness or brightness of pink color, a* falls between (a, -a) red and green and b* between (b, -b) yellow and blue. The L* values was non-significantly changed or decreased with mean values of 64.82 ± 0.08 to 64.13 ± 0.07 at 4°C and significantly with 64.81 ± 0.03 to 63.87 ± 0.07 and 64.77 ± 0.02 to 61.43 ± 0.05 at 7°C and 10°C respectively at 0–19th day of milk storage (). During storage, the pasteurized milk stored at all temperatures 4°C, 7°C, and 10°C showed that a* value non-significantly decreased from 48.69 ± 0.18 to 48.63 ± 0.18, 48.74 ± 0.14 to 48.57 ± 0.11, and 48.68 ± 0.18 to 47.97 ± 0.07 respectively. Similarly, the values of b* decreased non-significantly at 4°C and 7°C with the values of −17.56 ± 0.23 to −18.58 and −17.54 ± 0.25 to −19.85 ± 0.85 but significantly decreased at 10°C from −17.56 ± 0.23 to −43.22 ± 0.11 (). The original color of silicon dioxide-treated nanoparticles was light pink at 0 day of storage. The decrease in L* values indicated the dullness or brightness of pink color. The results indicated that it slightly decreased at 10°C. It means that pink color dullness increased or brightness decreased. But there was no change or decrease in brightness of nanoparticles color at 4°C and 7°C. The values of a* showed that no significant change was observed in red color toward green color at all temperatures and all storage days but a negligible change was observed at 10°C at 19th day. The main change was observed in the values of b*. The results showed that the color of nanoparticles turned form pink to dark blue or black. This was due to significant decrease in b* values at 7°Cand 10°C.
Table 4. Comparison of mean values of nanoparticles color (L*, a*, and b*) produced by volatiles of pasteurized milk stored at different temperatures for 19 days
Sensory evaluation
The statistical analysis given in showed that the aroma of the pasteurized milk was significantly influenced by the storage period and temperature. The mean scores at 0th day at all temperatures were 9.00 ± 0.00 and at 19th day were 5.67 ± 0.44, 5.43 ± 0.17, and 4.33 ± 0.17 at 4°C, 7°C, and 10°C. The aroma of fresh pasteurized milk was clean and pleasant at 0th day storage at all temperatures. According to the panelist score, it decreases as the time intervals were increased and similarly as the temperature increases it was also decreased and maximum decrease was observed at 10°C on the 19th day of storage. The taste of the pasteurized milk was significantly influenced by storage period and temperature (). The mean score at 0th day was 9.00 ± 0.00 which decreased to 6.00 ± 0.29, 5.00 ± 0.00, and 4.00 ± 0.00 at 4°C, 7°C, and 10°C. The maximum decrease was observed at 10°C on the 19th day of storage. Table 5 showed similar trends of overall acceptability of pasteurized milk. The score decreased from 9.00 ± 0.00 to 6.00 ± 0.00, 5.17 ± 0.17, and 4.00 ± 0.00 at 4°C, 7°C, and 10°C. The overall acceptability is the combination of appearance, aroma, and taste and it was clean and pleasant of fresh pasteurized milk at 0th day storage at all temperatures. According to the score of panelist, it decreased as the time intervals and temperatures increased and maximum decrease was observed at 10°C on the 19th day of storage. All the sensory parameters of pasteurized milk like aroma, taste, and overall acceptability were affected with production of different compounds like fatty acids, aldehyde, ketones, and alcohols by enzymatic fermentation of fat, protein, and lactose. The results are in close agreement with the results of Petrus et al.[Citation25] and Ziarno et al.[Citation22]
Table 5. Comparison of mean scores of aroma, taste, and overall acceptability (OAA) of pasteurized milk stored at different temperatures for 19 days
Correlation of sensory parameters with color change of nanoparticles for spoilage
When we correlated the sensory results with color change of nanoparticles with storage time and temperature, it was observed that there was negligible change in L* from 64.810 ± 0.08 to 64.13 ± 0.07 with 6.00 ± 0.00 acceptable sensory score of aroma, taste, and overall acceptability out of 9.00 at 4°C from 0th to the 19th day of storage (). Whereas the reasonable decrease in L* reading from 64.81 ± 0.04 to 63.87 ± 0.07 and 61.43 ± 0.05 was viewed at 7°C and 10°C which correlated with unacceptable sensory scores (5.17 ± 0.17, 5.00 ± 0.00, and 5.17 ± 0.17 and 4.33 ± 0.17, 4.00 ± 0.00, and 4.00 ± 0.00) respectively of aroma, taste, and overall acceptability at 19th day of storage ( and ). When we correlated the sensory parameters with the color indicator a*, it expressed the almost similar trends as mentioned in case of L*. The combine effect of storage time and temperature on color variation of a* was nonsignificant (P > .05). However, the sensory scores were dropped in the same decreasing trends as mentioned for L* for all temperatures (–). The maximum decrease or change in color of nanoparticles was observed at b*. It decreased from −17.56 ± 0.23 to −43.00 ± 0.11 at 10°C at the end of storage days (–) and this was highly correlated with the indication of milk spoilage with unacceptable limit of sensory scores that were 4.33 ± 0.17, 4.00 ± 0.00, and 4.00 for aroma, taste, and overall acceptability. The color of nanoparticles changed very minute at 4°C but significantly at 7°C that was compatible with the sensory scores of panelist (). It was also observed that color of nanoparticles was significantly changed from pink to dark blue from 14th day of storage onwards to the end at 10°C (). This is highly correlated with the spoilage trends indicated by sensory panel with sensory scores (score 4.00).
Figure 4. Relationship of sensory scores with color variation of L* for spoilage detection of pasteurized milk stored at 4°C
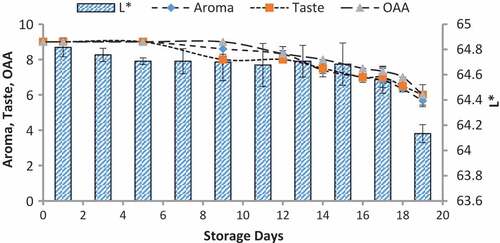
Figure 5. Relationship of sensory scores with color variation of L* for spoilage detection of pasteurized milk stored at 7°C
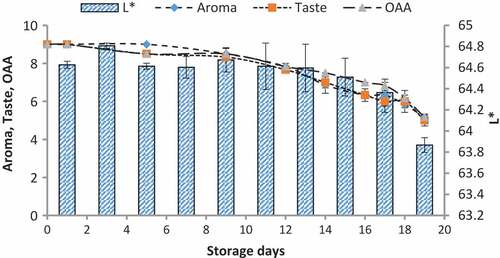
Figure 6. Relationship of sensory scores with color variation of L* for spoilage detection of pasteurized milk stored at 10°C
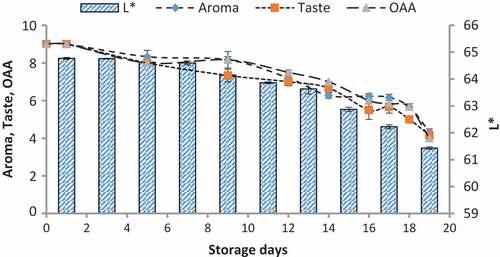
Figure 7. Relationship of sensory scores with color variation of a* for spoilage detection of pasteurized milk stored at 4°C
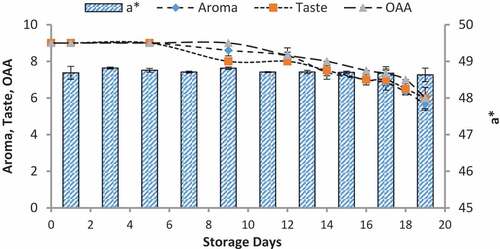
Figure 9. Relationship of sensory scores with color variation of a* for spoilage detection of pasteurized milk stored at 10°C
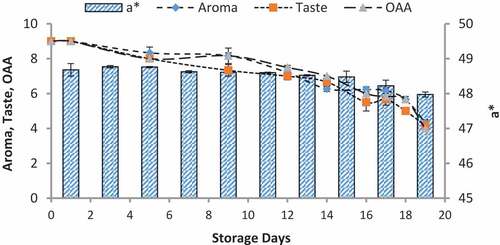
Conclusion
The physicochemical parameters of pasteurized milk were significantly affected by the temperature and time. During post-pasteurization cold storage, pH, fat, protein, and lactose contents decreased while the rates of Free Fatty Acids (FFA) and acidity increased significantly at 10°C as compared to 7°C and 4°C at the 19th day. Our research has shown that at 4°C the change in color of nanoparticles by the reaction with volatiles is negligible even at 19th day of milk storage. While at 10°C a distinct change was observed at 14th day to 19th day of storage which indicates that milk is going to spoil according to sensory scores. Coated silica nanoparticles have a reactive surface that binds Schiff’s reagent and readily interacts with food spoilage compounds including aldehydes and alcohols resulting in color changes at relatively low detection levels. So, silica nanoparticles can be used for the monitoring the shelf life of pasteurized milk by the colorimetric detection of volatiles. Moreover, this study can further be extended to develop a SiO2-based nano-sensor to detect volatile compounds produced by psychrotrophic bacteria during milk spoilage at refrigeration temperatures. It is recommended for the fluid milk industry that pasteurized milk should be stored at 4°C carefully; otherwise, a small increase in temperature can alter the shelf life of recommended storage days.
Acknowledgments
The authors thank to the management team of Wallmart Superstore, Pullman, Seattle, USA who provided zero day processed freshly pasteurized milk of Darigold, Inc. They greatly acknowledge Professor Barbara Rasco and Assistant Professor ShaymeSablani (School of Food Sciences, Washington State University, USA) for their valuable suggestions and providing them laboratory facility.
Additional information
Funding
References
- Craven, H.-M.; Macauley, B.-J. Microorganisms in Pasteurized Milk after Refrigerated Storage. Aust. J. Dairy Technol. 1992, 47(1), 38–45.
- Zadow, J.-G.;. Extending the Shelf Life of Dairy Products. Food Aust. 1989, 41(9), 935–937.
- Douglas, S.-A.; Gray, M.-J.; Crandall, A.-D.; Boor, K.-J. Characterization of Chocolate Milk Spoilage Patterns. J. Food Prot. 2000, 63(4), 516–521.
- Rankin, S.-A.;. Liquid Milk Products/Super-Pasteurized Milk. Encyclopedia of Dairy Science; Academic Press: Amsterdam, 2002; Vol. 3; pp 1633–1637.
- Meunier-Goddik, L.; Sandra, S. Liquid Milk Products/Pasteurized Milk. Encyclopedia of Dairy Sciences; Academic Press: Amsterdam, 2002; Vol. 3; pp 1627–1632.
- Fromm, H.-I.; Boor, K.-J. Characterization of Pasteurized Fluid Milk Shelf-Life Attributes. J. Food Sci. 2004, 69(8), 207–214. DOI: 10.1111/j.1365-2621.2004.tb09889.x.
- Cromie, S.-J.;. Microbiological Aspects of Extended Shelf Life Products. Aust. J. Dairy Technol. 1991, 46(2), 101–104.
- Qureshi, A.; Roci, I.; Gurbuz, Y.; Niazi, J.-H. An Aptamer Based Competition Assay for Protein Detection Using CNT Activated Gold-Interdigitated Capacitor Arrays. Biosens. Bioelectron. 2012, 34, 165–170. DOI: 10.1016/j.bios.2012.01.038.
- Nesli, S.; Jozef, L.-K. Nanotechnology and Its Applications in the Food Sector. Trends Biotechnol. 2009, 27(2), 10–16.
- Landaverde, P.-A.; Velazquez, G.; Torres, J.-A.; Qian, M.-C. Quantitative Determination of Thermally Derived Off-Flavor Compounds in Milk Using Solid-Phase Microextraction and Gas Chromatography. J. Dairy Sci. 2005, 88, 3764–3772. DOI: 10.3168/jds.S0022-0302(05)73062-9.
- AOAC. (1990). Official methods of analysis, 17th ed. Washington DC: Assoc. of Official Analytical Chemists.
- AOCS. Official Methods and Recommended Practices of the American Oil Chemists’ Society, 4th ed.; Edited by D. Firestone Champaign. American Oil Chemists’ Society: USA, 2004.
- Meilgaard, M.-C.; Civille, G.-V.; Carr, B.-T. Sensory Evaluation Techniques, 4th ed.; CRC PRESS: Boca Raton, FL, USA, 2007.
- Steel, R.-G.; Torrie, J.-H.; Dickey, D.-A. Principles and Procedures of Statistics. A Biometrical Approach, 3rd ed.; Mc-Graw Hill Book Co: New York, 1997.
- Vyletelova, M.; Hanovs, O.; Urbanova, E.; Kopunecz, P. Theoccurance and Identification of Psychrotrophic Bacteria with Proteolytic and Lipolytic Activity in Bulk Milk Samples at Storage in Primary Production Conditions. Czech J. Anim. Sci. 2000, 45, 373–383.
- Kuippers, O.-P.; Buist, G.; Kok, J. Current Strategies for Improving Food Bacteria. Res. Micro. 2000, 151, 815–822. DOI: 10.1016/S0923-2508(00)01147-5.
- Nagla, B.; Mohamed, O.; Abdel, A. Microbiological Quality of Heat-Treated Milk during Storage. Pak. J. Nutr. 2009, 8(12), 1845–1848. DOI: 10.3923/pjn.2009.1845.1848.
- Elmagli, A. A.-O.; Ibtisam, E.-M. Study on the Composition of Pasteurized Milk in Khartoum State (Sudan). Int. J. Dairy Sci. 2006, 1(1), 12–20. DOI: 10.3923/ijds.2006.12.20.
- Burdova, O.-L.; Maria, B.; Andrea, L.; Hanna, R.; Jolanta, G. Hygiene of Pasteurized Milk Depending on Psychrotrophic Microorganisms. Bull. Vet. Inst. Pulawy. 2002, 46, 325–329.
- Sùrhaug, T.; Stepaniak, L. Psychrotrophs and Their Enzymes in Milk and Dairy Products: Quality Aspects. Review. Trends Food Sci. Technol. 1997, 8(2), 35–41. DOI: 10.1016/S0924-2244(97)01006-6.
- Santos, M.-V.; Ma, Y.; Caplan, Z.; Barbano, D.-M. Sensory Threshold of Off-Flavors Caused by the Proteolysis and Lipolysis in Milk. Int. J. Dairy Sci. 2003, 86, 1601–1607. DOI: 10.3168/jds.S0022-0302(03)73745-X.
- Ziarno, M.; Molska, I.; Gronczynska, M. Quality and Shelf Life of Milk Pasteurized and Free from Post Pasteurization Contamination. MedycynaWeterynaryina. 2005, 61, 1165–116.
- Guy, E.-J.; Hicks, K.-B.; Flanagan, J.-F.; Foglia, T.-A.; Holsinger, V.-H. Effect of Storage of Raw and Pasteurized Goats’ Milk on Flavor Acceptability, Psychrotrophic Bacterial Count, and Content of Organic Acids. J. Food Prot. 1985, 48(2), 122–129. DOI: 10.4315/0362-028X-48.2.122.
- Ng, S.-Y.; Improved Shelf Life and Quality of Liquid Milk Products. Master of Appl Sci Thesis, RMIT Melbourne, 1991.
- Petrus, R.; Loiola, C.; Silva, C.; Oliveira, C. Microbiological and Sensory Stability of Pasteurized Milk in Brazil. Bull. Vet. Inst. Pulawy. 2005, 42(3), 125–129.