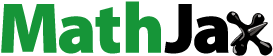
ABSTRACT
The gel properties and structural changes of sweet potato starch induced by heat-moisture treatment (HMT) were investigated, and its vermicelli textural properties were revealed. HMT increased the relative crystallinity, and the crystallinity reduced with increasing moisture content, which was consistent with the results of DSC. RVA results revealed that the HMT starches showed an increased setback viscosity and a reduced breakdown viscosity, indicating that the HMT starches gel lead a stronger retrogradation tendency. Rheometer results indicated that the HMT starches had a stronger elasticity behavior. The content of amylose of starch and the possible amylose fine molecular change during HMT at different water content were hypothesized, and this caused the gel properties of starch. Consequently, the HMT starch vermicelli exhibited higher hardness, springiness, and chewiness. These mechanistic relations among starch structure, gel properties, and vermicelli quality during HMT will provide useful information for the starch vermicelli industry.
Introduction
Sweet potato is the seventh most important food crop and is recognized as the primary starch-producing crop worldwide. Sweet potato is rich in carbohydrates, vitamins, and fiber, and it can be eaten raw or cooked, unripe or ripe. The main product of sweet potato processing is starch, which is used in the production of traditional foods, such as vermicelli. The starch functional properties of pasting, texture, retrogradation, and rheology are critical factors in determining the end use and market demand for sweet potato starch and flour. Native starch exhibits poor properties, including low solubility, high viscosity of starch gel, and poor thermal shear, which limit its use in the industry.[Citation1] Thus, physical and chemical modifications must be implemented to improve the functional properties and produce starch with special properties.
Heat moisture treatment (HMT) is a physical method that uses simple, environmentally safe processes with low cost and without by-products of chemical reagents, making it superior to chemical modification. For this reason, HMT starch can be safely used in various foods. Many researchers reported that HMT can change the structural and physicochemical properties of starch, including its pasting properties, retrogradation properties, and starch granule crystallinity.[Citation2–Citation5] However, the change trends vary depending on different starch species and HMT conditions. Moisture content and botanical sources are the determining factors of HMT condition, although moisture content is the only determining external reaction factor that has been challenged.[Citation6,Citation7] Few studies have explored the effect of HMT on the structural and physicochemical characteristics of sweet potato starch and its vermicelli quality under different moisture contents. Therefore, this study investigated this research gap to assess the feasibility of HMT in improving the vermicelli quality of sweet potato starch.
Materials and methods
Materials
Sweet potato starch (water content, 12.6%; protein, 0.38%; fat, 0.60%; and amylose content, 30.5%) was provided by Hunan Xiang Feng Potato Industry, China.
Heat-moisture treatment of starch sample
The modification method was conducted in accordance with Sun, Q., Nan, C.[Citation8] The exact moisture content of sweet potato starch must be measured using a moisture analyzer (MA45, Sartorius AG, Goettingen, Germany) before the moisture content of sweet potato starch can be adjusted. The moisture content of sweet potato starch was adjusted to 26%, 30%, and 34%, and the samples were sealed and stored at room temperature for equilibration for 24 h. Then, the samples were placed in a covered Petri dish (D = 12 mm) and heated in a dry oven at 105°C for 1 h. After being cooled to room temperature, the samples were ground by a mechanical grinder. The powdered starch was sieved through a 100-mesh sieve and then stored in airtight plastic bags at 4°C until use.
X-ray diffraction and relative crystallinity
X-ray diffraction tests were conducted on the samples by using an X-ray diffractometer (Modl D5005, Bruker, Kawlsruhe, Germany) under the following conditions: X-ray tube Cu-Ka nickel filter of 40 kv, voltage of 100 mA, scanning 2θ range of 5–40°, and continuous scan.
The relative crystallinity was calculated by Jade6.0 according to EquationEquation (1)(1)
(1) .
where X is the area of the crystalline region, and Y is the area of the amorphous and crystalline regions.
Pasting properties
The pasting properties of all starch samples were examined by using a Rapid Viscosity Analyzer (RVA, Model 4D, Newport Scientific, Australia). Starch samples (3.0 g, 14 g/100 g moisture basis) were weighed directly in the RVA canister. The samples were equilibrated at 50°C for 1 min, heated to 95°C for 4 min, held at 95°C for 5 min before cooling to 50°C, and then held at 50°C for 4 min. The mixture was stirred at 960 rpm for 10 s and then at 160 rpm for the remainder of the test. All tests were replicated thrice. The values measured from the pasting profile were peak viscosity (PV), hot paste viscosity (HPV), final viscosity (FV), breakdown (BD), setback (SB), and pasting temperature (PT).
Thermal properties
The thermal properties of the starch samples were measured using a differential scanning calorimeter (DSC 200 F3 Maia Netzsch-Geratebau GmbH, Germany) in accordance with the method of Zebin Guo et al.[Citation9] In this study, 3.0 mg of starch (dry basis) was weighed in an aluminum DSC pan, and 10 μL of distilled water was added. The pan was sealed, equilibrated for 1 h at room temperature, and heated from 25°C to 120°C at 10°C/min. An empty stainless steel pan was used as the reference, and all measurements were performed in triplicates. Netzch Proteus 6 was used to calculate the onset temperature (To), peak temperature (Tp), conclusion temperature (Tc), and enthalpy of gelatinization (∆H).
Rheological properties
The rheological properties of the HMT sweet potato starch sample were determined using a rotational rheometer (Physical MCR301, Anton Paar GmbH Stuttgart, Germany). The gelatinized starch was placed in the determination platform of the instrument, which was equipped with a parallel plate with a diameter of 40 mm and a gap of 1.0 mm. The rheological properties of steady flow and dynamic viscoelasticity were determined thrice for each sample.
Steady flow measurement
Steady flow tests were performed with the shear rate varying between 1 and 500 s−1 at 25°C with a tableted geometry of 40 mm diameter. The Herschel–Bulkley rheological model was used to fit the experimental data of the flow curve according to EquationEquation (2)(2)
(2) .
where σ (Pa) is the shear stress, σ0(Pa) is the yield stress, γ (s−1) is the shear rate, K (Pa·sn) is the consistency coefficient, and n is the flow behavior index.
Dynamic viscoelastic measurements
In dynamic viscoelastic measurements, the storage modulus (G’) and the loss modulus (G’’) were evaluated by running frequency sweeps in the range of 0.1–10 rad/s at a constant strain (1%), which was within the linear region. The loss tangent tan δ was obtained according to EquationEquation (3)(3)
(3) .
Creep measurement
Creep tests were performed by applying a constant stress of 1 Pa and maintaining the duration of 250 s. The creep compliance was calculated as the ratio of the total strain at a given time to the constant stress. Each test was performed in triplicate.
Vermicelli preparation
Vermicelli was prepared from 28 g of starch, and the blends were formulated in accordance with the method proposed by Liao L, Wu W (2017) with slight modifications.[Citation10] A portion of 3 g of the starch sample was mixed with 20 mL of hot water under constant stirring to completely gelatinize the starch and obtain a slurry. Then, 25 g of the remaining sample was added to attain a total slurry concentration of approximately 45%. The slurry was poured into an acrylic plate of 20 cm diameter, spread to form a sheet thickness of approximately 1.0 mm, and equilibrated for 5 min. Then, the slurry was steamed at 92.5°C for 4 min to complete the gelatinization and then dipped into cold water for 1 min to cool to room temperature. The noodle sheet was left to stand for 30 min (retrograde) at room temperature before being scraped and cut into 5 mm strips and dried in a convectional dryer at 40°C until the final moisture content was 12%.
Textural analysis of vermicelli
The cooked noodles were kept in a covered Petri dish, and textural analysis was performed within 15 min by using a texture analyzer (TA/XT2i, Stable MicroSystems, Surrey, UK). Texture profile analysis was conducted as described by Qingjie Sun et al.[Citation11] with slight modifications. A strand of cooked noodle with 1.0 mm thickness was compressed by a cylinder probe (P/36) until the deformation reached 75% at a speed of 1.0 mm/s. The interval between the first and second compressions was 0.5 s. The textural parameters, including hardness (HD, g) (height of the peak), springiness (ratio of the distance recorded during the second compression to that recorded during the first compression), and chewiness (CH, g× mm) (gumminess× springiness), were determined from the force–time curve of the texture profile. Ten measurements were made for each replication for a total of 30 readings recorded for each sample.
Statistical analysis
The experiments were conducted in replicates, and data were expressed as the mean with the standard deviation obtained using Duncan’s multiple range test (p < .05) in SPSS20 software.
Results and discussion
X-ray diffraction and relative crystallinity
The X-ray diffraction pattern and relative crystallinity of the native and HMT sweet potato starches at 22%, 26%, 30%, and 34% moisture contents for 1 h are illustrated in . As shown, HMT did not change the inherent diffraction pattern of native starch. All the modified starch samples exhibited higher relative crystallinity (15.52%, 15.12%, 14.86% and 14.73% for moisture content 22%, 26%, 30%,34%,respectively) than native starch sample (14.58%), which might be due to further enhanced associations between starch chains, and the structure rearrangement of the disrupted double helices that led to an increase in crystal perfection or the new crystallite formation of HMT.[Citation12] Moreover, the formation of new crystallite also could induce a reduction in swelling power, which is desirable in the manufacture of vermicelli. The increased relative crystallinity was consistent with the previous results observed for HMT starches from sweet potato starch,[Citation13] potato starch,[Citation14] and mung bean starch.[Citation15] The relative crystallinity of modified starch samples was decreased from15.52% for moisture content of 22% to 14.73% for moisture content of 34%. The decrease relative crystallinity samples following the increasing moisture content might be due to partial gelatinization of the starch granules resulting disruption of amylopectin crystallinity during HMT. Furthermore, water could promote the disruption of starch granule intra and intermolecular hydrogen bonding, which could induce a reduce regularity of the molecular packing.[Citation16] These results indicated that the changes in the X-ray relative crystallinity of the HMT starches are influenced by moisture content, which is consistent with the previous findings of Hoover, R and Vasanthan, T (1994) and Tattiyakul et al. (2006).[Citation17,Citation18]
Pasting properties
The pasting parameters including the pasting temperature (PT), peak viscosity (PV), hot paste viscosity (HPV), breakdown (BD), and setback (SB) of native and HMT samples treated at 22%, 26%, 30% and 34% moisture contents, respectively, are summarized in . As shown in , compared with that of native sample, the PT of the HMT samples increased from 79.25°C to 85.96°C as the starch moisture content increased, confirming that the starch structure disintegrated and that the paste formation required more heat because of the changes in the structure and reorientation of starch granules, molecules, or chains. After HMT, PV and BD decreased from 5227 to 2764 cp to 4771 and 652 cp, respectively, compared with that of the native sample. These results agreed with previous findings.[Citation19–Citation21] They claimed that structural rearrangement about the extent of starch chains associations in the amorphous regions, and the degree of crystalline order contributed to these changes. The reduction in BD caused by HMT showed that the modified starches were more resistant to the influence of shear and heat conditions than native starch, which was consistent with previous findings.[Citation22,Citation23] However, as the moisture level of the starch samples increased after HMT, the values of HPV, FV, and SB increased significantly (p < .05) from 2464, 3220, and 756 cp to 4119, 6386, and 2267 cp, respectively, which contradicted the results obtained by Chung, H J, Liu, Q, & Hoover, R and Lan, H, et al.[Citation24,Citation25]Meanwhile, these reports have been identified that the reduction of amylose content plays a role in the decrease of the setback viscosity. During the cooling, the amylose of the starch paste retrogrades and viscosity increases to the final value; thus, the amylose content also affects the final viscosity. Therefore, FV and SB reflected the retrogradation tendency of amylose and the gelling ability. The information of apparent viscosity curves is primarily provided about the paste temperatures, the heating viscosity, the resistance of the swollen granules about mechanical action, as well as the effect on cooling of viscosity (retrogradation). The linear structural of amylose molecules are mainly responsible for the occurrence of retrogradation. This increase in setback values (SB) of HMT samples was due to the increscent in the amount of amylose leached resulting in stronger short-term retrogradation during treatment. Furthermore, the more moisture content there was, the amylose content increased. A higher retrogradation tendency of the starch samples indicated its suitability in the application of jelly foods and vermicelli. Lii, C Y, and Chang, S M reported that a starch paste with a high setback value on cooling can produce good-quality vermicelli.[Citation26] These results suggest that HMT is an effective method for changing the pasting properties of sweet potato starch for the production of starch vermicelli.
Table 1. RVA pasting parameters of native starch and the heat moisture-treated sweet potato starch at different water levels
Thermal properties
The thermal characteristic parameters and statistical analysis results samples were summarized in . Heat moisture treatment altered the thermal properties markedly, with its effect being highly dependent on the moisture content during treatment. The onset, peak, and conclusion temperatures (To, Tp, and Tc) of the gelatinization of samples increased from69.10, 79.04, 86.87 (native starch) to74.78, 86.27, 90.53 (HMT treated with 34% moisture content) respectively. The more moisture content there was, the more increased was the gelatinization temperatures. This remarkable increscent was attributed to the partial gelatinization and disruption of the starch granules, the disrupted molecules rearrangement and the increasing of the starch chains associations simultaneously, which caused the perfection of double helical order increasing and decrease the content of the double helical order. Furthermore, the more the perfection helical double order there was, the higher gelatinization temperatures there was needed. A decrease was shown in the gelatinization temperature range (∆T), which was similar to the reports by Ahn. et al. and Ting-Ting Huang.et.al.[Citation27,Citation28] The result was likely contributed to increscent of the starch chains associations and the starch granules internal bonds strengthened. In this study, the gelatinization enthalpy (∆H) of the HMT samples was higher than the native samples. Heat moisture treatment was influenced to some extent by the starch source, the amylose chain length, and the moisture content prevailing during treatment.[Citation16] Silverio J et al. reported that the extent of increase ∆H has been shown to the influenced by the level of phosphate groups located on the inner and outer sections of the B-chains of amylopectin.[Citation29] This increase maybe was due to the dislocations in the amylopectin clusters induced by the reorientation of the sweet potato starch phosphate groups during HMT. However, the more moisture content there was, the more reduced was the ∆H () and the relatively crystallinity () of HMT samples. These results were likely due to the greater flexibility and mobility of starch chains for rearrangement at higher moisture content.
Table 2. DSC thermal characteristics of native starch and the heat-moisture treated sweet potato starch at different water levels
Rheological properties
The state flow behaviors of native and HMT samples treated at different moisture content were illustrated in . The flow curves for the shear stress and shear rate of the native and HMT starches were well fitted by the Herschel–Bulkley rheological model with a high determination coefficient (R2 = 0.995–0.999). The detailed model parameters, including the yield stress (σ0), consistency coefficient (K), flow behavior index (n), and determination coefficient (R2) of each samples flow curve, were summarized in .
Table 3. Herschel-Bulkley parameters of the native starch and the heat-moisture treated sweet potato starch at different water levels
Figure 2. Steady shear flow curves parameters of the native starch and the heat-moisture treated sweet potato starch at different water levels
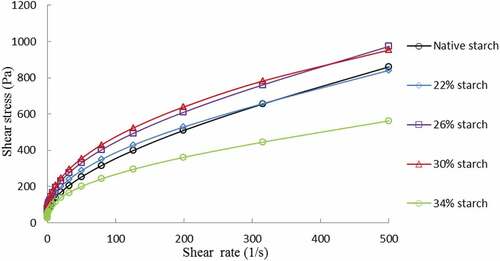
As shown in , the shear stress of all samples increased with the increasing shear rate. HMT samples have an increase in the shear stress at moisture content from 22% to 30% compare to native sample. However, the sample at moisture content 34% has a conspicuous decrease in the shear stress. This reduction of the shear stress might be due to in the partial gelatinization of starch during a certain amount of water, resulting weakening the molecular interactions in the starch pastes. These values of the behavior index (n) of all samples were lower than 1 (n = 0.32–0.47), indicating that all sample pastes exhibited a pseudoplastic flow behavior. In general, the flow behavior index shows the extent of deviation from Newtonian behavior, specifically, n < 1 denotes that shear-thinning behaviors of the paste system.[Citation30] Furthermore, the higher shear-thinning behavior tendency is influenced by increasing amylose content. Obviously, the HMT samples were higher shear-thinning tendency than the native sample, which should be explained by the high liability of amylose to short-term retrogradation contributing the paste with higher amylose containing more amylose pre-gel cluster. The more the micro-particles in paste samples, the higher the severity of the particle reorientation was upon shearing, and this eventually leads to a higher shearing-thinning tendency. This result was consistent with the pasting properties result of the setback value (). Similarly, the HMT samples were observed to have higher values for the consistency coefficient (K) than the native sample (). Wang Y, Ye F, Liu J, et al. reported that the higher amylose content was accompanied with the significantly higher values in K, and also implied that the higher values K, the more the resultant paste were a solid tendency,[Citation31] which be explained the vermicelli textural quality of hardness increasing by increasing moisture content during HMT ().
Table 4. Vermicelli texture parameters of the native starch and the heat-moisture treated sweet potato starch at different water levels
The mode of oscillatory testing, i.e. the frequency sweep, was measured to evaluate the change of the gel structure and viscoelasticity during HMT. The changes of the storage modulus (G′) and loss modulus (G″) as a function of frequency for the native and HMT sweet potato starches are presented in . As shown in , for all samples, the G′ was significantly higher than G″, and the highest tanδ value was less than 0.3 (), indicating that all of the samples were more elastic or solid-like nature.[Citation32] The G′ values of HMT samples were higher than that of native sample and increased with the increase as the moisture content from 22% to 30% and then decreased with further increase in moisture content, suggesting that the degradation of starch molecules could lead to the falling of the G′. The G″ values of HMT samples also were higher than that native sample, an exception of the moisture content at 34%. Similarly, it could be seen in , the samples had low tanδ values at low frequency, indicating a tendency toward solid-like behavior for gels at high deformation rates. After heat-moisture treatment, the tanδ values were decreased as compared with those of the native starch. () This result suggested the complex effect on the starch structure by heat-moisture treatment, as well as the extent of breaking of the native starch structure and the ordered molecular structures formation or maintenance at different moisture content during HMT.
Figure 3. Dynamic mechanical spectra as a function of frequency of the native starch and the heat-moisture treated sweet potato starch at different water levels (Closed symbols represent G′, open symbols represent G”)
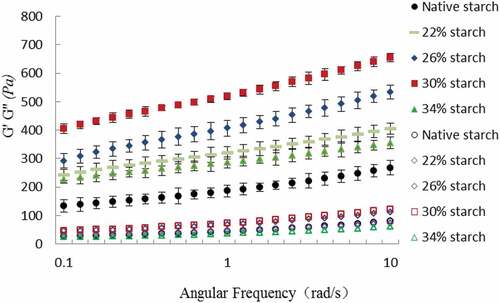
Figure 4. Tanδ as a function of frequency of the native starch and the heat-moisture treated sweet potato starch at different water levels
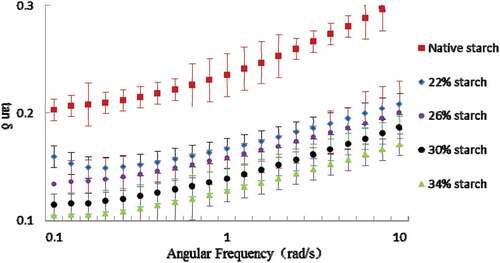
In the creep tests, constant stress was applied to these samples and these changes in strain or compliance was measured over time. shows an example of the creep curves of the native sample and these samples by heat-moisture treatment with different moisture content. The creep curves rapidly reached the maximum compliance values, suggesting that the nonlinear response to stress, which indicated that those of samples were normal behavior of viscoelastic materials combing both elastic and viscous components. HMT samples exhibited the lower compliance values than native sample during creep experiment, and those of HMT samples compliance values decreased with the increasing moisture, however, the compliance values increased from moisture content at 30% to at34%. Previous study reported that the creep curve behavior was mainly influenced by the reorientation or alignment of microstructure, and the compliance value reflected the gel strength and the molecules flexibility.[Citation33]A high compliance value corresponded to a weak gel system with high deformability, whereas a low compliance value corresponded to a strong gel system with low deformability. As shown in , HMT increased to the strength of starch gels, and the extent of strengthening effect was linked to the moisture content. The compliance values of HMT samples were smaller than that of a native one, suggesting that HMT samples are characteristic of stronger or harder structures than the naïve sample. The phenomenon of the compliance value increased at moisture content 34%, indicating that this HMT sample had more deformability than the other HMT samples. Such result is in good agreement with the dynamic viscoelasticity results ( and ) and the HMT samples product vermicelli texture property (). Thereby, it can be concluded that the proper moisture content results in a better gel characteristic of starch paste during HMT.
Vermicelli quality
In view of the texture of the cooked Vermicelli, which is the most critical characteristic that determines the consumer acceptance of Vermicelli products, a wide variety of textural parameters were observed in all Vermicelli samples. The hardness, springiness, and chewiness of the Vermicelli prepared from native sweet potato starch and from the various HMT starches with different moisture contents varied from 490.5 to 1332.3, from 0.91 to 0.95, from 396.8 to 1030.9, and from 366.9 to 953.2, respectively (). All textural parameters of the HMT samples significantly increased with increasing moisture content. In consideration of the definition of the textural parameters of springiness and chewiness, all of these texture indexes were associated with the change of the hardness. The change in the hardness may be attributed to the higher amylose content of the HMT starch, which caused an increased retrogradation of the starch during gel formation and consequently a stronger gel structure. The gel firmness was mainly caused by the retrogradation of the starch gel, which was associated with the hydrogen bonds with water, the crystallization of amylopectin, and the cross-linking in amylose portion during HMT, leading to harder gels.[Citation21] This result is consistent with a previous finding that the setback value was increased by HMT with increasing moisture content. The hardness values recorded in this work agreed well with those reported by Collado and Corke et al.[Citation34] who recorded higher hardness values of vermicelli for HMT starches than for native one.
The results for the various textural properties of the native and HMT samples revealed that the vermicelli prepared by HMT displayed an increased hardness, chewiness and elasticity compared with those of the native sample as the moisture content increased. Furthermore, the textural index of hardness has a significant positive correlation with the sensory mouthfeel of hardness or firmness. Since no correlation was explicit between the physical-chemical properties and the vermicelli quality, the starch gel properties appear to be more suitable for predicting final vermicelli quality. Nura et al. stated that the improved vermicelli was reflected by the gels,[Citation35] which had firmer texture, higher springiness, and chewiness, indicating that HMT significantly improved the textural quality of sweet potato starch vermicelli in this work. Contextually, high firmness (pasting property) and elasticity (rheological property) of the starch gel can predict high bite and stretch hardness of the cooked vermicelli.
Conclusion
The crystalline, pasting, thermal, rheological, and vermicelli texture properties of sweet potato starch were significantly affected by HMT. X-ray results displayed that the crystallinity of the HMT starches increased compared with that of native starch, although the crystallinity reduced with increasing moisture content. RVA results revealed that the HMT starches showed an increased setback viscosity and a reduced breakdown viscosity, indicating that the HMT starches had more amylose leaching to lead a stronger retrogradation tendency than native starch. The changes in pasting gel properties according to rheometer and textural analyses indicated that the HMT starches had a stronger elasticity behavior than native starch. Results for the pasting, thermal and rheological properties were confirmed by the effect of amount of leached amylose. In addition to amylose content, our hypothesis is that the starch molecule structure, especially the amylose fine structure is a significant determinant of gel properties in the present work. Work is now in progress to investigate the structural changes of HMT starch at the granule and molecule scales as affected by HMT with the purpose of obtaining a more accurate and comprehensive understanding of the effect of HMT on starch gel properties. The HMT sweet potato starch vermicelli exhibited higher hardness, springiness, and chewiness textural properties than native sweet potato starch. Thus, HMT is an effective strategy for improving the textural quality of sweet potato starch vermicelli. These mechanistic relations among starch structure, paste properties, and vermicelli quality during HMT will provide useful information for the starch vermicelli industry. It could be got further discussion about the suitable parameters range of sweet potato starch vermicelli product by controlling the HMT gel property.
Additional information
Funding
References
- Collado, L. S.; Corke, H. Properties of Starch Noodles as Affected by Sweetpotato Genotype. Cereal Chem. J. 1997, 74(2), 182–187. DOI: 10.1094/CCHEM.1997.74.2.182.
- Jiranuntakul, W.; Puttanlek, C.; Rungsardthong, V.; Puncha-Arnon, S.; Uttapap, D. Microstructural and Physicochemical Properties of Heat-Moisture Treated Waxy and Normal Starches. J. Food Eng. 2011, 104(2), 246–258. DOI: 10.1016/j.jfoodeng.2010.12.016.
- Sun, Q.; Han, Z.; Wang, L.; Xiong, L. Physicochemical Differences between Sorghum Starch and Sorghum Flour Modified by Heat-Moisture Treatment. Food Chem. 2014, 145, 756–764. DOI: 10.1016/j.foodchem.2013.08.129.
- Qi, X.; Tester, R. F. Heat and Moisture Modification of Native Starch Granules on Susceptibility to Amylase Hydrolysis. Starch‐Stärke. 2016;68(9-10):816-820.
- Yadav, B. S.; Guleria, P.; Yadav, R. B. Hydrothermal Modification of Indian Water Chestnut Starch: Influence of Heat-Moisture Treatment and Annealing on the Physicochemical, Gelatinization and Pasting Characteristics. LWT Food Sci. Technol. 2013, 53(1), 211–217. DOI: 10.1016/j.lwt.2013.02.007.
- Da Rosa Zavareze, E.;. Dias A R G. Impact of Heat-Moisture Treatment and Annealing in Starches: A Review. Carbohydr. Polym. 2011, 83(2), 317–328. DOI: 10.1016/j.carbpol.2010.08.064.
- Sui, Z.; Yao, T.; Zhao, Y.; Ye, X.; Kong, X.; Ai, L. Effects of Heat–Moisture Treatment Reaction Conditions on the Physicochemical and Structural Properties of Maize Starch: Moisture and Length of Heating. Food Chem. 2015, 173, 1125–1132. DOI: 10.1016/j.foodchem.2014.11.021.
- Sun, Q.; Nan, C.; Dai, L.; Xiong, L. Effect of Heat-Moisture Treatment with Maltitol on Physicochemical Properties of Wheat Starch. LWT Food Sci. Technol. 2015, 62(1), 319–324. DOI: 10.1016/j.lwt.2015.01.027.
- Guo, Z.; Zeng, S.; Lu, X.; Zhou, M.; Zheng, M.; Zheng, B. Structural and Physicochemical Properties of Lotus Seed Starch Treated with Ultra-High Pressure. Food Chem. 2015, 186, 223–230. DOI: 10.1016/j.foodchem.2015.03.069.
- Liao, L.; Wu, W. Fermentation Effect on the Properties of Sweet Potato Starch and Its Noodle’s Quality by Lactobacillus Plantarum. J. Food Process Eng. 2017, 40(3), e12460. DOI: 10.1111/jfpe.2017.40.issue-3.
- Sun, Q.; Si, F.; Xiong, L.; Chu, L. Effect of Dry Heating with Ionic Gums on Physicochemical Properties of Starch. Food Chem. 2013, 136(3), 1421–1425. DOI: 10.1016/j.foodchem.2012.09.061.
- Jacobs, H.; Delcour, J. A. Hydrothermal Modifications of Granular Starch, with Retention of the Granular Structure: A Review. J. Agric. Food Chem. 1998, 46(8), 2895–2905. DOI: 10.1021/jf980169k.
- Vieira, F. C.; Sarmento, S. Heat‐Moisture Treatment and Enzymatic Digestibility of Peruvian Carrot, Sweet Potato and Ginger Starches. Starch‐Stärke. 2008, 60(5), 223–232. DOI: 10.1002/(ISSN)1521-379X.
- Vermeylen, R.; Goderis, B.; Delcour, J. A. An X-Ray Study of Hydrothermally Treated Potato Starch. Carbohydr. Polym. 2006, 64(2), 364–375. DOI: 10.1016/j.carbpol.2005.12.024.
- Li, S.; Ward, R.; Gao, Q. Effect of Heat-Moisture Treatment on the Formation and Physicochemical Properties of Resistant Starch from Mung Bean (Phaseolus Radiatus) Starch. Food Hydrocolloids. 2011, 25(7), 1702–1709. DOI: 10.1016/j.foodhyd.2011.03.009.
- Hoover, R.;. The Impact of Heat-Moisture Treatment on Molecular Structures and Properties of Starches Isolated from Different Botanical Sources. Crit. Rev. Food Sci. Nutr. 2010, 50(9), 835–847. DOI: 10.1080/10408390903001735.
- Hoover, R.; Vasanthan, T. Effect of Heat-Moisture Treatment on the Structure and Physicochemical Properties of Cereal, Legume, and Tuber Starches. Carbohydr. Res. 1994, 25(2), 33–53. DOI: 10.1016/0008-6215(94)84121-7.
- Tattiyakul, J.; Asavasaksakul, S.; Pradipasena, P. Chemical and Physical Properties of Flour Extracted from Taro Colocasia Esculenta (L.) Schott Grown in Different Regions of Thailand. Sci. Asia. 2006, 32(3), 279–284. DOI: 10.2306/scienceasia1513-1874.2006.32.279.
- Hormdok, R.; Noomhorm, A. Hydrothermal Treatments of Rice Starch for Improvement of Rice Noodle Quality. LWT Food Sci. Technol. 2007, 40(10), 1723–1731. DOI: 10.1016/j.lwt.2006.12.017.
- Chung, H. J.; Cho, A.; Lim, S. T. Effect of Heat-Moisture Treatment for Utilization of Germinated Brown Rice in Wheat Noodle. LWT Food Sci. Technol. 2012, 47(2), 342–347. DOI: 10.1016/j.lwt.2012.01.029.
- Liu, H.; Corke, H.; Ramsden, L. The Effect of Autoclaving on the Acetylation of Ae, Wax, and Normal Maize Starches. Stärke. 2000, 52(10), 353–360.
- Yadav, D. N.; Kaur, J.; Anand, T.; Singh, A. K. Storage Stability and Pasting Properties of Hydrothermally Treated Pearl Millet Flour. Int. J. Food Sci. Technol. 2012, 47(12), 2532–2537. DOI: 10.1111/ijfs.2012.47.issue-12.
- Palma‐Rodriguez, H. M.; Agama‐Acevedo, E.; Mendez‐Montealvo, G.; Gonzalez-Soto, R. A.; Vernon-Carter, E. J.; Bello-Pérez, L. A. Effect of Acid Treatment on the Physicochemical and Structural Characteristics of Starches from Different Botanical Sources. Starch‐Stärke. 2012, 64(2), 115–125. DOI: 10.1002/star.201100081.
- Chung, H. J.; Liu, Q.; Hoover, R. Impact of Annealing and Heat-Moisture Treatment on Rapidly Digestible, Slowly Digestible and Resistant Starch Levels in Native and Gelatinized Corn, Pea and Lentil Starches. Carbohydr. Polym. 2009, 75(3), 436–447. DOI: 10.1016/j.carbpol.2008.08.006.
- Lan, H.; Hoover, R.; Jayakody, L.; Liu, Q.; Donner, E.; Baga, M.; Asare, E. K.; Hucl, P.; Chibbar, R. N. Impact of Annealing on the Molecular Structure and Physicochemical Properties of Normal, Waxy and High Amylose Bread Wheat Starches. Food Chem. 2008, 111(3), 663–675. DOI: 10.1016/j.foodchem.2008.04.055.
- Lii, C. Y. I.; Chang, S. M. Characterization of Red Bean (Phaseolus Radiatus Var. Aurea) Starch and Its Noodle Quality. J. Food Sci. 1981, 46(1), 78–81. DOI: 10.1111/j.1365-2621.1981.tb14535.x.
- Ji Hong Ahn; Hye Rim Baek; Kyung Mi Kim; Gui Jung Han; Jun Bong Choi; Yang Kim; Tae Wha Moon. Slowly Digestible Sweetpotato Flour: Preparation by Heat-Moisture Treatment and Characterization of Physicochemical Properties. Food Sci. Biotechnol.. 2013, 22(2), 383–391.
- Huang, T. T.; Zhou, D. N.; Jin, Z. Y.; Xu, X.-M.; Chen, H.-Q. Effect of Repeated Heat-Moisture Treatments on Digestibility, Physicochemical and Structural Properties of Sweet Potato Starch. Food Hydrocolloids. 2016, 54, 202–210. DOI: 10.1016/j.foodhyd.2015.10.002.
- Silverio, J.; Svensson, E.; Eliasson, A. C.; Olofsson, G. Isothermal Microcalorimetric Studies on Starch Retrogradation. J. Therm. Anal. Calorim. 1996, 47(5), 1179–1200. DOI: 10.1007/BF01992822.
- Guha, M.; Ali, S. Z. Changes in Rheological Properties of Rice Flour during Extrusion Cooking. J. Texture Stud. 2011, 42(6), 451–458. DOI: 10.1111/jts.2011.42.issue-6.
- Wang, Y.; Ye, F.; Liu, J.; Zhou, Y.; Lei, L.; Zhao, G. Rheological Nature and Dropping Performance of Sweet Potato Starch Dough as Influenced by the Binder Pastes. Food Hydrocolloids. 2018, 85, 39–50. DOI: 10.1016/j.foodhyd.2018.07.001.
- Niu, M.; Hou, G. G.; Zhao, S. Dough Rheological Properties and Noodle-Making Performance of Non-Waxy and Waxy Whole-Wheat Flour Blends. J. Cereal Sci. 2017, 75, 261–268. DOI: 10.1016/j.jcs.2017.05.002.
- Samutsri, W.; Suphantharika, M. Effect of Salts on Pasting, Thermal, and Rheological Properties of Rice Starch in the Presence of Non-Ionic and Ionic Hydrocolloids. Carbohydr. Polym. 2012, 87(2), 1559–1568. DOI: 10.1016/j.carbpol.2011.09.055.
- Collado, L. S.; Mabesa, L. B.; Oates, C. G.; Corke, H. Bihon‐Type Vermicelli from Heat‐Moisture‐Treated Sweet Potato Starch. J. Food Sci. 2001, 66(4), 604–609. DOI: 10.1111/jfds.2001.66.issue-4.
- Nura, M.; Kharidah, M.; Jamilah, B; Roselina, K. Textural Properties of Laksa Noodle as Affected by Rice Flour Particle Size. J. Int. Food Res. 2011, 18(4), 1309–1312.