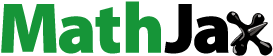
ABSTRACT
Cassava (Manihot esculenta, Grantz) leaves are known for their pharmacological and nutraceutical properties, but the extraction of active compounds in CL has not been optimized yet. In this study, the optimal parameters for the extraction of flavonoids from cassava leaves were determined using a Taguchi L9 orthogonal array design. The extraction variables included: concentration of ethanol, temperature, the solid: liquid ratio and the extraction time. Optimum extraction conditions were 50% ethanol at 50°C for 4 h at a solid: liquid ratio of 1:40. The total flavonoids obtained under these conditions were 877.7 mg/100 g extract and rutin content was 622 mg/100 g as determined by HPLC-UV. Seven flavonoid compounds were identified by HPLC-FTICR-MS analysis. The relative amount of each compound in the extract was 0.96% of clovin, 4.81% of myricetin-3-O-rutinoside, 1.25% of robinin, 58.89% of rutin, 2.51% of hyperoside, 29.31% of nicotiflorin, and 2.28% of narcissin of the total flavonoids. Therefore, CL has the potential to be explored as the medicinal foods or therapeutics for improving human health.
Introduction
Cassava (Manihot esculenta, Grantz) is commonly known as Yuca, mandioca or tapioca is an annual plant which belongs to the genus cassava. Cassava is widely grown in tropical and subtropical countries of Africa, Asia and Latin America with an estimated production of 276.7 million tons (FAOSTAT, 2013). Generally, cassava tuber is the common edible part of the plant. Cassava leaves (CL) are also consumed as a vegetable in Latin America and is used as an ingredient in sauces.[Citation1] In China, only the tuber is consumed, and large amounts of tuber peel, leaves, and stalks are generated as waste. Inadequate waste management of this by-product can lead to environmental pollution and loss of this bioresource.
Passos et al.[Citation2] argued that CL deserves more attention as a source of protein and nutrients for human nutrition and should get as much attention as the tubers since it contains high amounts of fiber, vitamins, B1, B2, C, crude protein (17.7–38.1% DM: dry matter basis), essential amino acids, and carotenoids. CL has been reported to possess compounds that have pharmacological properties including anti–inflammatory, anti-oxidative, and renal protection.[Citation3,Citation4] Furthermore, some studies indicated the effectiveness of CL against mycosis hemostatic agent and prevention of headache as well as anthelmintic agent.[Citation3] Fresh CL ethanol extracts inhibited protein synthesis and demonstrated antiviral and anti-mycobacterial activities.[Citation3] These pharmacological actions are believed to be due to phenolic compounds in CL such as tannins, anthocyanidins, cyanidin, and delphinidin.[Citation4,Citation5]
Ultrasonic-assisted extraction (UAE) is a new technology that supports the extraction of biological compounds from cellular materials of plants and other biological systems that has been widely reported in literature.[Citation6] UAE is regarded as an efficient and inexpensive alternative to conventional extraction systems.[Citation6] High-pressure liquid chromatography Fourier transform ion-cyclotron resonance mass spectrometry (HPLC-FTICR-MS) is an analytical tool to determine the accurate mass of precursor and product ions, and also is a useful tool for the identification of flavonoids. The instrument uses a linear ion trap that automatically permits easy control and selection of the amounts of ions sent to the ion-cyclotron resonance (ICR) cell compared to the conventional FTICR-MS, High mass accuracy of analyte compound can be achieved including in a rapidly changing concentration across the peaks of atypical high-performance liquid chromatography (HPLC).[Citation7] HPLC- FTICR-MS has been successfully applied to solve various analytical problems, including analysis of drug mixtures,[Citation8] analysis of trace impurities in a drug substance, proteomics analyses, toxicology, and forensic sciences, microbiology, and oligosaccharide analyses.[Citation7]
The aim of the study was to optimize the extraction of total flavonoid from CL using OED and UAE system and to determine the acute oral toxicity of the obtained extract. To improve the purity of the obtained extracts, six macroporous resins (HPD 100, D151, 001 ×1.1, NKA-9, H103, and D101) were investigated. Furthermore, the flavonoid compounds in CL extracts were identified using the HPLC- FTICR-MS technique. Therefore, this study will supply a good base for the exploitation of CL as nutritional food and source of bioactive compounds that will support comprehensive utilization of this natural by-product.
Materials and methods
Chemicals and equipment
Ultrapure water was prepared using a Millipore Direct®Q3 (Millipore Corp., MA, USA) and was used throughout the study. All of the reagents were of analytical or HPLC grade. Ethanol andethyl acetate were purchased from (Tianjin Biotechnology Co., LTD, Tianjin, China). Acetonitrile (HPLC grade) and rutin were purchased from Sigma Chemical Company (St. Louis, MO, USA). HCl, NaOH and macroporous resins (HPD, D151, 001 ×1.1, NKA-9, H103, and D101) were purchased from (Tianjin Biotechnology Co., LTD, Tianjin, China). UV-Vis absorption spectra was recorded on a TU-1900 double-beam UV-spectrophotometer (Beijing Purkinje General Instrument Co. Ltd., Beijing, China). Thermo Scientific LTQFT (Thermo Fisher Co. Ltd., San Jose, CA, USA) HPLC-MS, Ultrasonic extractor (BRANSON 2210., USA), Vacuum rotary evaporator (Buchi 461, Switzerland). A Shimadzu LC-20 AB high-performance liquid chromatography (HPLC) system (Shimadzu Corporation, Japan) consisted of a LC-20AB pump, a SPD-20A UV/VIS detector. Screwcap sample bottles (1.5 mL) and a nylon filter membrane (0.2 μm, 26 mm) were supplied by Waters (Milford, MA, USA).
Materials
CL of Hunan No.5 cultivar was obtained during harvesting season from the Research Center of Cassava, Tropical Crop Variety Resources Institute, Chinese Academy of Tropical Agriculture (Hainan, China).
Sample preparation
The samples were cleaned using running tap water and rinsed with distilled water prior to use.[Citation9] The CL was frozen at −20°C and freeze-dried (Martin Christ Co., LTD, Osterode, Germany). The dried CL was powdered using the micro plant grinding machine and were screened through a 40-mesh sieve to obtain particles with a mean diameter of ≤420 μm. These powders were flushed with nitrogen before being stored in sealed containers at −20°C until analysis.
Extraction procedure
Optimization of UAE extraction the flavonoids
An orthogonal L9 (3)4 experiment design was used to optimize the extraction conditions of flavonoids from the CL. Four independent UAE (THC-2B, Jining Tianhua Ultrasound Electronic Instrument Co., Ltd) extraction parameters (the concentration of ethanol, extraction temperature, the solid: liquid (S/L) ratio and extraction time) was investigated (). The investigated extraction parameters were studied at three levels; i. e. concentration of ethanol (X1: 40%, 50%, and 60%), extraction temperature (X2: 50, 60 and 90 °C), S/L ratio (X3: 1:20, 1:30 and 1:40 mg/mL) and extraction time (X4: 1 h, 2 h, and 3 h) (). The experiments run was carried out in triplicate and in a randomized order to avoid personal or subjective bias during the extraction process.[Citation10] The extract was centrifuged at 6000 r.p.m for 10 min, then 1 mL of supernatant was filtered by a nylon filter membrane (0.2 μm, 26 mm) before HPLC-UV analysis. A control containing only ethanol and methanol (without samples) was similarly prepared. The total flavonoids content was used as the dependent variable and measured as described in Section 2.6.2.
Table 1. Levels of the orthogonal test
Dispersive solid-phase extraction (DSPE)
Six macroporous resins (HPD 100, D151, 001 ×1.1, NKA-9, H103, and D101) were evaluated for their DSPE ability and to clean-up the obtained extracts. Information regarding the characteristics of six macroporous resins is shown in Supplementary Table S-1. The purification of the CL flavonoids was optimized using the macroporous resin types, the amount of resin to flavonoids solution, and the concentration of the elution solvent “ethanol” and all the experiments were conducted in triplicate.
Pretreatment and activation of resins
The macroporous resins were pretreated and activated according to the manufacturer’s instructions. Firstly, all the macroporous resins were washed by distilled water and filtered using a nylon filter cloth with mesh size of 0.3 mm in order to retain resins with particle diameters >0.3 mm. Then, all the resins were individually soaked in two-bed volumes (BV) with 95% ethanol for 24 h.[Citation11] After soaking, each type of resin was filled in a glass column followed by rinsing with 95% ethanol. Subsequently, all the resins were rinsed with the distilled water (two BV) in order to remove the ethanol. The generation of the resins was achieved by subjecting the columns to the following washing steps; one BV of 4% (w/v) sodium hydroxide, two BV of distilled water, one BV 4% (v/v) hydrochloric acid, and distilled water until the pH of eluent is pH = 7.
Purification of flavonoid compounds
The CL extracts that were obtained under the UAE optimized extraction conditions and then centrifuged at 5500 xg for 30 min. The resultant supernatants were loaded on the different resin columns to saturation where the concentration of flavonoids in the loaded and run through solution were equal. The flavonoid compounds were adsorbed onto the column while for other water-soluble compounds were eluted by two BV of distilled water until eluent become clear. The adsorbed flavonoids were eluted by acidified ethanol (0.5% V/V of hydrochloric acid) until the eluent was colorless.
The eluents were concentrated by a rotary evaporator at 60°C and then concentrated extracts were frozen at −20°C for freeze-dried. The efficacy of the resin in adsorbing the CL flavonoid compounds were determined using the method following as section 2.6.3. Ethanol at different concentrations (10%, 30%, 50%, 70%, and 90%) were used to investigate the best concentration for the elution of the adsorbed flavonoid compounds (Supplementary Table S-2). The dynamic adsorption capacity of the resin and the adsorption and desorption efficacies were determined as described below (Supplementary Table S-3).
Desorption of flavonoid compounds
Macroporous resin (5 g) was saturated with flavonoids compounds, transferred to a flask (250 ml) with different concentrations of acidified ethanol were used to desorb the flavonoids. The mixture was shaken at 125 rpm for 1 h at 25°C. The total flavonoid content of the eluted materials was determined by HPLC-UV (section 2.6.3).
Methods
Carotenoids extraction and determination of total carotene content
Total carotene content of the extracts was measured as described by De Carvalho et al.[Citation12] A 15 g of the sample was added with 3 g of celite 454 (Tedia, Ohio, USA). Then, 25 mL of acetone was added until a paste was obtained, which was transferred into a sintered funnel (5 μm) coupled to a 250 mL Buchner flask and filtered under vacuum. The sample extraction was repeated three times until the filtrate was colorless. The obtained extract was transferred to a separation funnel (500 mL) containing petroleum ether (40 mL), followed by slow addition of ultrapure water (Milli-Q -Millipore) to remove acetone and prevent emulsion formation. The water phase was discarded, and this procedure was repeated four times. The extract was transferred through a funnel containing 15 g of anhydrous sodium sulfate and obtained in a volumetric flask (50 ml). The absorbance of the obtained extract was measured at 450 nm. The total carotenoid content was calculated using the following formula:
where = Absorbance;
= Total extract volume;
= sample weight in grams;
=2592 (β-carotene Extinction Coefficient in petroleum ether).
HPLC determination of total flavonoids content (TFC) in CL
The CL extract samples were centrifuged for 20 min at 3582 xg and −4°C. The supernatant was filtered using a membrane filter (0.22 μm) and was analyzed by HPLC-DAD (LC-20 AB, Shimadzu). The HPLC system was connected to a reversed-phase Shim-pack VP-ODS column (5 µm, 300 mm x 416 mm i.d.) using a quaternary pump, an autosampler (SIL-20A), and a diode array detector (SPD-20A) for detection of eluted compounds monitored by LC solution software. The injection volume was 20 μL for all standards and samples. The mobile phase was methanol (solvent A) and 0.11% acetic acid solution in water (solvent B). A gradient program was set at 0–15 min, linear gradient from 35% to 85% B, then was maintained at 65% until 25 min. The flow rate was maintained at 1 mL/min. The detector was set to a wavelength of 360 nm and the column temperature was maintained at 30°C. So, the flavonoids were reported as mg rutin equivalent/g cassava leaf. Rutin standard series was used to produce seven points calibration curves (0 to 500 µg/ml).
Identification of flavonoid in CL using HPLC-FTICR-MS
High-performance liquid chromatography coupled with Fourier transform ion cyclotron resonance mass spectrometry (HPLC-FTICR-MS) analyses were performed on a Thermo Scientific LTQ FT equipped with a Surveyor LC Plus system, a Surveyor MS pump plus and a Surveyor autosampler (Thermo Fisher Co. Ltd., San Jose, CA, USA). MassLynxTM software (version 4.0, Waters, Milford, MA, USA) via electrospray ionization (ESI) interface operating in positive mode was used to control the instruments, data acquisition, and processing.
Liquid chromatography
The analysis was carried out as a previously described Wu et al.[Citation7] and alter modified the method with slight changes. Samples were separated on a ZORBAX Eclipse Plus-C18 column (100 x 2.1 mm, 3.5 mm) protected by a ZORBAX SB-C18 guard column (12.5 x 2.1 mm, 3.5 mm). The analysis was conducted using an injection volume of 5 µL under isocratic conditions at a flow rate of 0.2 mL/min. The mobile phase was acetonitrile (solvent A) and 0.3% ethyl acetate solution in water (solvent B). A gradient program was set at 0–10 min, linear gradient from 10% to 15% A, 10–45 min linear gradient from 15%-25% A, 45–50 min linear gradient from 25%-35% A, and then maintained at 35% A until 50 min. The system then was recovered to initial conditions within 30 s, and the column was reconditioned for 4.5 min. The detector was set to a wavelength of 270 nm and the column temperature was maintained at 25°C.
Mass spectrometry
Ultrahigh-purity collision gas and high-purity N2 were used as a nebulizing gas. The optimized parameters in the positive ion mode were as follows: ion spray voltage of 3.5 kV; capillary temperature of 300°C capillary voltage of 30 V; sheath gas flow rate of 35 L/h; auxiliary gas flow rate of 10 L/h; sweep gas flow rate of 3 (arbitrary units); and tube lens of 90 V. Compounds were detected by full-scan mass analysis from m/z 100 to 2000 at a resolving power of 100,000 with data-dependent MS/MS analysis triggered by the most abundant ions from the parent mass list of predicted metabolites (mass list: 757, 626, 740, 610, 464, 594, 624) followed by MS/MS analysis on the most abundant product ions. The resolving power used for multiple-stage mass analysis was the same as that employed for the full-scan mass analysis. Data acquisition and reduction were performed with Xcalibur version 1.4 software (Thermo Fisher) after HPLC-FTICR-MS analysis.
Statically analysis
All the experiments were analyzed in triplicate and all the results are reported as mean ± standard error mean. Analysis of variance (ANOVA) was used to investigate the effects of the extraction variables on the recovery of TFC. The difference among the means were separated using Fisher test at P < .05. In order to investigate the effect of the extraction conditions of the total flavonoids content from CL, the Orthogonality Experiment Assistant software (Sharetop Studio Software, Beijing, China) was used.
Result and discussion
Selection of operational conditions: the effect of the extraction solvent variables
The recovery of TFC obtained using the different extraction solvents is shown in supplementary Fig. S-2. The two highest recoveries of TFC were attained using methanol and ethanol as the extraction solvents (1.5% and 1.2%, respectively). While the highest recovery of TFC was obtained with methanol, which might be due to methanol as a high polar solvent had a higher affinity for polar compounds such as flavonoid compounds. However, in subsequent studies ethanol was used the extraction solvent as it is safer for human health compared to methanol.[Citation13] The recovery of TFC obtained from the UAE optimization is shown in . Finally, all the extraction variables had significant effects on TFC (p < .05) () and the influence of the extraction factors in decreasing order were; concentration of ethanol > the S/L ratio > temperature > time. The optimum extraction parameters were 50% ethanol as extraction solvent at a S/L ratio 1:40 and 55°Cfor 2 h that resulted in TFC of 877.71 mg/100g dry extract, which is higher than the TFC value (52.38 mg/g of dry powder) reported by Zhou et al.[Citation14] for CL extract obtained with 90% ethanol extracted by UAE. The extraction solvents have a big effect on TFC due to the proportion of individual groups, which easily dissolve in ethanol from the total amount of flavonoids compounds because of the principle “like dissolves like”.[Citation13] In turn, TFC obtained in CL from Tamil Nadu (238 mg/g extract) extracted with acetone.[Citation15] Although a direct comparison of this study results with the result of Suresh et al.[Citation15] is not appropriate since the extraction method and the sample is different. However, it still confirms that TFC can be affected by the extraction solvents.
Table 2. Results of the orthogonal test
Orthogonal array experiment
In this study, four three-level process parameters, i.e. concentration of ethanol (%), extraction temperature (°C), solid/liquid (S/L) ratio and the extraction time (min) were considered. Factorial design using four extraction parameters at three levels (34) would have resulted in 81 experiment runs. However, L9 orthogonal array only needed nine experiment runs (). The optimal extraction conditions for TFC were obtained at 70°C with 50% ethanol in S/L ratio of 1:40 for 2 h. All the values given in were the mean of triplicate experiments. The sequence of the experiments was randomized to avoid any personal or subjective bias.
Analysis of variance
Analysis of variance (ANOVA) was used to investigate the effects of the extraction variables on the recovery of TFC. Fisher test was used to determine which process parameter had a significant effect on TFC (). Results of ANOVA indicated that concentration of ethanol and the S: L ratio were highly significant factors affecting TFC ().
Table 3. Variance analysis of the results of orthogonal test
Adsorbent capacity of different resins to CL flavonoids compounds
The dispersive solid-phase extraction (DSPE) was used as clean-up procedure, which is a rapid and simple approach to achieve high accuracy and recovery of TFC. In particular, macroporous resins can be used to remove proteins and pigments that could interfere with the targeted compounds during the processing of the complex biological matrix.[Citation16] The highest TFC can be obtained with 50% ethanol (supplementary Table S-2). The TFC absorption capacity of the six resins was evaluated and was found to be in the following order: HPD 100 > D 101 > NKA-9 ≥ H103 > D 151 > 001 × 1.1. The adsorbing capability of TFC in CL extract was primarily related to the pore radius and, to a lesser extent, on the surface area. For example, HPD 100 which had the largest pore radius (0.3–1.2 mm) and surface area (650–700 m2/g) exhibited the best adsorption capacity. The total adsorption capacity of HPD 100 resin (215 mg RE/g) was more than twice that of the other resins. According to a previous study by Liu et al.[Citation11] the TFC total adsorption capacity of HPD 100 resin was assessed by TFC, and obtained 9.1 mg RE/g, which was 23 times lower than this study.
The nonpolar H103 resin had similar pore radius (0.3–1.2 mm), and bigger surface area (1000–1100 m2/g) compared to HPD 100, but showed the second largest adsorption capacity suggesting that a larger surface area is less critical factor for the adsorption of flavonoid compounds compared to polarity. In support of this contention, NKA-9, a strong polar resin, had the smallest surface area (250∼290 m2/g) and similar pore radius (0.3–1.2 mm), and showed similar adsorption ability to H103 resin. The 001 × 1.1 resin is a strong polar resin that has similar pore radius (0.3–1.2 mm). This resin had the lowest adsorption capacity among all the resins. These findings are consistent with the results reported by Di Mauro et al.[Citation17] despite some differences in the exact ranges of pore radius and surface area of the resins used in their study.
Determination of the total carotene content in CL
In this study, the average total carotene content obtained from CL with petroleum ether extraction was 26.7 mg/100 g dry weight (Fig.S-1). Chávez et al.[Citation18] investigated the total carotene concentration in cassava leaves and reported the concentration in the range of 23 to 86 mg/100g fresh weight with an average. It may not be feasibly compared to the results in this study because of the different measurements for extraction and units applied in total carotene content. The differences in concentration of carotene are likely due to different types of cassava since Achidi et al.[Citation19] reported 3.3 mg/100 g dry weight basis (dwb) and 4.5 mg/100 g dwb in two different cassava leaves varieties. It is worth noting that Iglesias et al.[Citation20] reported higher carotene content in the CL more than cassava roots and the carotene content in the leaves can be up to tenfold higher in CL than that in cassava roots.[Citation18] In Chávez et al.[Citation18] study, the total carotene content indicated the wide range in the various species of cassava leaves that ranged from 23 to 86 mg/100 fresh weight that further confirm the species, and the cultivation region play important role in the content of carotene.
Determination of the level of total flavonoids content (TFC) in CL
TFC in the CL extracts was determined directly using an HPLC as shown in Fig S-4. The extracts were obtained under optimal extraction conditions and were cleaned up using the HPD resin clean-up (Fig.S-2). The concentration of TFC was expressed as rutin equivalent obtained 6.37 mg/100g dry weight which is substantially higher than the content reported by Yong et al.[Citation21] for CL from Hainan was 1 172.17 mg/100 g dry weight using chemical extraction (NaHCO3) method optimized by response surface methodology combined with Box-Behnken design under the condition as at 79.65°C, in S:L ratio of 1:30.77 mL/g for 11.82 min with added 0.03 g of NaHCO3. In a study by Yong et al.[Citation21] the TFC in CL from Hainan was higher than that in this study. The probable reason is that the type of the CL has contributed to the concentration of TFC, and the region and the growth environment also play important factors for the concentration of TFC. Suresh et al.[Citation15] evaluated TFC value in CL from Tamil Nadu and reported 116, 124 and 2.38 mg/g fresh extract in methanol containing 1% HCl, methanol, and acetone extracts, respectively. Comparison of the result in this study with their finding is difficult because of the variation of TFC under the different extraction conditions and measurement units. Furthermore, in an additional study of CL from China using 90% ethanol extraction, 52.38 mg TFC/g dry powder was obtained by UAE.[Citation14] It is suggested that the various content of rutin in CL is mainly due to the environmental factors such as soil condition, UV, and temperature. In another study, He et al.[Citation22] found the rutin content in CL was 9.32 mg/g dry powder using 0.1% HCl-Methanol: H2O (7:3, V/V) and UAE. It is indicated that the different extraction methods were used to obtain different values of TFC, and the origin and environmental factor play as important role for the variation in TFC values.
Determination and identification of the seven flavonoids compounds character in CL
In order to identify the active compounds in CL, the ethanol extract was analyzed by HPLC/FTICR-MS. Seven compounds exhibited UV spectra that were characteristic of flavonoids compounds. Fragmentation of scheme is illustrated in Figure S-6–12. To identify the flavonoids compounds in the matrices of CL, retention times, UV-vis, mass spectral characteristics were compared to commercial standards, and online retrieval structure comparison with Chemindustry (http://www.chemindustry.com) and the American chemical abstracts database (www.cas.org). These resulted in the recognition of specific spectral patterns between the glycosylated flavones and their respective aglycones: rutin. For the preceding flavone pairs, the λ maxima values were the same as the glucuronic acid moiety providing little contribution to the UV absorbance pattern due to the lack of chromophores. Rutin had λ maxima of 270 nm. In addition, a mass spectral pattern was determined between the same three flavone pairs. Mass spectra of each flavone pair differed by only one ion peak: the [M + H] +of the glycosylate flavone. Each of the three glycone analytes’ spectra contained [M + H] + and an intense aglycone ion peak, [A + H] +, corresponding to the neutral loss of a glucuronic acid moiety, 176 amu, from the parent molecule (spectra shown in Fig. S-9). This neutral loss fragmentation pattern was attributed to in-source CID due to the high fragmentation voltage. Recent investigations by Wu et al.[Citation7] and Niu et al.[Citation8] successfully utilized HPLC/FTICR-MS for the structural elucidation of various-conjugated flavonoids, including glycosylated flavonoids. Based on the commercial standard, compound 4 was identified as rutin.
There were three flavonoids compounds that were quite similar to compound 4. Compounds 2 had only a saccharide group at C3, and gave an [M + H] + ion at m/z 627 (C27H30O17). The product ion of highest intensity, at m/z 481, and at m/z 319 appeared in the MS2 spectrum because of cleavage of the glycosidic bond by the neutral loss of C6H11O5 and C6H12O6, respectively, from the molecular ion of m/z 627 as shown in Figure S-7. Compound 2 was identified as myricetin-3-O-rutinoside (Fig. S-7), IUPAC Name:5,7-dihydroxy-2-(3,4,5-trihydroxyphenyl)-3-[(2S,3R,4S,5S,6R)-3,4,5-trihydroxy-6-[[(2R,3R,4R,5R,6S)-3,4,5-trihydroxy-6-methyloxan-2-yl]oxymethyl]oxan-2-yl]oxychromen-4-one. Compound 6 had two saccharide groups at C3 and gave an [M + H] + ion at m/z 595. The fragment ion of greatest intensity was detected at m/z 449, because of cleavage of the glycosidic bond between the two sugars at C3. The fragment ions at m/z 449 and m/z 287 were also formed by the neutral loss of C10H11O5 and C10H12O6, respectively, from the ion of m/z 595 (Fig. S-11). The mass spectral fragmentation pattern of nicotiflorin (6) was the same as that of myricetin-3-O-rutinoside (2), IUPAC Name:5,7-dihydroxy-2-(4-hydroxyphenyl)-3-[(2S,3R,4S,5S,6R)-3,4,5-trihydroxy-6-[[(2R,3R,4R,5R,6S)-3,4,5-trihydroxy-6-methyloxan-2-yl]oxymethyl]oxan-2-yl]oxychromen-4-one.
For the seventh peak (compound 7), two saccharide groups were found at C3 and had an [M + H] + ion at m/z 625 (C28H32O16,). The fragment ion of greatest intensity was detected at m/z 449, because of cleavage of the glycosidic bond between the two sugars at C3. The fragment ions at m/z 479 and m/z 317 were also formed by the neutral loss of C10H11O5 and C10H12O6, respectively, from the ion of m/z 595. The mass spectral fragmentation pattern of narcissin (7) was the same as that of myricetin-3-O-rutinoside (2) (Fig. S-12), IUPAC Name:5,7-dihydroxy-2-(3-hydroxy-4-methoxyphenyl)-3-[3,4,5-trihydroxy-6-[(3,4,5-trihydroxy-6-methyloxan-2-yl)oxymethyl]oxan-2yl]oxychromen-4-one. To identify the structure of the first peak (compound 1), MS and MS2 analysis of compound 1 were performed to obtain characteristic mass fragmentations. The MS analysis of the first peak (Rt = 15.9 min) gave an [M + H] + ion at m/z 757 (C33H40O20, calculated [M + H] + 757.229, error 0.23 ppm). In the MS2 spectrum, the highest product ion at m/z 610.82 [M + H]+ ion was formed by the neutral loss of a rhamnoside (C6H11O5, 146 Da), and the ions at m/z 464.89, and 303.01 were formed by the loss of C6H11O5 and C6H12O6, (146D, and 162D) respectively, from the saccharide group attached to the C-6 position of the aglycone, which is the characteristic loss of the sugar moiety from C-glycosylation. In the MS3 spectrum of the ion at m/z 464.89, the product ions at m/z 449.02 (base peak), 413.05, 344.92, 303.01 and 257.00 were also observed due to similar fragmentation to the MS2 spectrum (Fig. S-6). The Compound 1 was identified as cloven, IUPAC Name:2-(3,4-dihydroxyphenyl)-5-hydroxy-7-[(2S,3R,4R,5R,6S)-3,4,5-trihydroxy-6-methyloxan-2-yl]oxy-3-[(2S,3R,4S,5R,6R)-3,4,5-trihydroxy-6-[[(2S,3R,4R,5R,6S)-3,4,5-trihydroxy-6-methyloxan-2-yl]oxymethyl]oxan-2-yl]oxychromen-4-one.
The formula of third peak (compound 3) was calculated as C33H40O19 based on the FTICR-MS (m/z 741.25458, error 0.02 ppm), which indicated a theoretical protonated molecular mass of m/z 741. From the MS2 spectrum of peak 3, the [M +H] + ion at m/z 741 produced a prominent ion at m/z 594.92, shown the characteristic loss of C6H11O5 because of cleavage of the glycosidic bond between two sugars at C3. This also indicated that the acetyl group was not located on the lateral aldohexose residue. The further neutral loss of C6H11O5 (146D) led to a fragment ion at m/z 448.89 from the prominent ion at m/z 594.92. Next, the fragment ion at m/z 287.04 was attributed to the loss of a C6H12O6 (162D) residue from C6. The most intense fragment ion was detected at m/z 448.89, formed by the neutral loss of acetic acid from the ion of m/z 594.92. The fragment ions at m/z 432.98 and 413.24 were formed by the neutral loss of H2O and 2H2O, respectively, from the ion of m/z 448.89 (Fig S-8). In addition, the MS3 spectrum showed an ion of m/z 287.04, which was the most intense fragment ion in the MS2 spectrum. The main product ion (m/z 257.63) was formed by the neutral loss of 2H2O residue. From these data, compounds 3 was formed by conjugation of an acetyl group with the inner sugar on the C3 position of compound 3. The proposed fragmentation pathway of peak 3 is given in Fig. S-8. The mass spectral fragmentation pattern of peaks was named as robinin (3), which was the same as that of robinin (3), as both compounds had similar structures. IUPAC Name: 5-hydroxy-2-(4-hydroxyphenyl)-7-(3,4,5-trihydroxy-6-methyloxan-2-yl) oxy-3-[3,4,5-trihydroxy-6-[(3,4,5-trihydroxy-6-methyloxan-2-yl) oxymethyl]oxan-2-yl]oxychromen-4-one.
Representative HPLC/FTICR-MS chromatogram of flavonoids compounds in CL extract
In this study, HPLC-FTICR-MS was used to rapidly identify the structures of unknown flavonoids compounds in the CL extracts. The accuracy of HPLC-FTICR-MS m/z value analysis was within 3 ppm (<1ppm in most cases), which enabled rapid prediction of molecular structures and resulted in seven flavonoids compounds been identified, of which six (compounds 1, 2, 3, 5, 6, 7) are reported for the first time in CL. In order to obtain a good separation, identification and total ion current,[Citation23] a mobile phase consisting of acetonitrile and 0.3% aqueous ethyl acetate was chosen as the eluting solvent system. The representative HPLC/FTICR-MS chromatograms of the CL extract and seven authentic compounds are presented in Figures S-6–12. Seven peaks were detected and showed maximum absorption in wavelength range 190–300 nm in the UV spectra. Pseudo-molecular weights with highest mass accuracy of less than 3 ppm and multi-stage MS/MS fragmentation information were obtained for these seven compounds (). These seven peaks indicated similar retention behavior, similar UV spectra, and accurate mass data to five authentic compounds. Therefore, these compounds were confirmed as clovin (peak 1), myricetin-3-O-rutinoside (peak 2), robinin (peak 3), rutin (peak 4), hyperoside (peak 5), nicotiflorin (peak 6) and narcissin (peak 7). In order to obtain the fragmentation patterns and identify the other compounds in the CL extract, a high accuracy mass spectra and multi-stage MS/MS fragmentation information for the five authentic compounds were carefully analyzed. In this study, four authentic compounds had similar structural skeletons with different substituting groups at C-6. According to Wang et al.[Citation24] only hyperoside (peak 5) was exclusively identified as flavonoid C-glycoside with one saccharide group, while the two compounds identified as clovin (peak 1) and robinin (peak 3) were O-glycosyl-C-glycosyl flavonoids.
Table 4. Compositions and contents of cassava leaves flavonoids
Yield of flavonoids compounds in cassava leaves
As shown in , the major compound was rutin (compound 4) with a yield of 58.89% CL extract in cassava leaves. The second highest compound was six nicotiflorin with a yield of 29.31% extract. All other yields of flavonoids compounds were markedly lower than Rutin and nicotiflorin with compound 1 was the lowest around 0.96% extract of total flavonoids content in CL. The contents of compounds 2, 3, 5 and 7 were 4.81% extract, 1.25% extract, 2.51% extract, and 2.28% extract, respectively. A study by Marie-Magdeleine et al.[Citation3] used the shaking technology and methanol solvent to extract flavonoids compound in CL from Guadeloupe (French West Indies) and found that flavonoids are very strong coloration through Thin layer chromatography. Intarakasem et al.[Citation20] reported rutin content in Thai CL to be 123 mg RE/100g extract obtained with absolute methanol extraction. Which was four times lower than that in this study. The probable reason for this clear difference is that the origin and type of CL play important roles in the production of flavonoids content in CL. Further study, Wang et al.[Citation24] reported wide range of these compounds in CL extracts obtained from SC 09 variety: catechin (116.6–474.48 mg/kg), rutin (1590.08–4397.84 mg/kg), hesperidin (5.47–83.26 mg/kg), quercetin (0.55–14.42 mg/kg), kaemperol (17.2–32.05 mg/kg), and amentoflavone (125.61–642.09 mg/kg). Lower and wide range of contents were found in the other CL variety “SC205”: catechin (12.31–171.18 mg/kg), rutin (755.04–3989.45 mg/kg), hesoeridin (0.93–81.59 mg/kg), quercetin (0.56–24.74 mg/kg), kaemperol (17.13–56.42 mg/kg) and amentoflavone (243.69–883.02 mg/kg). It is worth noting that these varieties were from Hainan (China), suggesting that different varieties of CL grown in different locations contain different amounts and flavonoid compounds. The contents of flavonoid compounds in Chinese CL reported by various researchers and in this study were not expected to be the same due to extraction conditions, growth conditions, and the cassava variety. However, the dominant flavonoid compound was consistent among the studies showing rutin as the most content of flavonoids compounds in CL.
Conclusion
The chemical composition of major trace and heavy elements were found in CL, while the macrominerals had a higher content than the other elements, and also the value of total flavonoids and carotene content were higher than other plants. The evaluation of acute toxicity assay reflected that CL could be used as a functional food to improve human health. Identification and quantification of seven flavonoids in CL were carried out by HPLC/FTICR-MS analysis. The main peak was identified as rutin by comparison with the HPLC retention times of standard flavonoids, while the other six flavonoids were speculated based on their high accurate mass spectrometry of FTICR-MS, and they were identified as clovin, myricetin-3-O-rutinoside, robinin, hyperoside, nicotiflorin, and narcissin; the six flavonoids were reported for the first time in CL. The obtained results from this study would be helpful in further understanding the bioactive functions of CL.
Supplemental Material
Download MS Word (1.4 MB)Acknowledgments
This work has received financial support from Special Chinese Funds of National Public Welfare Research Project (nyhyzx07-013) in the Ministry of Agriculture and Rural Affairs, Taishan in Scholars Project in Shandong Province, National Special Support Program for High-level Personnel.
Supplementary material
Supplemental data for this article can be accessed here.
Additional information
Funding
References
- Latif, S.; Müller, J. Potential of Cassava Leaves in Human Nutrition: A Review. Trends Food Sci. Technol. 2015, 44(2), 147–158. DOI: 10.1016/j.tifs.2015.04.006.
- Passos, C. P.; Yilmaz, S.; Silva, C. M.; Coimbra, M. A. Enhancement of Grape Seed Oil Extraction Using a Cell Wall Degrading Enzyme Cocktail. Food Chem. 2009, 115(1), 48–53. DOI: 10.1016/j.foodchem.2008.11.064.
- Marie-Magdeleine, C.; Udino, L.; Philibert, L.; Bocage, B.; Archimede, H. In Vitro Effects of Cassava (Manihot Esculenta) Leaf Extracts on Four Development Stages of Haemonchus Contortus. Vete Parasitol. 2010, 173(1–2), 85–92. DOI: 10.1016/j.vetpar.2010.06.017.
- Santos, M. A. I.; Fraguas, R. M.; Braga, M. A.; Marques, T. R.; Duarte, M. H.; Dos Santos, C. M.; Correcirc, A. D. Antioxidants and Chlorophyll in Cassava Leaves at Three Plant Ages. Africa J. Agric. Res. 2013, 8(28), 3724–3730. DOI: 10.5897/AJAR2013.6746.
- Padmaja, G.;. Evaluation of Techniques to Reduce Assayable Tannin and Cyanide in Cassava Leaves. J. Agric. Food Chem. 1989, 37(3), 712–716. DOI: 10.1021/jf00087a029.
- Azmir, J.; Zaidul, I.; Rahman, M.; Sharif, K.; Mohamed, A.; Sahena, F.; Omar, A. Techniques for Extraction of Bioactive Compounds from Plant Materials: A Review. J. Food Eng. 2013, 117(4), 426–436. DOI: 10.1016/j.jfoodeng.2013.01.014.
- Wu, C.; Sheng, Y.; Zhang, Y.; Zhang, J.; Guo, B. Identification and Characterization of Active Compounds and Their Metabolites by High‐Performance Liquid chromatography/Fourier Transform Ion Cyclotron Resonance Mass Spectrometry after Oral Administration of a Herbal Extract of Epimedium Koreanum Nakai to Rats. Rapid Commun. Mass Spectrom. 2008, 22(18), 2813–2824.
- Niu, C.-Y.; Wu, C.-S.; Sheng, Y.-X.; Zhang, J.-L. Identification and Characterization of Flavonoids from Semen Zizyphi Spinosae by High-Performance Liquid Chromatography/Linear Ion Trap FTICR Hybrid Mass Spectrometry. J. Asian Nat. Prod. Res. 2010, 12(4), 300–312. DOI: 10.1080/10286021003752284.
- Zhang, H.; Birch, J.; Yang, H.; Xie, C.; Kong, L.; Dias, G.; Bekhit, A. E. D. Effect of Solvents on Polyphenol Recovery and Antioxidant Activity of Isolates of Asparagus Officinalis Roots from Chinese and New Zealand Cultivars. Int. J. Food Sci. Technol. 2018. DOI: 10.1111/ijfs.13829.
- Mandal, V.; Mohan, Y.; Hemalatha, S. Microwave Assisted Extraction of Curcumin by Sample–Solvent Dual Heating Mechanism Using Taguchi L9 Orthogonal Design. J. Pharm. Biomed. Anal. 2008, 46(2), 322–327.
- Liu, X.; Xiao, G.; Chen, W.; Xu, Y.; Wu, J. Quantification and Purification of Mulberry Anthocyanins with Macroporous Resins. Biomed Res. Int. 2004, (2004(5), 326–331.
- De Carvalho, L. M. J.; Gomes, P. B.; de Oliveira Godoy, R. L.; Pacheco, S.; Do Monte, P. H. F.; de Carvalho, J. L. V.; Ramos, S. R. R. Total Carotenoid Content, α-carotene and β-carotene, of Landrace Pumpkins (Cucurbita Moschata Duch): A Preliminary Study. Food Res. Int. 2012, 47(2), 337–340. DOI: 10.1016/j.foodres.2011.07.040.
- Zhang, H.; Birch, J.; Xie, C.; Yang, H.; Dias, G.; Kong, L.; Bekhit, A. E.-D. Optimization of Extraction Parameters of Antioxidant Activity of Extracts from New Zealand and Chinese Asparagus Officinalis L Root Cultivars. Ind. Crops Prod. 2018, 119, 191–200. DOI: 10.1016/j.indcrop.2018.03.066.
- Zhou, N.; Zhao, X.; Xie, Q. Study on Ultrasound-Assisted Extraction of Flavonoids in Cassava Leaves and Its Antioxidant Activity. Cereals Oils. 2016, 10, 21–24.
- Suresh, R.; Saravanakumar, M.; Suganyadevi, P. Anthocyanins from Indian Cassava (Manihot Esculenta Crantz) and Its Antioxidant Properties. Int. J. Pharm. Sci. Res. 2011, 2(7), 1819.
- Liu, P.; Li, -Y.-Y.; Li, H.-M.; Wan, D.-J.; Tang, Y.-J. Determination of the Nucleosides and Nucleobases in Tuber Samples by Dispersive Solid-Phase Extraction Combined with Liquid Chromatography–Mass Spectrometry. Anal. Chim. Acta. 2011, 687(2), 159–167. DOI: 10.1016/j.aca.2010.12.025.
- Di Mauro, A.; Arena, E.; Fallico, B.; Passerini, A.; Maccarone, E. Recovery of Anthocyanins from Pulp Wash of Pigmented Oranges by Concentration on Resins. J. Agric. Food Chem. 2002, 50(21), 5968–5974. DOI: 10.1021/jf025645s.
- Chávez, A. L.; Bedoya, J.; Sánchez, T.; Iglesias, C.; Ceballos, H.; Roca, W. Iron, Carotene, and Ascorbic Acid in Cassava Roots and Leaves. Food Nat. Bull. 2000, 21(4), 410–413. DOI: 10.1177/156482650002100413.
- Achidi, A. U.; Ajayi, O. A.; Maziya‐Dixon, B.; Bokanga, M. The Effect of Processing on the Nutrient Content of Cassava (Manihot Esculenta Crantz) Leaves. J. Food Process. Preserv. 2008, 32(3), 486–502. DOI: 10.1111/j.1745-4549.2007.00165.x.
- Iglesias, C.; Mayer, J.; Chavez, L.; Calle, F. Genetic Potential and Stability of Carotene Content in Cassava Roots. Euphytica. 1997, 94(3), 367–373. DOI: 10.1023/A:1002962108315.
- Yong, L. M.; Liu, C.; Shi, S. Response Surface Methodology for Optimizing Extracion of Flavonoids from Cassava Leaves. Food Res. Dev. 2017, 38(10), 63–67.
- He, C. W.; Tan, J. P.; Huang, Q. N. Content Determination of Rutin in Manihot Esculenta Leaves by HPLC. J. Chin. Pharm.. 2011, 22(23), 2160–2161.
- Kerio, L. C.; Wachira, F. N.; Wanyoko, J. K.; Rotich, M. K. Total Polyphenols, Catechin Profiles and Antioxidant Activity of Tea Products from Purple Leaf Coloured Tea Cultivars. Food Chem. 2013, 136(3–4), 1405–1413. DOI: 10.1016/j.foodchem.2012.09.066.
- Wang, D. M.; Wang, W.; Mei, L. W.; Yang, X.; Li, Q. F. Simultaneous Determination of 6 Flavonoids in Cassava Leaves from Different Harvest Time by HPLC. Food Res. Dev.. 2017, 38(18), 132–210.