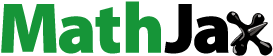
ABSTRACT
Kudzu starch has been used as a natural healthy food worldwide. Low solubility, poor water dispensability, high paste viscosity and low fluidity limit its wholesome utility. In order to improve its processing properties and applicability, kudzu starch has been modified using a branching enzyme (BE) from Rhodothermus obamensis and in combination with maltogenic amylase (MA) from Bacillus stearothermophilus. The results indicated that amylose content and molecular weight decreased with enzyme treatment but the proportion of short chains and branch density increased. The natural A-starch type crystalline structure changes to V-type but with decreased crystallinity. The storage modulus and loss modulus depended on the enzyme treatment time. Overall, enzymatic modification results in starch fractions with increased solubility and paste transparency, reduced gelatinization temperature, gelatinization enthalpy, and viscosity. The outcome opens up new opportunities to develop more palatable and marketable products based on kudzu starch.
Introduction
Kudzu (Pueraria lobata) is an herbal plant utilized in traditional Chinese medicine for generations. It has several bioactive isoflavones (16.2%, w/w) such as daidzein, daidzin and puerarin with anti-cancer properties in addition to treating influenza, fever, dysentery, hypertension, and angina pectoris.[Citation1–Citation4] Among the other nutrients, starch is an important component and accounts for about 15–34% of fresh kudzu root.[Citation5] Kudzu starch is natural and is not processed as of corn and potato starches. It possesses a small quantity of isoflavonoids with neutral flavor and smooth paste texture and is being used as a functional food.[Citation6,Citation7] However, its large-scale utility is limited due to poor solubility and dispersal, high paste viscosity and bad fluidity, to name a few.
Figure 2. HPAEC diagram of amylopectins from natural (a) and enzyme-modified kudzu starches of (b) BE; MA→BE (different BE time): (c) 1 h; (d) 3 h; (e) 6 h; (f) 9 h; (g) 12 h
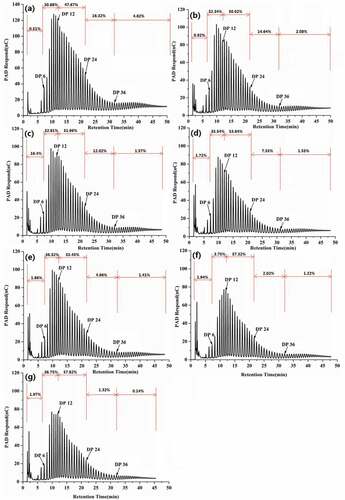
Kudzu starch contains around 21% amylose and the remainder amylopectin. The molecular weight of amylopectin is of the order 107–109 with an average chain length of 20.5.[Citation8,Citation9] The ratio of short/long chains ranges from 3:1 to 2:1.[Citation10] The internal and external parts of amylopectin form the amorphous lamellae and crystalline region in the starch granule.[Citation11] Its chain length distribution and internal structure have significant effect on the starch solubility, swelling, gelatinization, pasting and retrogradation. In general, the external chains can be classified as A, B, and C chains, wherein A-chains do not carry any other chains, B-chains hold one or more chains, and the C-chain contains the reducing end. The B-chains could be further classified into B1, B2, B3 and B4 depending on the number of clusters they take part: B1 chains participate in one cluster while B2 and B3 chains link two and three clusters, respectively, whereas B4 chains extend to four or more clusters. These external chains contribute to molecular entanglement leading to higher shear resistance. The low swelling power of starches is attributed to low amount of A chains, high percentage of B1 (DP 13–24) and B2 (DP 25–36) chains.[Citation12] In addition, high swelling and solubility arise from low molecular weight of amylose along with an incompact granule structure.[Citation13] The starch swelling further is precluded by amylose and amylopectin interactions.[Citation14] The amount of B3-chains (DP > 36) positively correlates to the gelatinization temperature and enthalpy, whereas A chains (DP 6–12) and B1 chains negatively correlate.[Citation11,Citation15] Amylopectin with Bfp (DP 3–7) and B1b (DP 18–24) chains shows lower viscosity of starch paste, whereas amylopectin with B3 chains exhibits higher viscosity.[Citation11] The presence of increased α-1, 6 linkages retards the starch retrogradation, and reduces peak viscosity and setback value, as well as increase solubility and gelatinization enthalpy.[Citation16,Citation17] A higher branching degree and shorter branching chain length lowers the starch retrogradation.[Citation18] Thus, it would be more effective to change the amylopectin internal molecular structure of kudzu starch to gain the desired functionality toward well-rounded industrial applications.
Branching enzyme (BE, EC 2.4.1.18) has been used to alter the branch chain length and branch density of starches.[Citation19] The BE the α-1, 4-glucosidic bonds, and then creates new α-1, 6-glucosidic bonds via transglycosylation reaction on the cleaved glucan chains, and hence produces new branched glucans and amylopectin clusters.[Citation19] On the other hand, Maltogenic α-amylase (MA) from Bacillus stearothermophilus (EC 3.2.1.133) is an endo-acting enzyme that internally hydrolyses α-1, 4-glycosidic linkages and displays high transglycosylation activity through the formation of α-1, 6-glucosidic bonds and α-1, 3-glucosidic bonds, which results in branched α-glucans with lower molecular weight.[Citation20,Citation21] In this regard, a combined reaction of BE and MA could be effective to modify the molecular structure of kudzu starch along with increasing α-1, 6 linkages and shorten the amylopectin chain lengths. Herein, kudzu starch has been subjected to[Citation1] BE alone and[Citation2] combination of MA→BE treatments, and the outcome provides a theoretical basis to modulate the physicochemical properties of kudzu starch toward developing novel functional foods.
Materials and methods
Materials
Kudzu starch was from Geye Starch (Anhui, China). Maltogenic amylase (MA) from Bacillus stearothermophilus (MA, ≥400 U/mg), isoamylase (160,000 U m/L) from Pseudomonas amyloderamosa and pullulanase from Bacillus subtilis (≥ 1000 U m/L) were purchased from Sigma-Aldrich (St. Louis, Missouri, USA). BE from Rhodothermus obamensis (4900 U/mL) was gift from Novozymes (Denmark).
Enzyme treatment
Kudzu starch (10 g) was dispersed in 200 mL of 0.02 mol/L sodium acetate buffer (pH 6.4). The dispersion was then treated with BE (300 U/g dry weight of starch). The reaction was conducted at 50°C for 1 h to gain BE-modified starch. Later 1 mol/L NaOH solution of 2.5 mL was added to stop the reaction. MA-treated starches were prepared by treating the starch dispersion with MA (10 U/g dry weight of starch) at 50°C for 7 h, stirring constantly, and the reaction was stopped with 1 mol/L NaOH solution of 2.5 mL. The 0.02 mol/L sodium acetate buffer was used to adjust the enzymatic hydrolysate to pH 6.4, and the MA-treated starch dispersion was hydrolyzed with BE (300 U/g dry weight of starch). The BE reaction was conducted at 50°C for time intervals of 1, 3, 6, 9, and 12 h to prepare MA/BE-modified starches. The enzymatic hydrolysates were dialyzed using dialysis bags with molecular weight cut off 3500 Dalton in deionized water to remove low molecular sugars and salt ions. Three volumes of anhydrous ethanol was added to hydrolysates to precipitate starch. The precipitate was then centrifuged at 8000 rpm for 5 min and then washed with purified water. The enzyme-modified starches were freeze-dried and crushed for further characterization.
Scanning electron microscopy
A S-4800 Hitachi scanning electron microscope (Hitachi High-Technologies Corporation, Japan) was used to image the starch granules. Starch sample was placed onto the surface of double-faced carbon rubberized fabric set on an aluminum strip, and then thin gold film of 20 nm covered the whole sample in an argon atmosphere.
Structure characteristics
Amylose level was measured following the literature reported protocol.[Citation22] Briefly, the starch (0.1 g) was blended with 1 mL absolute ethyl alcohol and 9 mL sodium hydroxide solution (0.8 mol/L). The mixed dispersion was heated in boiling water bath for 25 min, and then diluted with purified water to 80 mL. Subsequently, 1 mL of acetic acid (1 mol/L) and 2 mL of iodine solution (0.02% I2 and 0.2% KI) were added into 5 mL mixed solution and stirred uniformly. The purified water was added into the mixed solution to dilute to 100 mL, mixed, and then set for 30 min. Amylose level was calculated using a standard curve.
Molecular mass was measured according to a previous approach.[Citation23] Briefly, the 1 mL starch solution was filtered and then transfused to a high-performance size-exclusion chromatography (HPSEC) integrated with multi-angle laser light scattering (MALLS) and a differential refractive index (RI). Shodex OH-Pak 804 and 806 columns were connected in tandem and equilibrated at 25 °C and then 200 μl sample was injected. The weight average molecular weight (MW) was calculated using ASTRA V4.09.07 (Wyatt Technology) with the Berry extrapolation for curve fitting with the dn/dc value of 0.148 mL/g. The 100 mmol/L NaNO3 aqueous solution was used as a moving phase and then filtered with a membrane filter (0.2 μm) and degassed. The flow velocity was set to 0.2 mL/min.
Amylopectin was extracted and purified from the starch sample.[Citation24] The chain length distribution of amylopectin was analyzed following our previous method.[Citation25] The debranching amylopectin was measured using a high-performance anion-exchange chromatography (HPAEC) equipped with pulsed amperometric detection (PAD). The CarboPac PA-100 analytical column (4 × 250 mm) together with a CarboPac PA-100 guard column (4 × 50 mm) was adopted. The hydrolysate was washed at 0.3 mL/min with a gradient of the mixture of eluent B (1.0 mol/L sodium acetate) and eluent A (0.5 mol/L sodium hydroxide) as follows: 0–17 min, 15–38%; 17–28 min, 38–42%; 28–55 min, 42–50%; 55–58 min, 50–100%; 58–60 min, 100–15%; and 60–80 min, 15%.
Determination of the ratio of α-1, 6-glycosidic linkage
Proton nuclear magnetic resonance (1HNMR) analyses were carried out by using the proton nuclear magnetic resonance spectroscopy (DD2 600 MHz, Agilent, USA). The 3 mg sample was dispersed in 2 mL of deuterium oxide (D2O) and stirred for 2.5 h in boiling water bath, and then lyophilized. The D2O-dissolved sample was redissolved in 1 mL D2O. The levels of α-1, 4-glycosidic linkage and α-1, 6-glycosidic linkage were quantified from the peaks at 5.4 and 5.0 ppm, respectively. The ratio of α-1, 6-glycosidic linkage was determined through dividing the peak area corresponding to α-1, 6-glycosidic linkage by the total peak area corresponding to α-1, 4-glycosidic linkage and α-1, 6-glycosidic linkage.[Citation26]
Starch crystallinity
The starch crystallinity was measured using a Bruker D8 Discover A25 X-ray diffractometer equipped with a copper tube operating at 40 kV and 40 mA with Cu Kα radiation of a 0.154 nm wavelength. The scanning region was set from 5 to 40° of (2θ) at a rate of 2°/min and a step size of 0.05°. The relative crystallinity (RC, %) of each starch samples was estimated by dividing the crystalline peak area by the total area of the X-ray diffractogram using the Jade 7.0 software.
Solubility and paste transparency
The solubility of the starch sample was determined following a previous method.[Citation27] Briefly, 0.3 g sample was blended with the 25 mL of pure water in a centrifuge tube. It was vortexted for 20 min and then heated in a boiling water bath for 0.5 h. The gelatinized starch paste was then cooled to 25 °C, and centrifuged at 10000 g for 10 min. The supernatant was dehydrated at 110 °C to constant weight. The percent transmittance (%T) of starch pastes was measured at 640 nm. The solubility was calculated using the following equation:
Thermal analysis
The thermal analysis was based on a reported method.[Citation28] Briefly, gelatinization temperatures and gelatinization enthalpy (ΔH) were measured by using the DSC8000 Thermal Lab System (PerkinElmer, USA). Gelatinization temperature range (Tc-To) was calculated. Starch of 4 mg (dry weight basis) was placed into an aluminum pan and the moisture content was adjusted to 10% by adding deionized water. The pan was sealed and sample was equilibrated for 24 h at 25°C. The sample was scanned from 5 to 90°C at a heating rate of 10°C min−1.
Rheological properties
The rheological properties were carried out according to our established protocol.[Citation29] The 10% starch dispersion was gelatinized with a magnetic stirrer for 60 min and then cooled to 30°C. The DHR-1 rotational rheometer (TA Instruments, New Castle, USA) was utilized with a cone plate of 40 mm at a 0.06 mm gap and a cone angle of 2°. The apparent viscosity was measured as a function of shear rate in the range 0 to 300 s−1. The elastic modulus (G′), the viscous modulus (G″), loss tangent (Tan δ) and complex viscosity (η*) were measured as a function of angular frequency (ω) in the range 0.1 to 100 rad/s at 2% strain, in the linear viscoelastic region.
Statistical analysis
The results were presented as the means ± standard deviations from triplicate measurements. The Variance analysis was adopted to analyze the significance in differences at a 0.05 level of confidence.
Results and discussion
Morphological characteristics
The photomicrograph of all starch samples is displayed in . The natural kudzu starch granules display spherical, polygonal and irregular shapes of different sizes but with smooth and nonporous surfaces ()). The outermost layer has been peeled off non-uniformly after the BE treatment ()). In addition, pores are noticed on the granules. Thus, it appears that BE attacks from outside-in on starch granules presumably due to smooth granular surface that precludes enzyme channeling into the interiors of granules. Interestingly, BE in combination with MA appears to be beneficial to accelerate the starch hydrolysis and the hydrolysis degree depends on the BE treatment time. Pore size enlarges along with broken granule fragments as the BE treatment time increases from 1 to 12 h (–)). Several micropores along with tiny cracks are noticed on the granules with the BE treatment time of less than 6 h (–)). Deep pores with variable sizes are noticed, however, on starch granules with prolonged treatments (≥ 9 h, ,)) along with bigger cracks and honeycomb granules suggesting that granules are getting hydrolyzed. Overall, combination of MA and BE appears to be effective in synergistically attacking the exterior and interior portions of kudzu starch granules.
Chain length distributions
The chain length distributions of amylopectin are generally categorized into four parts based on the degree of polymerization (DP): A-chains with DP 6–12, B1-short chains with DP 13–24, B1-long chains with DP 25–36 and B2 chains with DP ≥ 37.[Citation30] In the present case, samples exhibit lower proportions of shorter chains (0.81–1.97%) with DP less than 6 (). The BE treatment enhances the A and B1 chains by 4.73% and 4.93%, respectively, compared to the natural starch, however, B2 and B3 chains reduce by 10.29% and 54.98%, respectively. The MA→BE modification gradually boosts the percentages of A and B1 chains but with substantial decreases in the B2 and B3 chains For example, at 12 h of BE treatment, A and B1 chains enhance by 25.49% and 22.06%, respectively but B2 and B3 chains decline by 91.91% and 97.84%, respectively. It appears that during the enzymatic treatment, amylose or the external side chains of amylopectin are getting cleaved resulting in shorter linear chains, and these shorter linear chains are then transferred to branch chains, resulting in increased percentage of A and B1 chains along with decreased proportions of B2 and B3 chains.
Table 1. Molecular structure and hygroscopic characteristics of natural and enzyme-modified starches
Table 2. Gelatinization properties of natural and enzyme-modified starch granules
Branched density
The 1HNMR spectra and the ratio of α-1, 6-glucosidic bonds are presented in . The peaks at 3.3–4.5 ppm originate from H-2, H-3, H-4, and H-5 protons and the 4.8 ppm is from the H2O protons. The peak at 5.4 ppm represents α-1, 4-glucosidic bonds, and the 5.0 ppm corresponds to α-1, 6-glucosidic bonds.[Citation31] The ratio of α-1, 6-glucosidic bonds in BE-modified starch increases to 7.2% compared to 6.4% of natural starch. In combination with MA, the ratios of α-1, 6-glucosidic bonds of MA/BE-modified starches increase remarkably from 8.3 to 13.4% with increasing the BE time. These results indicate clearly the cleavage of α-1, 4-glucosidic bonds with the MA treatment and formation of new α-1, 6-glucosidic bonds with the BE.
Crystalline properties
The X-ray diffraction patterns of all starch samples are highlighted in . In the natural kudzu starch, the typical 15.1° peak coupled with the two unresolved peaks at 17.2° and 18.2°, and a broad peak at 23.5° suggest the typical A-starch structure. The relative crystallinity is found to be 23.9%. After enzyme treatment, these peaks disappear barring the 17.2° that further strengthens with the BE treatment time. In addition, three new peaks at 7.6°, 13.1° and 19.7° are noticed, which also show intensity increase with the BE treatment time. These new peaks correspond to the V-type starch structure. Thus, the initial A-type kudzu starch structure transforms to V-starch structure after enzyme treatment. This finding further corroborates the formation of more short linear glucans and is in congruence with the results observed in the chain length distributions and branch density sections. As is shown in , all enzyme treatments result in the chain length reduction. The chain length of BE-modified starch decreases from 19.30 to 17.65 compared to that of the natural starch. The chain lengths further continue to decline with the MA→BE treatment. The chain lengths decrease from 14.32 to 5.68 as BE incubation time increases from 1 to 12 h. Thus, after dual enzyme modification, amylopectin may recrystallize spontaneously as chain length becomes shorter with enhanced branch density in the amorphous lamellae, as well as the distance between branching points decreases, forming the V-type starch structure.[Citation30,Citation32] It can also be seen from that compared to the natural starch, enzyme-modified starches exhibit lower crystallinity suggesting that more short branch chains are produced by the MA and BE treatment that are not prone to form crystalline aggregates leading to reduced crystallinity. The BE treatment time influences the relative crystallinity and increases from 15.8 to 19.5% is noticed for 1 to 12 h of treatment. The initial decrease could be due to formation of higher amount of short linear chains with MA but as the BE modification results in V-type crystals but with prolonged BE treatment the short linear chains complex with anhydrous ethanol and thus increase the overall crystallinity.
Solubility and paste transparency
The solubility and paste transparency of all starch samples have been listed in . The natural starch has lower solubility (43.2%) and paste transparency (50.6%). On the other hand, enzyme modified starches display higher solubility of 64.5–77.9% and paste transparence of 58.6–79.4%. This is due to the fact that increasing the branch points in amylopectin ruptures the highly ordered structure of starch and prevents glucan chains from realigning. As is indicated in , in comparison with natural starch, the amylose decreases by 7% after the BE treatment. The amylose content reduces after MA→BE treatment, especially decreases from 17.3 to 11.8% as BE incubation time increases from 1 to 12 h. Thus the reduction of amylose content is significantly influenced by the extent of BE treatment. Molecular mass of enzyme-modified starches also reduces significantly (). In comparison with natural starch (6.8 × 108 g/mol), the molecular weight of BE-treated starch decreases to 3.6 × 108 g/mol. After the MA→BE treatment, the molecular mass further declines to 2.1 × 108 with 1 h BE incubation and finally drops to 0.4 × 108 g/mol at 12 h of treatment. Thus it appears that the molecular mass of kudzu starch is largely influenced by the BE incubation time. The reduction of molecular mass could be due to the synergistic action of MA and BE on the longer linear chains of amylose in generating shorter linear chains and/or branched linkages.[Citation33] Moreover, smaller clusters with limited molecular distribution could form through hydrolyzing the internal α-1, 4-jointed linear chains between the amylopectin clusters.[Citation34] Overall, low amylose levels, decreased molecular weight, high proportion of short chains, and branching degree as a result of enzyme treatment result in increased starch solubility.
Thermodynamic properties
The gelatinization temperatures of native kudzu starch range from 68.5 to 81.2°C (). The enzyme-modification results in lower temperature range of 66.2 to 77.8°C presumably due to reduction in longer chains after enzyme treatment leading to higher content of shorter double helices. In addition, not only the α-1, 6 glycosidic bonds produced decrease the heat resistance of starch granules, but also the disruption of α-1, 4 glycosidic bonds. The culminating effect results in the gelatinization temperature.[Citation11]
The melting temperature range (Tc-To) reflects variations in the crystalline orientation in the starch granules.[Citation35] As compared to the native starch, narrower melting range in BE- or MA/BE-treated starches suggests the presence of ordered chain structures and hence forming homogeneous crystalline texture during enzyme catalysis. The shortened branch chains produced by BE and MA/BE modification contribute to weaker and less stabilized crystalline texture.[Citation36] The difference of Tc-To could as well result in the formation of crystallites and double helices with variable stabilities. The reduction in the enthalpy change (ΔH) of enzyme-treated starches () further suggests presence of small amount of double helices or weak interactions between molecular chains in the crystalline zone as a result of enzyme treatment.[Citation37,Citation38]
Rheological behavior
The steady rheological behavior of 10% starch pastes at 30oC is depicted in . The apparent viscosities of all starch samples decrease as shear rate enhances (), suggesting kudzu starch suspension is a pseudoplastic fluid. All samples exhibit the shear thinning behavior. Native starch displays significantly higher viscosity compared to the enzyme-modified starches. This could be due to decrease in amylose content after dual enzyme modification, causing reduction in the entanglements between the amylose chains.[Citation39] Besides, BE or MA/BE treatment reduces interactions between molecular chains due to larger degree of branching, which in-turn decreases possible binding domains in the starch paste network leading to a rise in the shear thinning ability.[Citation40] also shows that apparent viscosities of enzyme-modified starches gradually decline as BE treatment time increases. This may be because that the molecular chains of starch transfer from random entanglement to a direction arrangement due to higher proportion of short linear chains. Such a process reduces the interstitial space in the network and associated entanglement points resulting in a larger reduction of viscosity.[Citation41] Also, increase of branch points in starch breaks the highly ordered structure and prevents realignment of linear chains after the BE treatment and could as well continue to the decrease in the apparent viscosity.[Citation42,Citation43] highlights that shear stress augments as shear rate enhances. Native starch presents a remarkably higher shear stress than all the enzyme-treated starches. It further indicates that all starch pastes follow the non-Newtonian fluid behavior with a pseudo-plastic property. The pseudo-plastic behavior enhances with the BE treatment time. On the other hand, shear stress of all enzyme-treated starches gradually decreases with increasing the BE treatment time.
Figure 5. Apparent viscosity versus shear rate of native (a) and enzyme-modified kudzu starches of (b) BE; MA→BE (different BE time): (c) 1 h; (d) 3 h; (e) 6 h; (f) 9 h; (g) 12 h
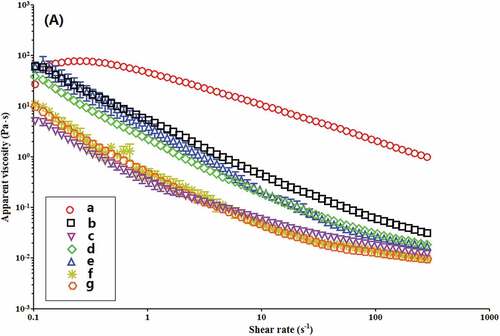
The elastic modulus (G′) and the viscous modulus (G″) of 10% starch pastes as a function of the angular frequency (ω) in the range 0.1 to 100 rad/s at 30 oC are shown in . The G′ and G″ show the frequency dependency. In all the samples, G′ is higher than G″ portraying a solid-like behavior.[Citation44] In the BE-treated starches, G′ and G″ are greater than the natural starch. In comparison with the native starch, these two moduli of enzyme-treated starches are generally higher when the BE treatment time is 1 h, presumably due to chain rearrangement. They are similar to that of natural starch when the BE treatment time is 3 h. However, lower values are observed for 6 to 12 h of the BE treatment mainly due to the short branched chain lengths and low molecular weights. It is known that amylose allows the gelation of starch and increasing its concentration augments the solid behavior. The cleavage of short linear chains can decrease the gel strength leading to a liquid like behavior when the time of BE treatment is equal to or higher than 6 h. These results demonstrate that the elastic characteristics of enzyme-treated starches depend on the extent of BE modification.
Figure 6. The elastic modulus (G′) and the viscous modulus (G″) as a function of angular frequency (ω) of native (a) and enzyme-modified kudzu starches of (b) BE; MA→BE (different BE time): (c) 1 h; (d) 3 h; (e) 6 h; (f) 9 h; (g) 12 h
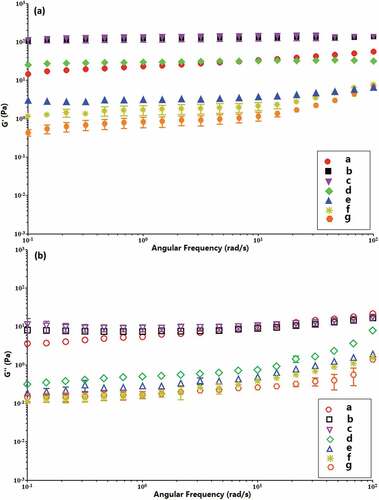
Tan δ (G″/G′) has been used to elucidate the rheological change of starch pastes. In general, elastic behavior will be noticed when tan δ < 1 and viscous nature for tan δ is larger than 1.[Citation45] shows that tan δ values of all the samples are less than 1, implying the elastic behavior exceeds the viscous behavior. Generally, tan δ values of all starch samples are nearly constant at low frequencies, but raise with the frequency. The tan δ of the enzyme-modified starches are larger than the native starch after the BE treatment or the combination of MA and BE (BE treatment time = 1 h), which is associated with larger resilience and more rigid texture of starch pastes. However, they are higher than the native starch after MA→BE modification when BE incubation time ranges from 6 to 12 h. Thus, it appears that larger viscous and smaller elastic behaviors occur after double enzymatic treatment.
Figure 7. The loss tangent (Tan δ) and complex viscosity (η*) as a function of angular frequency (ω) of native (a) and enzyme-modified kudzu starches of (b) BE; MA→BE (different BE time): (c) 1 h; (d) 3 h; (e) 6 h; (f) 9 h; (g) 12 h
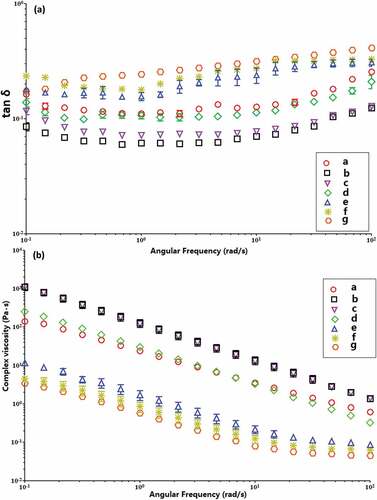
shows complex viscosity η* as a function of ω for all starch samples. The η* value of native starch decreases linearly with increasing frequency. Also, the η* values of enzyme-modified starches at BE treatment time ≤ 3 h are parallel to each other and decline linearly with an increase in the frequency, which implies the frequency dependence of η* of the enzyme-modified starches. However, for the magnitudes of η* of enzyme-modified starches at BE treatment time ≥ 6 h, they are parallel to each other and decrease linearly at lower frequency, whereas they are almost constant at higher frequency, indicating a weak frequency dependence of η*. These results suggest that the elastic property of starch pastes decrease at larger frequencies, which is mainly due to decreasing rate of chain rearrangement.
Conclusions
The molecular structures and functional characteristics of kudzu starch are influenced significantly with the BE enzyme treatment and its combination with the MA. Molecular weight and amylose content decrease with an increase in the branch density and number of short chains. The modification also induces structural changes and the initial A-type starch structure transforms to V-type coupled with reduced overall relative crystallinity. The resulting starches are highly water-soluble and yield low viscosity solutions, and further depend on the BE treatment time. Overall, this study provides an efficient, elegant and “green process” of regulating physicochemical properties of kudzu starch toward developing novel functional foods, such as instant kudzu powder, kudzu powder drink, etc., but further research is warranted.
Acknowledgments
This research has been supported by National Natural Science Foundation of China (Grant No. 31771933), International Cooperation Foundation of Qilu University of Technology (Shandong Academy of Sciences) (Grant No. QLUTGJUZ014), Special Funds for Taishan Scholars Project, Independent Training Innovation Team project (Grant No. 2018GXRC004) and the USDA National Institute for Food and Agriculture (HATCH project SD00H648-18).
We confirm that there are no conflicts of interest associated with this publication.
Additional information
Funding
References
- Hirakura, K.; Morita, M.; Nakajima, K.; Sugama, K.; Takagi, K.; Nitsu, K.; Ikeya, Y.; Maruno, M.; Okada, M. Phenolic Glucosides from the Root of Pueraria Lobata. Phytochemistry. 1997, 46, 921–928. DOI: 10.1016/S0031-9422(97)00371-3.
- Keung, W. M.; Vallee, B. L. Kudzu Root: Anancient Chinese Source of Modern Antidipsotropic Agents. Phytochemistry. 1998, 47, 499–506.
- Shurtleff, W.; Aoyagi, A. The Ancient Genie. In The Book of Kudzu: Aculinary and Healing Guide; Shurtleff, W., Aoyagi, A., Eds.; Autumn press: Massachusetts, 1977; pp 8–18.
- Sibao, C.; Dajian, Y.; Shilin, C.; Hongxi, X.; Chan, A. S. Seasonal Variations in the Isoflavonoids of Radix Puerariae. Phytochem. Anal. 2007, 18(3), 245–250. DOI: 10.1002/pca.978.
- Soni, P. L.; Agarwal, A. The Starch of Pueraria Tuberose Comparison with Maize Starch. Starch. 1983, 35, 4–7. DOI: 10.1002/star.19830350103.
- Van Hung, P.; Morita, N. Chemical Compositions, fine Structure and Physicochemical Properties of Kudzu (Puerarialobata) Starches from Different Regions. Food Chem. 2007, 105(2), 749–755. DOI: 10.1016/j.foodchem.2007.01.023.
- Geng, Z.; Zongdao, C.; Yimin, W. Physicochemical Properties of Lotus (Nelumbo Nucifera Gaertn.) And Kudzu (Pueraria Hirsute Matsum.) Starches. Int. J. Food Sci. Tech. 2007, 42, 1449–1455. DOI: 10.1111/j.1365-2621.2006.01363.x.
- Aberle, T.; Burchard, W.; Vorwerg, W.; Radosta, S. Conformational Contributions of Amylose and Amylopectin to the Structural Properties of Starches from Various Sources. Starch. 1994, 46(9), 329–335. DOI: 10.1002/star.19940460903.
- Suzuki, A.; Hizukuri, S.; Takeda, Y. Physicochemical Studies of Kudzu Starch. Cereal Chem. 1981, 58, 286–290.
- Hizukuri, S.;. Relationship between the Distribution of the Chain Length of Amylopectin and the Crystalline Structure of Starch Granules. Carbohyd. Res. 1985, 141, 295–306. DOI: 10.1016/S0008-6215(00)90461-0.
- Zhu, F.;. Relationships between Amylopectin Internal Molecular Structure and Physicochemical Properties of Starch. Trends Food Sci.Tech. 2018, 78, 234–242. DOI: 10.1016/j.tifs.2018.05.024.
- Chung, H. J.; Liu, Q.; Donner, E.; Hoover, R.; Warkentin, T. D.; Vandenberg, B. Composition, Molecular Structure, Properties, and in Vitro Digestibility of Starches from Newly Released Canadian Pulse Cultivars. Cereal Chem. 2008, 85, 471–479. DOI: 10.1094/CCHEM-85-4-0471.
- Wani, I. A.; Sogi, D. S.; Hamdani, A. M.; Gani, A.; Bhat, N. A.; Shah, A. Isolation, Composition, and Physicochemical Properties of Starch from Legumes: A Review. Starch. 2016, 68, 834–845. DOI: 10.1002/star.201600007.
- Srichuwong, S.; Jane, J. L. Physicochemical Properties of Starch Affected by Molecular Composition and Structures: A Review. Food Sci. Biotechnol. 2007, 16, 663–674.
- Kong, X. L.; Bertoft, E.; Bao, J. S.; Corke, H. Molecular Structure of Amylopectin from Amaranth Starch and Its Effect on Physicochemical Properties. Int. J. Biol. Macromol. 2008, 43, 377–382. DOI: 10.1016/j.ijbiomac.2008.07.018.
- Lee, C. K.; Le, Q. T.; Kim, Y. H.; Shim, J. H.; Lee, S. J.; Park, J. H.; Lee, K. P.; Song, S. H.; Auh, J. H.; Lee, S. J.; et al. Enzymatic Synthesis and Properties of Highly Branched Rice Starch Amylose and Amylopectin Cluster. J. Agr. Food Chem. 2008, 56, 126–131. DOI: 10.1021/jf072508s.
- Ren, J. Y.; Li, Y.; Li, C. M.; Gu, Z. B.; Cheng, L.; Hong, Y.; Li, Z. F. Pasting and Thermal Properties of Waxy Corn Starch Modified by 1, 4-α-glucan Branching Enzyme. Int. J. Biol. Macromol. 2017, 97, 679–687. DOI: 10.1016/j.ijbiomac.2017.01.087.
- Singh, H.; Lin, J.-H.; Huang, W.-H.; Chang, Y.-H. Influence of Amylopectin Structure on Rheological and Retrogradation Properties of Waxy Rice Starches. J. Cereal Sci.. 2012, 56, 367–373. DOI: 10.1016/j.jcs.2012.04.007.
- Van der Maarel, M. J. E. C.; Leemhuis, H. Starch Modification with Microbial Alpha-Glucanotransferase Enzymes. Carbohyd. Polym.. 2013, 93, 116–121. DOI: 10.1016/j.carbpol.2012.01.065.
- Kim, J. W.; Kim, Y. H.; Lee, H. S.; Yang, S. J.; Kim, Y. W.; Lee, M. H.; Kim, J. W.; Seo, N. S.; Park, C. S.; Park, K. H. Molecular Cloning and Biochemical Characterization of the First Archaeal Maltogenic Amylase from the Hyperthermophilic Archaeon Thermoplasma Volcanium GSS1. Biochim. Biophys. Acta Proteins Proteom. 2007, 661–669. doi:10.1016/j.bbapap.2007.03.010.
- Park, K. H.; Kim, T. J.; Cheong, T. K.; Kim, J. W.; Oh, B. H.; Svensson, B. Structure, Specificity and Function of Cyclomaltodextrinase, a Multispecific Enzyme of the α-amylase Family. BBA. 2000, 1478, 165–185.
- Biselli, C.; Cavalluzzo, D.; Perrini, R.; Gianinetti, A.; Bagnaresi, P.; Urso, S.; Orasen, G.; Desiderio, F.; Lupotto, E.; Cattivelli, L.; et al. Improvement of Marker-Based Predictability of Apparent Amylose Content in Japonica Rice through GBSSI Allele Mining. Rice. 2014, 7, 1–18. DOI: 10.1186/1939-8433-7-1.
- Jo, A. R.; Kim, H. R.; Choi, S. J.; Lee, J. S.; Chung, N. M.; Han, S. K.; Park, C.-S.; Moon, T. W. Preparation of Slowly Digestible Sweet Potato Daeyumi Starch by Dual Enzyme Modification. Carbohyd. Polym. 2016, 143, 164–171. DOI: 10.1016/j.carbpol.2016.02.021.
- Guo, L.; Li, H.; Cui, B. Enzymatic Hydrolysis of Amylopectins from Lotus Rhizome and Kudzu Starches. J. Food Nutr. Res. 2018, 57, 396–407.
- Guo, L.;. In Vitro Amylase Hydrolysis of Amylopectins from Cereal Starches Based on Molecular Sturcture of Amylopectins. Food Hydrocolloid. 2018, 77, 238–247. DOI: 10.1016/j.foodhyd.2017.09.039.
- Lee, B. H.; Yan, L.; Phillips, R. J.; Reuhs, B. L.; Jones, K.; Rose, D. R.; Nichols, B. L.; Quezada-Calvillo, R.; Yoo, S. H.; Hamaker, B. R. Enzyme-Synthesized Highly Branched Maltodextrins Have Slow Glucose Generation at the Mucosal Alpha-Glucosidase Level and are Slowly Digestible in Vivo. Plos One. 2013, 8(4), 1–10.
- Leach, H. W.; McCowen, L. D.; Schoch, T. Structure of the Starch Granule. II. Swelling Power and Solubility Patterns of Various Starches. J. Cereal Chem. 1959, 36, 534–544.
- Guo, L.; Cui, B. The Relationship between Entanglement Concentration and Physicochemical Properties of Potato and Sweet Potato Starch Dispersions. Int. J. Food Sci. Tech. 2018, 53, 337–346. DOI: 10.1111/ijfs.13590.
- Guo, L.; Hu, J.; Zhang, J. J.; Du, X. F. The Role of Entanglement Concentration on the Hydrodynamic Properties of Potato and Sweet Potato Starches. Int. J. Biol. Macromol. 2016, 93, 1–8. DOI: 10.1016/j.ijbiomac.2016.08.075.
- Hanashiro, I.; Tagawa, M.; Shibahara, S.; Iwata, K.; Takeda, Y. Examination of Molar-Based Distribution of A, B and C Chains of Amylopectin by Fluorescent Labeling with 2-Aminopyridine. Carbohyd. Res. 2002, 337, 1211–1215. DOI: 10.1016/S0008-6215(02)00110-6.
- Hernández, J. M.; Gaborieau, M.; Castignolles, P.; Gidley, M. J.; Myers, A. M.; Gilbert, R. G. Mechanistic Investigation of a Starch-Branching Enzyme Using Hydrodynamic Volume SEC Analysis. Biomacromolecules. 2008, 9, 954–965. DOI: 10.1021/bm701213p.
- Planchot, V.; Gérard, C.; Bertoft, E.; Colonna, P. An approach to structure analysis of granules using genetically modified starches. In Starch: Advances in Structure and Function; Barsby, T. L., Donald, A. M., Frazier, P. J., Eds.; Royal Society of Chemistry: Cambridge, UK, 2001; p 103–106.
- Jensen, S. L.; Zhu, F.; Vamadevan, V.; Bertoft, E.; Seetharaman, K.; Bandsholmb, O.; Blennow, A. Stabilization of Semi-Solid-State Starch by Branching Enzyme-Assisted Chain-Transfer Catalysis at Extreme Substrate Concentration. Carbohyd. Polym. 2013, 98, 1490–1496. DOI: 10.1016/j.carbpol.2013.07.071.
- Kittisuban, P.; Lee, B.-H.; Suphantharika, M.; Hamaker, B. R. Slow Glucose Release Property of Enzyme-Synthesized Highly Branched Maltodextrins Differs among Starch Sources. Carbohyd. Polym. 2014, 107, 182–191. DOI: 10.1016/j.carbpol.2014.02.033.
- Le, Q.-T.; Lee, C.-K.; Kim, Y.-W.; Lee, S.-J.; Zhang, R.; Withers, S. G.; Kim, Y.-R.; Auh, J.-H.; Park, K.-H. Amylolytically-Resistant Tapioca Starch Modified by Combined Treatment of Branching Enzyme and Maltogenic Amylase. Carbohyd. Polym. 2009, 75, 9–14. DOI: 10.1016/j.carbpol.2008.06.001.
- Tester, R. F.; Morrison, W. R. Swelling and Gelatinization of Cereal Starches. I. Effects of Amylopectin, Amylose, and Lipids. Cereal Chem. 1990, 67, 551–557.
- Lopez-Rubio, A.; Flanagan, B. M.; Gilbert, E. P.; Gidley, M. J. A Novel Approach for Calculating Starch Crystallinity and Its Correlation with Double Helix Content: A Combined XRD and NMR Study. Biopolymers. 2008, 89, 761–768. DOI: 10.1002/bip.21005.
- Cooke, D.; Gidley, M. J. Loss of Crystalline and Molecular Order during Starch Gelatinisation: Origin of the Enthalpic Transition. Carbohyd. Res. 1992, 227, 103–112. DOI: 10.1016/0008-6215(92)85063-6.
- Ai, Y.; Jane, J. L. Gelatinization and Rheological Properties of Starch. Starch. 2015, 67, 213–224. DOI: 10.1002/star.201400201.
- Li, W. W.; Li, C. M.; Gu, Z. B.; Qiu, Y. J.; Cheng, L.; Hong, Y.; Li, Z. F. Relationship between Structure and Retrogradation Properties of Corn Starch Treated with 1, 4-α-glucan Branching Enzyme. Food Hydrocolloid. 2016, 52, 868–875. DOI: 10.1016/j.foodhyd.2015.09.009.
- Tsai, M. L.; Li, C. F.; Lii, C. Y. Effects of Granular Structures on the Pasting Behaviors of Starches. Cereal Chem. 1997, 74, 750–757. DOI: 10.1094/CCHEM.1997.74.6.750.
- Donth, E.; Beiner, M.; Reissig, S.; Korus, J.; Garwe, F.; Vieweg, S. Fine Structure of the Main Transition in Amorphous Polymers: Entanglement Spacing and Characteristic Length of the Glass Transition: Discussion of Examples. Macromolecules. 1996, 29, 6589–6600. DOI: 10.1021/ma951881a.
- Liu, P. F.; Kang, X. M.; Cui, B.; Wang, R.; Wu, Z. Z. Effects of Glycerides with Different Molecular Structures on the Properties of Maize Starch and Its Film Forming Capacity. Ind. Crop. Prod. 2019, 129, 512–517. DOI: 10.1016/j.indcrop.2018.12.039.
- Yuan, C.; Sang, L. Y.; Wang, Y. L.; Cui, B. Influence of Cyclodextrins on the Gel Properties of Kappa-Carrageenan. Food Chem. 2018, 266, 545–550. DOI: 10.1016/j.foodchem.2018.06.060.
- Tung, M. A.; Paulson, A. T. Rheological Concepts for Probing Ingredient Interactions in Food System. In Ingredient Interactions: Effect on Food Quality; Gaonkar, A. G., Ed.; Marcel Dekker: New York, 1995; pp 45–83.